Abstract
Coronavirus disease 2019 (COVID-19) is currently a major public health concern causing devastating sociological, economic, and psychological damage to livelihood all over the world. The most intense severity of COVID-19 is not only acute respiratory distress syndrome (ARDS), it also causes multi-organ failure, the post-infection secondary effect as well as death. The fast-mutating ability and high transmissibility rate of the virus cause emergence of the new variants and also the occurrence of breakthrough infections. Evidence suggests that vaccination against COVID-19 has been effective at preventing the severity of illness, hospitalization, and death. The efficacy of vaccines depends on multiple factors including the host’s ability to mount a robust and sustainable immune response, the virus’s ability to mutate its genome, and programmatic factors such as vaccine dose, storage, dosing schedules, etc. In this article, an overview of severe acute respiratory syndrome coronavirus-2 (SARS-CoV-2) infection, its pathogenesis, host immune responses to infection, and different type of COVID-19 vaccines, including vaccine efficacy and adverse effects are described.
Keywords
Coronavirus disease 2019, vaccines, immune response, efficacy, adverse effectIntroduction
The coronavirus disease 2019 (COVID-19) pandemic caused by severe acute respiratory syndrome coronavirus-2 (SARS-CoV-2) has caused millions of deaths worldwide in the last three years since it was first identified in December 2019. So far, as of 28th May 2022, approximately, 527.8 million people were affected with SARS-CoV-2 and approximate deaths of 6.3 million worldwide, in which the greater numbers were reported from the United States of America with approximately 83.7 million affected and approximately 1.0 million deaths. In India, the number of people affected was approximately 43.1 million with approximately 0.52 million deaths (https://news.google.com/covid19/map?hl=en-IN&gl=IN&ceid=IN%3Aen). The estimated economic loss was approximately $8.5 trillion, thus causing huge public health and economic burden worldwide (https://www.un.org/en/desa/covid-19-slash-global-economic-output-85-trillion-over-next-two-years). Obesity and other non-communicable diseases, including type 2 diabetes mellitus, cardiovascular disease, neurodegenerative diseases, cancer, and non-alcoholic fatty liver disease (NAFLD), have emerged as major determinants of severe COVID-19. Further, recent studies have found that other impaired metabolic determinants, specifically visceral obesity, hyperglycemia, hypertension, and subclinical inflammation, increase the risk of severity as well as found to be consequences of severe COVID-19 and might adversely affect the efficacy of COVID-19 vaccines [1].
SARS-CoV-2 is an enveloped, positive sense single-stranded RNA (ssRNA) virus of approximately 30 kb genome size belongs to the Cononaviridae family. The rod-shaped spike (S) protein present on the envelope binds to the angiotensin converting enzyme 2 (ACE2) receptors present on the host cell surface and mediates the viral entry into cells. The S protein is the main protein that determines the diversity of coronaviruses, host tropism responsible for virus antigenic properties [2]. The S protein contains two subunits S1 and S2. The S1 mediates the binding to the ACE2 while S2 catalyzes the fusion of cell membrane and viral membrane ensuring the virus entry through receptor-mediated endocytosis and release of the virion into the cytoplasm of host cells [3]. ACE2 expression is high in the lung, liver, heart, colon, ileum, kidney, and bladder [4]. Studies have also found that the small intestine, testis, thyroid, and adipose tissue have the highest ACE2 expression levels, while blood, spleen, bone marrow, blood vessels, and muscle have the lowest ACE2 expression. This indicates that SARS-CoV-2 can infect other tissues in addition to the lungs, including male tissues such as the testis and seminal vesicle [5]. The expression of ACE2 in various tissues and the lungs suggests that SARS-CoV-2 infection adversely affects other tissues’ functioning. Subjects with cirrhosis have higher hepatic decompensation and liver failure rates following SARS-CoV-2 infection. The possible pathogenesis involved is increased systemic inflammation, cirrhosis-associated immune dysfunction, coagulopathy, and gut dysbiosis, causing respiratory failure and death [6]. These results further indicate that impaired liver function may adversely affect the vaccine efficacy in subjects with liver cirrhosis.
ACE2 receptors are highly expressed on the apical surface of lung epithelial cells thus allowing them as a portal of entry for the virus. Once virion is released into the cell, viral RNA undergoes replication. Viral messenger RNA (mRNA) is used as a template for the synthesis of new viral proteins. Subsequently, new viral particles are packaged in the cell and released out of that host cell thus inducing the host cell lysis. The death of lung epithelial cells matches the fact that early lung injury is often observed in the distal airway [7, 8].
Following the lysis of host cells, the virus gets exposed to the innate immune cells including dendritic cells (DCs), and alveolar macrophages which get activated phagocytize the dying cells and present the viral antigen to the adaptive immune cells such as T lymphocytes which in turn mount the adaptive immune response. The immune response following the viral infection varies according to the immune status, age, disease severity, and presence of underlying comorbidities such as diabetes, cancer, etc., in subjects. Over a period of time, natural infection contributes to the development of herd immunity that subsequently helps in minimizing the spread of infection, hospitalization, and deaths against various viral diseases including SARS-CoV-2 and its variants including the delta found in India [9]. The herd immunity can be achieved either by natural infection or by artificially through vaccination. However, achieving herd immunity by natural infection in COVID-19 has been found to be risky and takes a longer duration [10]. The advancement in biomedical research has paved the way for the development and production of a number of COVID-19 vaccines including nucleic acid-based [Pfizer/BioNTech’s (BNT162b2), Moderna’s (mRNA-1273)] viral vector-based [Oxford-AstraZeneca vaccine and serum institute AZD1222 (ChAdOx1)], subunit [Novovax (NVX-CoV2373)] and inactivated whole virus [Bharat Biotech (Covaxin, BBV152)] vaccines [11].
As vaccines induce the protective immune response by promoting the production of COVID-19 specific virus-neutralizing antibodies and immune memory cells, evidence from case studies and large observational studies suggests that the protective immune response lasts for approximately 6–12 months [12]. Further, emerging data, including evidence from breakthrough infections suggest that the effectiveness of the vaccine might be reduced significantly against emerging variants of concern such as “Omicron” and hence secondary vaccines will need to be developed to maintain herd immunity in addition to developing other antiviral drugs. Further, vaccine efficacy may also get adversely affected due to underlying comorbidities. Studies have found that diabetes, obesity, and other diseases associated with impaired metabolic health affect COVID-19 outcomes independently of each other. One of the studies done on a large community-based cohort (6,910,695 patients) from the UK reveals that high body mass index (BMI, > 23 kg/m2) in the age group of 10–39 years old was associated with adverse COVID-19 outcomes independently of several other COVID-19 risk factors including type 2 diabetes mellitus in patients [13]. Further, hyperglycemia was found to promote viral replication and cytokine production in monocytes, and glycolysis was found to sustain SARS-CoV-2-induced monocyte response and viral replication [14]. Similarly, studies have shown that pre-existing comorbidities such as overweight, type 2 diabetes, and cardiovascular disease were associated with vaccine breakthrough COVID-19 infections [15]. Further, it was found that vaccine efficacy drops down to 86% and 89% in individuals with three or more existing conditions, respectively, compared to 91% with documented infection and 93% with symptomatic illness following seven days post-vaccination with a second dose of the BNT162b2 mRNA vaccine [16]. Studies also indicate that a time-dependent decline in antibody levels might increase the risk of breakthrough infections [17]. These results suggest that comorbidities downregulate the COVID-19 vaccine efficacy, thus contributing to the risk of breakthrough infections. There are reports that the spread of some variants has been attributed to enhanced virus transmissibility and partial immune escape indicating that vaccines may offer reduced protection against some variants. There is little evidence to suggest that alpha evades vaccine-induced immune response and neutralization by post-vaccination sera is similar to wild type, and minimal differences in vaccine effectiveness have been observed [16]. However, studies report conflicting evidence against the beta variant [18]. There is also some evidence of a reduction in vaccine effectiveness against gamma and delta variants [19]. For the recent Omicron variant, reports suggest a greater reduction in vaccine effectiveness in fully vaccinated individuals after receipt of booster vaccine doses. However, the mild to moderate course of illness indicates that full vaccination followed by a booster dose still protects against the severity caused by the Omicron [20]. Further reports also suggest that the risk of hospitalization or death was lower for Omicron cases than delta cases [21].
On the other side, safety concerns have been raised following the administration of COVID-19 vaccines. Some of the common adverse effects include pain at the injection site, and non-specific systemic symptoms, such as fatigue, myalgia, headache, and fever. These symptoms may be observed soon after the vaccination and get resolved in a short period. However, recent reports from case studies have found vaccine-induced side effects in the form of unusual thrombotic events with thrombocytopenia, neurological effects, such as transverse myelitis, Guillain-Barre syndrome (GBS) following administration of COVID-19 viral vector vaccines [22]. This review provides a brief overview of host immune responses to the SARS-CoV-2 infection and vaccination, followed by vaccine efficacy, safety, immunogenicity, and their side effects.
Immune response to COVID-19 infection
COVID-19 infection induces a robust host humoral and cellular immune response. It has been found that COVID-19-specific immunoglobulin A (IgA) and IgG have been detected from both mucosal sites and serum of the COVID-19 infected persons [23, 24]. Also, the presence of IgM, IgA, and IgG was found in the blood sample of the infected person 5–15 days prior to the onset of symptoms [17]. In the very first week of the infected person, the IgM antibodies peak and then fall down until the detectable limit within 2–3 months after the infection [25, 26]. In the meantime, IgA antibodies also decrease gradually and become undetectable within the 3 months after infection while IgG antibodies are more stable. COVID-19-specific memory B cells and T cells will start to emerge within the first month following the infection [27, 28]. The degree of immune response after infection depends on multiple factors. Several studies reported that subjects infected with COVID-19 and who are symptomatic tend to have a higher antibody titer as compared to the asymptomatic person, also the person who is hospitalized tends to have higher antibody titers as compared to the outpatients. In subjects with severe COVID-19 infection, both binding and neutralizing antibody titers rise faster and reach the peak [25, 28, 29].
SARS-CoV-2 enters the host cell through S protein by receptor-mediated endocytosis. The virus replicates and gets packaged and gets released out of the target cell and infects new cells. Following the release out of the cell, the virus comes in contact with the innate immune system [30]. Epithelial cells, DCs, and alveolar macrophages form the main cellular components of the innate immunity in the airway [31]. DCs and macrophages function as innate immune cells to fight against viruses till adaptive immunity is mounted. During the initial phase of infection, DCs and macrophages phagocytize virus-infected epithelial cells and present the viral antigen through class II major histocompatibility complex (MHC) to T helper (Th) cells. SARS-CoV-2 can also infect DCs through DC-specific intercellular adhesion molecule-3 grabbing non-integrin (DC-SIGN) and furin and DC-SIGN-related (DC-SIGNR) protein in addition to the ACE2 and transmembrane protease serine 2 (TMPRSS2) [32]. Th cells in turn activate B-cells and induce their differentiation into plasma cells that produce antibodies thus mounting the humoral response, while cytotoxic T cells can kill the virus-infected cells thus activating the adaptive response to SARS-CoV-2 infection. Further, the innate immune response also gets activated through pattern recognition receptors (PRRs), such as C-type lectin-like receptors, NOD-like receptor(NLR), Toll-like receptor (TLR), and RIG-I-like receptor (RLR). The COVID-19 virus induces the expression of numerous inflammatory factors, and the synthesis of type I interferons [IFNs; IFN-γ, IFN-α, interleukin-1β (IL-1β), IL-6, IL-12, IL-18, IL-33, tumor necrosis factor-α (TNF-α), transforming growth factor beta (TGF-β), C-C motif chemokine ligand 2 (CCL2), CCL3, CCL5, C-X-C motif ligand 8 (CXCL8), CXCL9, CXCL10, receiver operating characteristic (ROC)], which limit the spread of viral and accelerate macrophage phagocytosis of the viral antigens resulting in the clinical recovery [33–37]. However, the nucleocapsid (N) protein of COVID-19 helps the virus to escape from the immune responses. A schematic representation of the immune response against COVID-19 is presented in Figure 1.
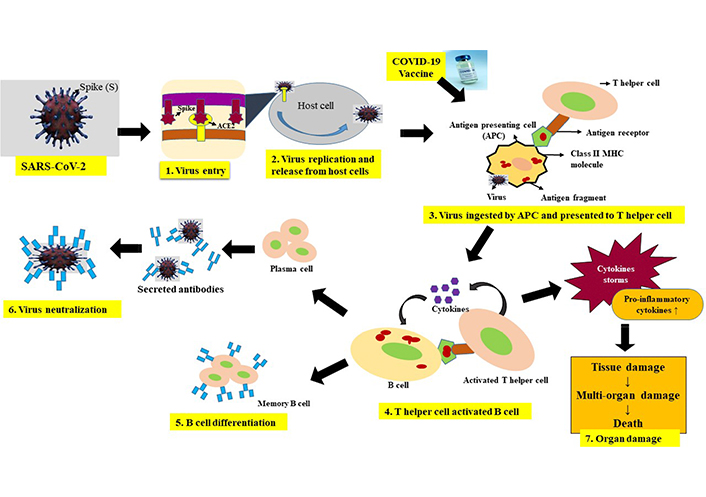
The picture depicts the overview of host immune response to COVID-19 infection or COVID-19 vaccine. SARS-CoV-2 enters the host cell through its S protein by receptor mediated endocytosis. Inside the host cell, virus replicates and new viral particles are released causing target cell lysis. Antigen presenting cells recognize the virus infected cells and phagocytize them. Process the viral antigen and present to the Th cells via class II MHC. In the case of vaccine, following the vaccine injection, antigen presenting cells, process the S protein (in case of viral vector vaccines and nucleic acid vaccines) or process the antigen from the inactivated whole virus vaccine, and present to the Th cells as above. Th cells recognize the viral antigen and activated and secrete cytokines which in turn activate B cells. Activated B cells differentiate into plasma cells and memory B cells. Plasma cells secrete antibodies which neutralize the viral antigen. As disease progress pathogenic CD4+ secrete higher amounts of proinflammatory cytokines such as IL-6, monocyte chemoattractant protein 1 (MCP-1), TNF-α, granulocyte-macrophage colony-stimulating factor (GM-CSF), etc., which in turn cause infiltration of neutrophils and tissue macrophages, lymphocytes leading to tissue and organ failure. Memory cells formed due to vaccine or infection, following the repeat encounter of the same antigen or virus gets activated and differentiated into antibody producing plasma cells. CD4: cell differentiation 4; ↓: decrease; ↑: increase
As the disease progresses in some patients, there is a reduction in peripheral blood T cells (lymphopenia) as determined by the elevated expression levels of checkpoint receptor transmembrane domain 3 (Tm3)+ programmed cell death protein 1 (PD-1)+ in the cluster of CD4+ and CD8+ T cells and elevation of NK group 2 member A (NKG2A) in CD8+ T cells [38]. Further, it was observed that activated CD4+ cells express higher levels of proinflammatory cytokines including IL-6, IL-10, granulocyte-colony stimulating factor (G-CSF), MCP-1, macrophage inflammatory protein 1α (MIP1α), and TNF-α [39]. The severity is correlated with the levels of IL-6. The elevated expression levels of CD69, CD38, and CD44 indicate the hyperactivation of CD4+ and CD8+ cells. The aberrant pathogenic CD4+ cells co-express IFN-γ and GM-CSF [40]. GM-CSF though helps in the differentiation of innate immune cells and activates T cells, but it can initiate tissue damage in excess [41]. GM-CSF+IFN-γ+CD4+ cells were found at substantially higher levels in autoimmune encephalomyelitis (EAE) models that secreted higher amounts of IL-6. Additionally, SARS-CoV-2 infected cells also secrete higher amounts of IL-8, which is a chemoattractant for neutrophils and T cells. Infiltration of a large number of inflammatory cells was observed in severe COVID-19 patients [42, 43], and these cells presumably consist of a group of neutrophils and T cells. Neutrophils in large numbers induce lung injury [44–46]. Considering the reduction in circulating T cells, infiltrating T cells, similar to neutrophils, can kill the virus and also cause lung injury when present in excess at the tissue level [47]. Additionally, a large number of monocytes (CD14+CD16+) were also found to infiltrate into the lung tissue and these cells secrete higher levels of IL-6, which is likely to accelerate the progression of systemic inflammatory response. Together, aberrant expression of chemoattractant and proinflammatory factors by CD+ cells cause huge infiltration of both nonspecific and adaptive immune cells causing tissue damage and failure.
Immune response to COVID-19 vaccine
Vaccination is the best way to protect oneself against the COVID-19 infection, as it may train one’s immune system to respond quickly and effectively to the virus infection, thereby preventing severity of the illness. The vaccine works with the immune system and builds immune memory T and B-lymphocytes for the COVID-19 and can protect for a long time after receiving both doses of the vaccine.
Different types of vaccines work in different ways to offer protection. Following the vaccination, the Th cells will detect the S protein and activate other immune cells followed by the killer T cells that seek out the S protein. B cells will recognize the S protein and will create an antibody against the virus. Vaccination will induce the production of anti-S and anti-receptor-binding domain (RBD) binding and neutralizing antibodies in the blood and similar to an infection, vaccine results in early production of the serum IgA, IgM, and IgG antibodies, and also induce long-lasting memory B-cell and T-cell responses [48–50]. Second dose vaccine which is mostly administered 4–8 weeks later will train the immune system further and strengthen the response and will create an immune memory for the virus. The immune system will be ready to fight quickly and effectively against COVID-19 in the future, thereby preventing the infection and controlling the spread of the pandemic [51].
The various study supports the vaccination concept which has the potential to prevent COVID-19 infection. In one of the studies, it was found that vaccination of primates protects against viral challenges, and the development of functional neutralizing antibodies, thereby protecting against severe illness. Similarly, epidemiologic studies in humans have also suggested that neutralizing antibodies is associated with protection from infection [13, 52, 53].
Vaccines against COVID-19 that evoke protective immune responses are crucial to the prevention and mitigation of the morbidity and mortality caused by SARS-CoV-2 infection. Many reports suggest that balancing humoral and Th1-directed cellular immune response might be an important aspect of protecting against COVID-19 and also avoiding the vaccine-enhanced diseases [54, 55]. Considering this, several vaccines have been developed and tested which include nucleic acid vaccines, inactivated virus vaccines, protein/peptide subunit vaccines, live attenuated vaccines, and viral vectored vaccines [56, 57]. Each vaccine has its own advantages and disadvantages. By the end of 2020, many vaccines had been developed and made available for use in different parts of the world. Out of these vaccines, the World Health Organization (WHO) has approved 7 vaccines to fight against COVID-19.
COVID vaccines, efficacy, and reported adverse effects
Moderna: mRNA-1273
After the analysis of SARS-CoV-2 virus genome, the mRNA-1273 vaccine was developed by Moderna to prevent and protect persons against the COVID-19. The mRNA-1273 vaccine is a lipid nanoparticle-encapsulated mRNA-based vaccine that encodes the S protein stabilized in the prefusion conformation, thereby improving expression and increasing its immunogenicity.
Efficacy: Data reported by Corbett et al. [58] shows that a low dose of mRNA-1273 vaccine induces potent neutralizing antibody responses, thereby protecting against SARS-CoV-2 infection in the mice without the evidence of immunopathology. This data has provided the possibility that the vaccine developed with mRNA-1273 could help to fight against COVID-19 in nonhuman primates and further investigation has been carried out by Corbett et al. [59]. In this study, the nonhuman primates vaccinated with mRNA-1273 induced the neutralizing activity against SARS-CoV-2 thereby protecting the upper and lower airways with no pathogenesis. During the phase 1 trial, 34 healthy adults in the age group 18–55 years have been administered with 100 μg dose of mRNA-1273 vaccine and found that it produced high levels of binding and neutralizing antibodies that slightly decline over time but remain elevated for 3 months after the booster vaccination [60]. During the phase 2 trial, vaccination with mRNA-1273 shows high immune responses against SARS-CoV-2 in all the participants of age group 18–55 years with an acceptable safety profile which confirmed the safety and immunogenicity of 50–100 μg of dose which should be given as 2-dose regimen [61]. During the phase 3 study, a randomized, double-blind trial was carried out with 30,420 participants ≥ 18 years old, which were administered with either vaccine or placebo in two intramuscular injections 28 days apart. Results obtained show that two doses of the mRNA-1273 vaccine were safe and provided 94.4% efficacy against symptomatic COVID-19 in persons aged 18 or older [62].
Immune response and booster dose: The Moderna vaccine has shown higher effectiveness against COVID-19 soon after the second dose is administered, as binding and neutralizing antibody responses are similar to those seen in convalescent plasma with vaccination in healthy individuals of 18–55 years of age. Moderna vaccine is also very effective in adults older than 55 years and shows a similar immune response when compared with the younger population. CD4 cell responses with a Th1 bias were also detected [63]. Some of the findings reported that protective immunity remains high with a little decrease in antibodies after six months of the administration. One of the studies reported the level of antibodies CD4+ T cells, and CD8+ T cells remained high after six months of the administration. Also, it was found that the vaccine generates a similar immune response against the COVID-19 S protein to that of the natural COVID-19 infection. The levels of antibodies, CD4+ T cells and CD8+ T cells after six months were comparable with the recovered individuals [64]. The study also reported the need for a booster dose, which will improve immune memory. The booster dose will rapidly restore the memory B cells and T cells which is important for long-term protection against COVID-19 [65].
Side effect: As all vaccines have advantages as well as disadvantages, the Moderna vaccine has also been reported to have some adverse reactions during clinical trials after administration of the vaccine which include pain, swelling, erythema at the injection site, headache, fatigue, chills, arthralgia, myalgia, nausea/vomiting, axillary swelling, fever. During the mass vaccination, some other severe allergic reaction has been reported including anaphylaxis, myocarditis, pericarditis, and syncope [66–71].
Pfizer: BNT162b2
Pfizer and BioNTech have developed a series of mRNA-based COVID-19 vaccines, out of the series tested, the BNT162b1 vaccine which is a lipid nanoparticle-formulated, nucleoside-modified mRNA vaccine, elicited RBD-binding IgG and neutralizing antibodies with mild side effects. BNT162b2 has been authorized for worldwide use.
Efficacy: During the random trial among the children and adults, the Pfizer vaccine was highly effective during the first several months after the vaccine is administrated, and in the phase III trial, a large placebo-controlled among 36,000 participants aged 16 years and older the vaccine shows 95% efficacy after 7th day of the second dose administered [72]. The study reported that the Pfizer vaccine was 91.7 % effective among adults ≥ 65 years who had medical comorbidities or obesity. The efficacy of the vaccine among adolescents aged 12–15 years was 95% and among children aged 5–11 years was 91% with lower doses. Till date, no severe cases were reported in the trials of the vaccine for children and adolescents [73, 74].
Immune response and booster dose: In phase I/II trial, the randomized, placebo-controlled, observer-blind dose-escalation study carried out in healthy adults between 18–85 years of age, the results obtained show the binding and neutralizing antibody responses which were very similar to those seen in convalescent plasma from the patients who had asymptomatic or moderate COVID-19 infection [75]. The immune response in the participants ≥ 65 years old was found lower as compared to the younger participants. The vaccine is more effective and appears more immunogenic among children and adolescents as compared to young adults. Studies have found that the neutralizing antibody titers induced in participants aged 12–15 years are significantly higher as compared to the aged group 16–25 years [73]. In one of the studies, it was found that the neutralizing antibody titers declined with time following the administration of the BNT162b2 vaccine [76]. Booster doses boost the immune response for longer protection. In a study carried out in Israel, which involve the participant 60 years of age or older and who had already received two doses of the BNT162b2 vaccine at least 5 months earlier, it was found that the rate of positive COVID-19 cases and severe illness were very low among the participant who received a booster dose of the BNT162b2 vaccine [77].
Side effect: The most common side effects after the second dose are of very mild and moderate severity and stay almost 2–3 days after vaccination [78]. Some of the side effects are an injection site reaction (redness, pain, swelling, and pruritus), fatigue, headache, myalgias which are seen after each dose, and fever, chills, and joint pain which are seen mostly after the second dose. Fatigue, headache, chills, and myalgia are commonly seen among adolescents aged 12–15 years, and mild symptoms among children 5–11 years were reported [73, 74].
Janssen (Johnson& Johnson): Ad26.COV2. S
Janssen vaccine has been approved by WHO for emergency use on 12th March 2021. The Janssen vaccine is a non-replicating adenoviral vector vaccine that contains vector viruses and helps in delivering instruction to the host cells to produce an antigen called S protein against COVID-19, thereby triggering the production of antibodies, helping to generate an immune response and to retain the information in memory immune cells. It is given intramuscularly as a single dose but is also being evaluated as two doses 56 days apart.
Efficacy: During the Clinical trials in participants who were administered with a single dose of vaccine, a total of 468 confirmed cases were found after the 14 days of vaccine administration, whereas 464 participants faced mild to moderate severity, showing 66.9% efficacy against COVID-19 infection. Following this, after the 28th day of vaccine administration, 66 more cases were found facing severe-critical cases showing 93.1% efficacy. Also, it was found that there were fewer severe-critical cases among the older patients than the younger patients, thereby indicating early protection against the virus [79–81].
Immune response and booster dose: During the phase I and II trials with double-blind and placebo-controlled subjects, higher rates of neutralizing and binding antibodies were found in healthy individuals among 18–85 years old after the administration of the first dose [79]. CD4 cell responses with a Th1 bias were also detected after the first dose. After the second dose, there was an increase in neutralizing titers among the participants [82]. It was found that the Janssen vaccines are largely stable over eight months with both one or two doses [49].
Side effects: Some of the common side effects after the first day of vaccination are of mild or moderate severity by Shay et al [83]. Post-vaccination surveys among over 330,000 vaccine recipients revealed that 76% faced at least one systemic reaction and 61% reported one injection site reaction in the first week. Most of the common systemic reactions reported after the administration of the vaccine were pain, headache, fatigue, and anxiety-related events which included tachycardia, hyperventilation, light-headedness, and syncope [84]. Also, some severe cases were reported, like thromboembolic events, tinnitus, and seizures among the vaccine recipients [85].
Oxford/AstraZeneca: AZD1222
AZD1222 vaccine is developed based on a chimpanzee adenovirus vector that encodes the S glycoprotein of COVID-19. The AZD1222 vaccine (ChAdOx1/AZD1222) has been developed by the collaboration of both Oxford/AstraZeneca. It is given intramuscularly in two doses 4–12 weeks apart.
Efficacy: The efficacy of the AZD1222 vaccine varies as per the interval of the dose administration. At first, the vaccine doses were planned as single-dose vaccines, later it was found that administration of second doses significantly increases the neutralizing antibodies. In an interim study which includes data from four ongoing blinded, randomized and controlled trials across UK, Brazil, and South Africa, where the participants were 18 years old and older; it was found that the efficacy of the two doses of the vaccine was 70.4% and the protection of 64.1% after the one single dose against the COVID-19 virus. Based on these results, the vaccine was authorized for emergency use with a regimen of two standard doses 4–12 weeks apart for adults aged 18 years old and older.
Immune response and booster dose: During the phase I/II clinical trial among 18–55 years age group in a single-blind, randomized controlled subject, it was found that neutralizing antibody titers after 28 days of the last dose administered which was similar to those detected in convalescent plasma treatment [86]. It was reported that after the second dose, the antibody titers were high, and after the third dose, it is even higher [87]. The cellular response was also detected. Also, it was reported that the immune response of the older recipients > 70 years is similar compared to the younger adults after the second dose [88]. A third dose (booster dose) of the AZD1222 vaccine induces the antibodies to a level that correlates with high efficacy and boosts T cell response [87, 89].
Side effect: During the earlier phase of clinical trials, some of the common side effects seen were pain at the injection site, headache, fatigue, and fever in almost 8% of the recipients [86]. And during the phase III clinical trial, among all the vaccine recipients, two cases were found to have transverse myelitis and sclerosis, which were not clear whether it is related to the vaccine [90].
Sinopharm: BBIBP-CorV
BBIBP-CorV has been developed by Sinopharm, is a two-inactivated whole-virus, alum-adjuvanted vaccine, and they are given intramuscularly in two doses 28 days apart [48].
Efficacy: In a phase III efficacy clinical trial (December 2020), UAE’s Ministry of Health and Prevention announced the interim analysis of the Sinopharm vaccine has 86% efficacy against COVID-19. Later on, on 7th May 2021, the WHO reported the efficacy of the vaccine to be 79% against the COVID-10 virus symptomatic diseases and 79% against hospitalized patients [91].
Immune response and booster dose: Sinopharm vaccines appeared to induce high seroconversion rates and induce a similar level of antibody responses against the virus. Sinopharm vaccine-elicited neutralizing and binding antibody responses in healthy individuals of 18–80 years old in several phase trials with no severe reaction [92]. The administration of the third dose of Sinopharm significantly increased the S protein antibody levels among the participants [93].
Side effect: After the first vaccination, the most common side effect among the participants aged ≤ 49 and > 49 are normal injection site pain, fatigue, and headache. And after the second dose most common side effects reported were pain at the injection site, headache, lethargy, and tenderness. All the side effects were even more common among the participants aged ≤ 49 and in females as compared to males [93].
Sinovac (CoronaVac)
The Sinovac vaccine has been developed in China, and is an aluminum-hydroxide-adjuvanted, inactivated whole virus vaccine. It is given intramuscularly in two doses 28 days apart.
Efficacy: In a phase 3 clinical trial in Brazil, after the administration of the second dose at an interval of 14 days, it was found that the efficacy of the vaccine was 51% among the symptomatic COVID-19 infection, 100% among the severe COVID-19 and 100% against the hospitalization. No severe cases were reported. The efficacy of the vaccine was maintained in the groups with or without comorbidities. The median duration of the follow-up was 73 days. In phase III trials, an interim analysis in Indonesia, the efficacy of the vaccine was 65.3% and in turkey, it was 83.5% against the COVID-19 infection [94].
Immune response and booster dose: In phase I/II clinical trial with randomized, placebo-controlled, double-blind subjects among healthy individuals aged 18–59 years, it was found that the vaccine was well-tolerated and induced humoral responses against the COVID-19 infection [95]. Also, the study conducted among children and adolescents aged 3–17 years shows higher neutralizing antibody titers [96]. The study conducted among adults aged 60 years and older also show tolerability and high neutralizing antibody titers [97]. The administration of the third dose of Sinovac after 6 or more months following the second dose significantly increases the antibody levels and generates good immune memory [98].
Side effects: Some of the local side effects are most commonly seen, which were pain at the injection site, redness, increased temperature and systemic side effects are very less [99].
Covaxin: BBV152
Covaxin (BBV152) vaccine was developed and is being used in India; is a whole virion inactivated COVID-19 antigen that is adsorbed to the alum and formulated with a TLR7/8 agonist imidazo quinolin gallamide (IMDG) and the preservative 2-phenoxyethanol [100]. The vaccine is given intramuscularly in two doses 29 days apart.
Efficacy: In phase III trials, the participants recruited were aged ≥ 18 years, an interim analysis was conducted and the efficacy of the vaccine was found 78% after 14 days of second dose administration. In the adults < 60 years, the efficacy of the vaccine was 79% and in participants aged ≥ 60 years was 68%. The efficacy of the vaccine against the asymptomatic COVID-19 infection was 64%. Overall, the BBV152 vaccine demonstrated acceptable safety and reactogenicity profile in adults aged ≥ 18 years [101].
Immune response and booster dose: The BBV152 vaccine appeared to be safe and immunogenic in healthy individuals aged 18–55 years. It has been found that the serum from the vaccine recipients has the tendency to neutralize the delta variants in India. Also, the IMDG and alum are adjuvants that are added to enhance immunogenicity, also study reported that TLR7/8 agonists enhance Th1 responses and inhibit Th2 responses which will help to fight against the COVID-19 virus. TLR7/8 agonists also help to increase the CD8 T-cell responses [100, 101].
Side effect: The most common side effect of the BBV152 vaccine were muscle pain, fever, pain at the injection site, headache, and fatigue. One case was reported as adverse events which were not deemed related to immune thrombocytopenic purpura [101]. The list of vaccines, their efficacy, and reported adverse effects are provided in Table 1.
List of vaccines and their efficacy and reported side effects
COVID vaccines | Efficacy | Adverse effect | References |
---|---|---|---|
Moderna: mRNA-1273 | 94.4% | Pain, swelling and erythema at the injection site, headache, fatigue, chills, arthralgia, myalgia, nausea/vomiting, axillary swelling, fever, and an allergic reaction which includes anaphylaxis, myocarditis, pericarditis, and syncope | [66–71] |
Pfizer: BNT162b1 | 91.7% | Injection site reaction (redness, pain, swelling, and pruritus), fatigue, headache, myalgias fever, chills, and joint pain | [73, 74] |
Janssen (Johnson & Johnson): Ad26.COV2. S | 93.1% | Pain, headache, fatigue, and anxiety-related events which included tachycardia, hyperventilation, light-headedness, and syncope | [84, 85] |
Oxford/AstraZeneca: AZD1222 | 70.4% | Pain at the injection site, headache, fatigue, fever, transverse myelitis, and sclerosis | [86, 90] |
Sinopharm: BBIBP-CorV | 79% | Normal injection site pain, fatigue, headache, lethargy, and tenderness | [93] |
Sinovac (CoronaVac) | 83.5% | Pain at the injection site, redness, increased temperature, and systemic side effects are very less | [99] |
Covaxin: BBV152 | 78% | Muscle pain, fever, pain at the injection site, headache, and fatigue | [101] |
Conclusions
The COVID-19 pandemic has caused significant devastating effects on public health and a huge economic burden worldwide. As new drugs and other treatment modalities and vaccines are being developed, the virus uses an alternative strategy in the form of mutants to evade the effect of drugs and vaccines to survive and propagate in susceptible hosts. Despite adverse effects particularly for viral vector vaccines have been reported, at this hour of public health crisis worldwide, vaccines still form the potent weapons that induce a protective immune response to keep the transmission of the SARS-CoV-2 virus under control, minimize the hospitalization and case fatality rate. Further, a thorough understanding of the interaction between host and viral proteins, viral evasion of the immune response, viral mutations, etc., will help in designing novel antiviral targets to counter viral pathogenesis. Additionally, based on age, type of vaccine, presence of comorbidities, individual variation in response to the vaccine, dwindling antibody levels over a period of time, evolving of viral mutants, new vaccines, and new strategies such as booster dosing or annual vaccination have to be debated and adapted based on scientific studies.
Abbreviations
ACE2: | angiotensin converting enzyme 2 |
CCL2: | C-C motif chemokine ligand 2 |
CD4: | cell differentiation 4 |
COVID-19: | coronavirus disease 2019 |
CXCL8: | C-X-C motif ligand 8 |
DCs: | dendritic cells |
IgA: | immunoglobulin A |
IL-1β: | interleukin-1β |
MHC: | major histocompatibility complex |
mRNA: | messenger RNA |
S: | spike |
SARS-CoV-2: | severe acute respiratory syndrome coronavirus-2 |
Th: | T helper |
TLR: | Toll-like receptor |
TNF-α: | tumor necrosis factor-α |
WHO: | World Health Organization |
Declarations
Acknowledgments
We thank the Director, CSIR-CFTRI for providing facilities to carry out this work. The corresponding author would like to thank the Department of Biotechnology (DBT), Govt. of India for providing the Ramalingaswami Fellowship during the study.
Author contributions
RPV: conceptualization and supervision and final draft preparation. BRS: data curation, original draft preparation. Both of the authors contributed to manuscript revision, read and approved the submitted version.
Conflicts of interest
The authors declare that they have no conflicts of interest.
Ethical approval
Not applicable.
Consent to participate
Not applicable.
Consent to publication
Not applicable.
Availability of data and materials
Not applicable.
Funding
Not applicable.
Copyright
© The Author(s) 2022.