Abstract
Sepsis was defined in 1991 by the systemic inflammatory response syndrome (SIRS) criteria which consisted mostly of physiologic responses to infection or inflammation (fever, tachycardia, tachypnea, and leukocytosis). These criteria were initially proposed to identify patients with gram-negative bloodstream infection (BSI). However, most patients with BSI are not critically ill at initial presentation using objective clinical scores for acute severity of illness, such as the Pitt bacteremia score (PBS). Lack of specificity and low positive predictive value (PPV) are other pitfalls of the SIRS criteria. Moreover, the implementation of sepsis interventions based on this outdated definition failed to improve patients’ outcomes and in some settings was associated with increased use of broad-spectrum antibiotics and Clostridioides difficile (C. difficile) infection. In 2016, sepsis was redefined as a dysregulatory host response to life-threatening infections using quick sequential organ failure assessment (qSOFA) score. The presence of two of three bedside clinical variables (hypotension, respiratory distress, and altered mental status) that have consistently predicted mortality in patients with infections now constitutes sepsis. The scientific debate continues in the medical literature regarding the performance of the new criteria. Some medical professionals and quality organizations consider these changes to the sepsis definition too revolutionary and are resistant to altering existing medical practice. This narrative review presents infection as a continuum from localized to systemic infection (pre-sepsis) with the potential progression into sepsis and septic shock if appropriate antibiotic therapy and source control are delayed. The review assesses host and microbial factors that may influence the rate of progression through the sepsis cascade and proposes diagnostic considerations and management decisions at each step of the way. It emphasizes the need to utilize precision medicine concepts in selecting empirical antibiotic therapy based on patient-specific risk factors for infections due to resistant bacteria and potential benefits from appropriate therapy across the sepsis spectrum.
Keywords
Bacteremia, bloodstream infection, survival, precision medicine, antimicrobialsIntroduction
Sepsis is a leading cause of mortality worldwide and its impact on morbidity for survivors is becoming more recognized [1, 2]. Early detection of sepsis allows timely control of the source of infection and appropriate antimicrobial therapy [3]. The unified effort to improve recognition and rapid intervention of sepsis has led to the implementation of systemic protocols and regulations governing response [4]. However, though commonly encountered, sepsis remains difficult to diagnose and appropriately treat, especially within the narrow windows set by current guidelines [3, 4]. While it could be argued that all patients presenting with sepsis, and particularly septic shock, are critically ill and require quick intervention, the underlying cause of true sepsis, a life-threatening infectious process, typically bacterial, may not be present in many cases. Diagnostic tools used to identify sepsis have overall low specificity as many patients identified with these tools do not have a documented infection. Moreover, many documented infections are not necessarily bacterial or fungal, nor require immediate antibiotic therapy, but rather represent common viral infections [5]. In an era of increasing antibiotic use and resistance, the practice of non-discriminatory use of broad-spectrum antibiotics in patients without life-threatening bacterial infections may have more risks than benefits [6]. The implementation of broad sepsis guidelines has resulted in overuse of broad-spectrum antibiotics and an increase in hospital-onset Clostridioides difficile (C. difficile) infection [7]. With all those limitations of the old sepsis definition, there is room for adjustment and improvement. Moreover, the rise of rapid diagnostic testing for microbial identification and antimicrobial resistance opens the horizons for modern treatment guidelines governing diagnostic work-up and management of sepsis. This allows for appropriately expedient care of patients with life-threatening infections while reducing unintended consequences of indiscriminate broad-spectrum antibiotic therapy.
The evolution of sepsis definition
Sepsis as a systemic infection or inflammation (1991)
Modern understanding of sepsis and its treatment have transitioned through several iterations. Bone et al. [8], commonly referred to as Sepsis-1, a consensus letter from three prominent physicians representing the American College of Chest Physicians (ACCP) and the Society of Critical Care Medicine (SCCM), sought mainly to define sepsis and focused on gram-negative bloodstream infection (BSI). In this manuscript, the term systemic inflammatory response syndrome (SIRS) was adopted. Sepsis was defined as a subset of SIRS with documented infection. Multiple organ dysfunction syndrome (MODS) was also coined at this time. Sepsis was diagnosed in patients who met two or more of the following SIRS criteria: body temperature > 38°C or < 36°C, heart rate > 90 beats per min, respiratory rate (RR) > 20 breaths per min or PaCO2 < 32 mmHg, and white blood cell (WBC) count > 12,000 cells/μL or < 4,000 cells/μL with > 10% immature neutrophils (Table 1).
Comparison between contemporary criteria for detection of systemic and life-threatening infections
Characteristic/score | SIRS | qSOFA | qPitt |
---|---|---|---|
Purpose: what is it designed to detect? | Systemic infections (e.g., BSI) | Life-threatening infections (i.e., sepsis) | Critically ill among those with systemic infections or BSI |
Components | Temperature > 38°C or < 36°C | SBP ≤ 100 mmHg | Temperature < 36°C |
Heart rate > 90/min | RR ≥ 22/min | SBP < 90 mmHg or vasopressor use | |
RR > 20/min | Altered mental status | RR ≥ 25/min or mechanical ventilation | |
WBC count > 12,000/μL or < 4,000/μL | Altered mental status | ||
Cardiac arrest | |||
Cut off score to meet criteria | SIRS ≥ 2 | qSOFA ≥ 2 | qPitt ≥ 2 |
Pros | High sensitivity for BSI | Simplest score | High discrimination to predict mortality |
Includes only bedside clinical variables | Includes only bedside clinical variables | ||
Cons | Low specificity for infections | Variable sensitivity and specificity based on population | Not evaluated yet in patients with suspected infections |
Low PPV | |||
Non-discriminatory performance for mortality | |||
Includes a laboratory variable |
qSOFA: quick sequential organ failure assessment; qPitt: quick Pitt bacteremia score; SBP: systolic blood pressure; RR: respiratory rate; PPV: positive predictive value
Levy et al. [9], commonly referred to as Sepsis-2 (2003), promulgated the expert consensus findings of the International Sepsis Definitions Conference. The authors’ goal had been to identify biomarkers to expedite the diagnosis of sepsis, an identified limitation from Sepsis-1. The definition of sepsis remained unchanged but was critiqued for not allowing staging of the patient’s course. The largest addition in Sepsis-2 was an expanded list of laboratory and physical exam findings to improve diagnosis. Sepsis-2 further emphasized the importance of clinical evaluation in the diagnosis of sepsis [9]. A rudimentary staging system was also proposed, but this never gained widespread use.
It is notable that SIRS criteria are a mixture of physiologic and dysregulatory phenomena. Most SIRS criteria represent physiologic responses to infection or inflammation (fever, tachycardia, leukocytosis). Mild tachypnea (RR of 20–22 breaths per min) may represent a physiologic response to systemic infection or inflammation. However, a much faster RR may be a sign of respiratory distress and hypoxia. On the other hand, hypothermia and leukopenia represent dysregulated host response to infection and imply worse prognosis [10].
Limitations of the SIRS criteria
Although the SIRS criteria have high sensitivity for identification of patients with BSI, they lack specificity [11]. This is not surprising since SIRS criteria were developed to identify patients at high risk of BSI or what was previously referred to as “septicemia” [8]. It is estimated that only 50% of patients who meet the SIRS criteria (have ≥ 2 of the SIRS criteria) have documented infections. The remaining 50% have other inflammatory conditions (acute pancreatitis, burns, etc.), major trauma, or surgical procedures. It is essential to emphasize here that meeting SIRS criteria is not synonymous with systemic bacterial infection. In fact, the majority of patients with SIRS do not require systemic broad-spectrum antibacterial therapy. Patients who have SIRS should be evaluated for suspected infections with full history, physical examination, and two sets of blood cultures to ascertain the risk and identify potential source of infection. Additional laboratory and imaging work-up may be obtained as directed by symptoms and signs. Once the source of infection is established, clinical samples from that site should be obtained for traditional cultures or rapid diagnostic methods of microbial identification. Moreover, many patients with SIRS and documented infections have either viral infections that do not require antibacterial therapy or uncomplicated bacterial infections such as cellulitis or otitis media that may be simply treated with narrow-spectrum oral antibiotics in ambulatory settings [5, 11]. Once systemic infections are ruled out after appropriate work-up, antibiotics should not be started or should be discontinued if started empirically. The SIRS criteria have low discrimination to predict mortality among patients with systemic infection such as gram-negative BSI [10].
The other pitfall of SIRS criteria is the low PPV. In some settings, the rate of positive blood cultures due to skin contamination (one of two blood cultures positive for coagulase-negative Staphylococcus or Corynebacterium species) may exceed that of true BSI among patients with SIRS. In one recent study, only 3% of patients with SIRS who had traditional blood cultures obtained had BSI [12]. This proportion increased to 13% by using rapid diagnostic techniques with higher sensitivity of detecting bacteria in the bloodstream such as magnetic resonance techniques [12].
Redefining sepsis as a life-threatening infection (2016)
In 2016, the SCCM and the European Society of Intensive Care Medicine worked together to revise the sepsis definition [13]. They applied existing and new potential definitions to a large multicenter cohort [14]. SIRS criteria were felt to perform poorly by expert opinion and had lower discrimination in predicting in-hospital mortality compared to sequential organ failure assessment (SOFA) and qSOFA scores. In particular, the reviewers felt SIRS criteria insufficiently captured multi-organ failure.
A major advantage of the new definition is the ability to diagnose sepsis at the bedside. All three components in qSOFA (hypotension, respiratory distress, and altered mental status) are clinical variables that can be measured by any healthcare professional regardless of experience or training. This means sepsis can be detected at home, en route to the hospital, in the emergency department, or in ambulatory settings. Clinical bedside skills and a sphygmomanometer are the only tools needed to detect sepsis. This is crucial from multiple points of view. First, early detection of sepsis without the need for a blood draw or laboratory capabilities. Second, the ability to diagnose sepsis outside the hospital. Third, the achievement of health equity across the globe. Sepsis can be diagnosed with the same accuracy in high- and low-income countries, in urban and rural settings regardless of medical resources or accessibility to a laboratory. The definition of respiratory failure as a RR ≥ 22 breaths per min was necessary to allow bedside diagnosis of sepsis despite the potential compromise in specificity. Defining respiratory failure based on oxygen saturation would have required special medical equipment (pulse oximetry) that may not be available worldwide. Similarly, defining respiratory failure based on arterial oxygen pressure would have required arterial puncture, access to an arterial blood gas analyzer, and delayed diagnosis waiting for laboratory results.
In contrast, the previous definition of sepsis based on SIRS criteria included one laboratory variable (leukocytosis, leukopenia, or bandemia) out of four. This implies that detection of sepsis may be delayed until venous puncture and WBC count results are available in patients who only meet one of the other three clinical criteria.
Sepsis-3 allows diagnosis of sepsis by a mixture of clinical and laboratory variables using the full SOFA score, particularly in intensive care unit (ICU)-onset sepsis. Calculation of SOFA score requires multiple laboratory variables (platelets, creatinine, bilirubin, and arterial oxygen pressure). However, many of these variables are routinely obtained in patients admitted to ICUs. Utilization of SOFA score for community-onset sepsis is not practical due to the significant delays obtaining venous and arterial blood and waiting for laboratory results. The rapid detection of sepsis using qSOFA leads to faster clinical decision-making regarding transfer to a medical facility, disposition within the hospital (regular floor, intermediate care unit, or ICU), further diagnostic work-up, appropriate antibiotic and fluid management, and potentially surgical consultation for source control.
Sepsis-3, sought to focus entirely on dysregulatory phenomena and to identify truly life-threatening infections, emphasizing prognostication and prediction of mortality. SIRS was initially replaced with SOFA and qSOFA, in part, due to SIRS’s lack of specificity, although it was rather sensitive. However, SOFA/qSOFA proved to be problematic to implement and many institutions continue to use SIRS in clinical practice. Despite recurrent expert collaboration and advancing diagnostic techniques, sepsis remains a largely clinical diagnosis [15].
Limitations of the SOFA/qSOFA scores
Sepsis-3’s choice of criteria to identify life-threatening infection must also be examined. Namely, body temperature as a criterion was removed entirely from the 2016 definition. Aberration in body temperature, particularly hypothermia has been associated with high mortality, while mounting fever was a good prognostic sign [10, 16]. Utilizing body temperature, particularly hypothermia, actually would fulfill Sepsis-3 goals well, identifying patients at risk for further decompensation. Implementing the qSOFA score retained, but altered, one SIRS criterion. Raising the RR from > 20 to ≥ 22 is a small but important step in the right direction since mild tachypnea represents a physiologic response to infection, while other studies demonstrated that a RR ≥ 25 better defined respiratory distress as a sign of dysregulation and was independently associated with high mortality in patients with BSI [10, 17].
The qSOFA was likely favorable overall to SIRS due to simplicity. However, improved specificity for infection compared to SIRS is difficult to conceptualize. Meeting SIRS criteria may be better used as a screening tool, to identify patients with suspicion of systemic infection (pre-sepsis) during the initial work-up and early treatment phase. The qSOFA or SOFA might then be applied to trend response, identifying patients with community-onset and ICU-onset sepsis, respectively.
Mandatory sepsis response
In 2015, the centers for Medicare and Medicaid Services (CMS) instituted required reporting of adherence to sepsis response protocols, the Severe Sepsis and Septic Shock Early Management Bundle (SEP-1) [4]. The bundle emphasizes early detection of sepsis using the Sepsis-2 definition, advocates for starting broad-spectrum antibiotics within 3 h, and requires serial measurements of serum lactate to monitor response to therapy [4]. While intended to standardize a response and thereby curb mortality, data has shown that the implementation of these mandatory responses has not met its goals. An analysis by Rhee and colleagues [18] demonstrated that the SEP-1 did not improve in-hospital mortality or discharge to hospice. Another study by Barbash and colleagues [19] also showed no improvement in in-hospital mortality, and additionally demonstrated no significant improvement in ICU admission or discharge home. Rhee and colleagues’ analysis [18] also documented an important finding of continued increase in broad-spectrum antibiotic use, including anti-pseudomonal and anti-methicillin resistant Staphylococcus aureus (MRSA) agents. Both studies demonstrated compliance with the SEP-1 guidelines, by documenting increased serum lactate measurements and timely antibiotic initiation. However, the institution of a strict logarithmic response to sepsis, then defined by Sepsis-2, did not significantly improve outcomes, nor did it have a positive impact on stewardship efforts [18, 19].
Beyond the old and new definitions of sepsis
The purpose of Sepsis-1 and Sepsis-2 definitions
When reading the previous definitions of sepsis, it is important to consider the purpose behind them. Sepsis-1 sought merely to define sepsis, but Bone and colleagues’ writing [8] implies an overall concern for the identification of patients with systemic infections, particularly BSI. The use of the outdated terminology “septicemia” at the time interchangeably with bacteremia and BSI was likely the driver for adopting the SIRS criteria to identify patients with sepsis. SIRS included a mix of criteria that represented physiologic response to systemic infection (fever, tachycardia, leukocytosis) and dysregulatory phenomena (hypothermia, leukopenia, and to some extent respiratory distress). Sepsis-2, while formally changing little, shifted focus toward dysfunction and expanded consideration of additional data points.
Even 30 years later, the SIRS criteria continue to perform well for what they were initially designed for, identification of patients with gram-negative BSI. A recent large cohort study demonstrated that 93% of hospitalized patients with gram-negative BSI had ≥ 2 SIRS criteria at initial presentation [10]. This supports the current clinical practice of obtaining two sets of blood cultures in all patients who meet the SIRS criteria prior to initiation of antibiotic therapy. However, not all BSIs are immediately life-threatening. For example, patients with BSI due to viridans group Streptococcus species usually have subacute presentation with fever, fatigue, weight loss, and anemia over several weeks, hence the term subacute bacterial endocarditis. Similarly, many patients, particularly young women, with E. coli BSI secondary to acute pyelonephritis may have fever, chills, flank pain, and urinary symptoms for several days prior to presenting to medical care. Most of these patients meet the SIRS criteria. However, their short-term prognosis is excellent in the absence of life-threatening symptoms and signs such as hypothermia, hypotension, respiratory failure, or altered mental status [10].
Does the Sepsis-3 definition represent a novel or revolutionary concept?
Multiple acute severity of illness scores have been used to identify patients with BSI who are critically ill. The most robust and commonly used score is the Pitt bacteremia score (PBS) which was proposed in 1989 [20]. Even among patients with gram-negative BSI, only one-third overall meet the criteria for critical illness with PBS ≥ 4 [9, 10, 21, 22]. Since hypotension, respiratory failure, and stuporous mental status are each assigned 2 points in the PBS, patients may be classified as critically ill by meeting two of the three criteria [23]. Additionally, patients may meet the criteria for being critically ill by having either coma or cardiac arrest (4 points each). Critically ill patients with PBS ≥ 4 have much higher mortality than those with PBS < 2. For simplification, a qPitt was derived and validated to predict mortality in patients with BSI [10, 17]. The qPitt consists of five clinical variables each assigned one point (hypothermia, hypotension, respiratory failure, cardiac arrest, and altered mental status) [10, 17, 23].
The PBS provides more than just prognostication. It identifies life-saving opportunities with appropriate empirical antibiotic therapy. Among a cohort of patients with gram-negative BSI, a survival benefit from appropriate empirical antibiotics was demonstrated only in those who met the criteria for being critically ill (PBS ≥ 4 or qPitt ≥ 2) [10, 24]. Mortality was 50% lower in patients who received appropriate empirical antibiotics compared to those who received inappropriate empirical therapy. On the other hand, mortality was < 5% in non-critically ill patients with gram-negative BSI, with no statistical difference between those who received appropriate and inappropriate empirical antibiotic therapy [10, 24].
Practical limitations of Sepsis-3
While many studies have commented on the implementation of Sepsis-3, there has yet to be a broad comparison of clinical outcomes [25–27]. Evaluation is certainly limited by the non-universal adoption of the 2016 definition. As such, the clinical impact of the Sepsis-3 criteria has yet to be determined. A recent study by Engoren and colleagues [28] compared mortality effects by the changed definition and found such discordant results between SIRS and SOFA that they suggested the existence of different sepsis syndromes. Engoren et al. [28] also found improved mortality outcomes if using both SIRS and SOFA scores in concert. Other studies have also suggested that utilizing both scores together would improve diagnosis of sepsis [29]. Given SOFA/qSOFA’s demonstrated validity in identifying critically ill patients, one would have expected at least a decrease in broad-spectrum antibiotic use, as those not meeting Sepsis-3 criteria would not require them, but this was not demonstrated. The Infectious Disease Society of America’s (IDSA’s) position paper on SEP-1, in fact, expressed concern that mandatory sepsis bundles would exacerbate broad-spectrum antibiotic use [30].
Potential progression from pre-sepsis to sepsis and septic shock
Despite the numerous attempts to categorize sepsis based on specific criteria, it should be well understood that sepsis represents a continuum. Most patients initially complain of symptoms of localized infection (pain, swelling, and erythema of the skin suggestive of cellulitis, for example). If untreated, this may progress into a systemic infection (heralded by fever, tachycardia, and leukocytosis) such as BSI due to group A Streptococcus. If untreated, this may progress to sepsis (hypotension, respiratory distress, and altered mental status), septic shock (hypotension refractory to intravenous fluid resuscitation), and ultimately death. The speed at which patients enter the sepsis cascade and progress from one stage to another depends on a variety of host and microbial factors. The extremes of age, the number and advanced stages of chronic medical comorbidities, immune-compromised status, and disruption of normal anatomy represent the most obvious host variables. The proportional increase of mortality with advancing age in patients with BSIs suggests faster progression through the sepsis cascade [24]. Similarly, patients with end-stage liver disease (cirrhosis) and cancer had a five- and three-times higher risk of mortality following BSI, respectively, compared to those without these two comorbidities [21, 22]. The progression to septic shock in patients with anatomical or functional asplenia is often very rapid, particularly when infected with encapsulated bacteria such as Streptococcus pneumoniae. The presence of complete ureteric obstruction in patients with acute pyelonephritis, large common bile duct stones in acute cholangitis, or colonic perforation with secondary peritonitis in patients with colitis often lead to faster progression of systemic inflammation, sepsis, and septic shock. Bacterial virulence factors also play an important role in the rapid progression from pre-sepsis to sepsis and septic shock. The most common example is toxin production in invasive group A Streptococcus infections often leading to a short time between diagnosis of localized cellulitis progressing to septic shock from necrotizing fasciitis.
One recent study examined the rate of progression from systemic inflammation and sepsis to septic shock in hospitalized patients with suspected infections in whom cultures were obtained and empirical antibiotics were initiated afterwards [31]. In this cohort, progression to septic shock occurred significantly faster in patients with sepsis (qSOFA ≥ 2) than in those with systemic inflammation or pre-sepsis (SIRS ≥ 2) with a median time of 11 h vs. 26 h, respectively [31]. Moreover, there was a 4% increase in the risk of progression to septic shock with each 1-h delay in starting appropriate antibiotics [31].
The identification of robust clinical and laboratory biomarkers that predict the rate of progression from pre-sepsis to sepsis and septic shock would be highly valuable. Currently, serum lactate level is the most widely used test as a surrogate for septic shock. It has been used to identify patients towards the end of the sepsis cascade. However, it does not provide an early assessment of the probability of progression to septic shock in patients with systemic inflammation or sepsis. The wider availability of serum interleukin levels and other biomarkers after the coronavirus disease 2019 (COVID-19) pandemic may help advance this field.
Maximizing benefit-to-risk ratio of empirical antibiotic therapy
A pandemic of broad-spectrum antibiotic overuse
As awareness of broad-spectrum antibiotic overuse is becoming more common, it is prudent to move towards an evidence-based approach to empirical antibiotic therapy. No empirical regimen is going to provide 100% coverage for all potential pathogens and resistance mechanisms. In addition, starting every patient with suspected infection on a broad-spectrum regimen will likely lead to unfavorable benefit-to-risk ratios. For example, in patients with suspicion of systemic infections without life-threatening criteria (SIRS ≥ 2, but qSOFA < 2 or qPitt < 2), there may not be a survival benefit from appropriate empirical antibiotic therapy [10, 24]. The risks of starting every single patient with pre-sepsis on broad-spectrum regimens of anti-pseudomonal and anti-MRSA agents (C. difficile infection, nephrotoxicity, other antibiotic adverse events, and selection of antibiotic resistance) may be difficult to justify when weighed against limited potential benefits [32].
A study of culture-positive sepsis by Rhee and colleagues [33] demonstrated that inadequate empirical antibiotic therapy was associated with 20–40% increased mortality. Interestingly, overtreatment was also associated with 20% higher mortality in patients without septic shock. Applying these principles can have a measurable effect on antibiotic use and adverse outcomes, such as C. difficile infection. For example, Hiensch and colleagues [7] documented an increased use of broad-spectrum antibiotics and C. difficile infections following the implementation of an electronic medical record integrated sepsis protocol. Rhee and colleagues [18, 33] documented a 26% increase in the rates of C. difficile infection with overtreatment given a continuing trend towards increased use of anti-pseudomonal and anti-MRSA agents. Clearly, this one-size-fits-all approach of starting empirical anti-pseudomonal and anti-MRSA therapy in every patient with suspected infection has failed and an alternative approach should be sought.
Acceptable margins of error in empirical antibiotic therapy
Determining acceptable margins of error for empirical antibiotic therapy in patients with serious infections is a well-known concept. Johnson and Russo [34] proposed an empirical regimen with ≥ 90% susceptibility in hospitalized patients with acute pyelonephritis to be modified based on patient-specific markers of severity. Haggard and colleagues [35] surveyed 316 infectious diseases and critical care pharmacists, and found they were most comfortable using empirical antibiotics regimens with overall susceptibility of 90–95% in sepsis and ≥ 95% in septic shock. Nimmich and colleagues [36] utilized the above margins of error to develop institutional management guidelines for gram-negative BSI using cumulative bloodstream antibiograms. However, there are very few examples in the literature where these acceptable margins of error have been incorporated into evidence-based management guidelines for serious infections.
Utilization of precision medicine concepts in selection of empirical antibiotic therapy
In addition to acute severity of illness, the risk of multi-drug resistant bacteria must also be taken into consideration. This risk is better assessed based on patient-specific risk factors for resistance rather than a hospital antibiogram. For example, in a geographical location with an overall prevalence of extended-spectrum beta-lactamase (ESBL)-producing bacteria of 10%, this risk may vary vastly from one individual to another based on prior antibiotic use and other healthcare exposures. Assessment of the predicted risk of ESBLs in each individual utilizing precision medicine concepts is superior to the simple assumption that each patient presenting to medical care has a predicted risk of infection due to ESBL-producing bacteria of 10%. Augustine and colleagues [37] demonstrated that the predicted risk of BSI due to ESBL-producing bacteria ranged from < 1% in those without risk factors to > 25% in the presence of major risk factors and > 50% in patients with multiple risk factors for ESBLs. Similarly, Hammer and colleagues [38] demonstrated that the predicted risk of BSI due to Pseudomonas aeruginosa varied between < 1% in the absence of risk factors to 8%, 24%, and 45% in the presence of one, two, or three risk factors, respectively.
Proposed management algorithm
We propose the following management algorithm based on the understanding of infection as a continuum and considering the advantages and potential limitations of SIRS and qSOFA. The management algorithm utilizes precision medicine concepts and clinical tools for prediction of antimicrobial resistance to maximize benefits from empirical antibiotics and minimize potential harms (Figure 1).
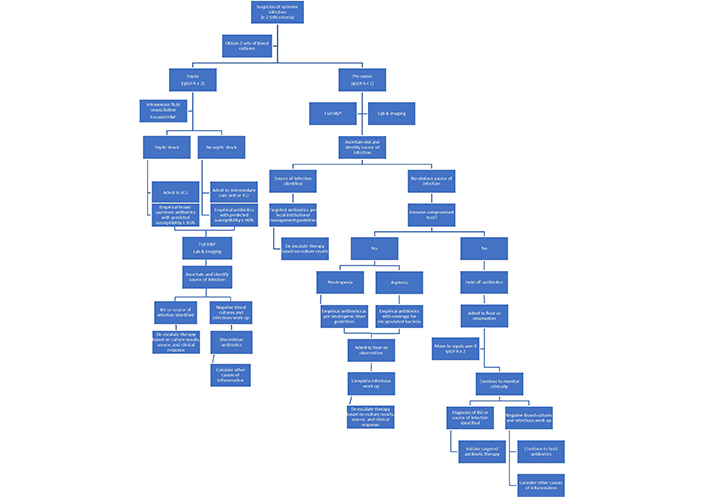
Proposed management algorithm in patients with suspected systemic infections. H: history; P: physical examination
The SIRS criteria are used as a screening tool to identify patients with suspected systemic infections. Two sets of blood cultures are obtained in all patients who have ≥ 2 SIRS criteria, given the high sensitivity of these criteria to identify patients with BSI. An urgent approach is taken in patients with sepsis (qSOFA ≥ 2) and septic shock, given the potential survival benefit from appropriate empirical antibiotics in patients with potentially life-threatening infections [10, 24]. After a focused history and physical examination to identify the source of infection, empirical antibiotics are started in these patients with different thresholds for predicted susceptibilities based on patient-specific risk factors. Source control should be achieved quickly to reduce the burden of infection and obtain clinical samples from that site for traditional cultures and/or other microbiological tests. Early de-escalation of antibiotic therapy based on blood and other clinical cultures, or rapid diagnostic testing results, is advocated to reduce the risk of C. difficile infection and other antibiotic adverse effects [39]. Antibiotic therapy may be discontinued in patients with negative blood cultures and unremarkable infectious diseases work-up. Alternative causes of inflammation should be considered in these patients. As the cost of rapid diagnostic technology declines and its use increases world-wide, the antibiotic stewardship practice of early de-escalation of therapy will become more prevalent [40]. This may eventually reverse the current trends of increasing broad-spectrum antibiotic use and reduce the rates of hospital-onset C. difficile infection [41].
In patients with pre-sepsis (qSOFA < 2), more time is available to perform full history, physical examination, laboratory, and imaging work-up to ascertain the risk and identify the potential source of infection. Empirical antibiotics are not immediately indicated in immune competent hosts without an identified source of infection. Importantly, patients should be monitored closely and moved to the sepsis arm of the algorithm if qSOFA score reaches 2 at any time. Targeted antibiotic therapy is initiated in patients with BSI or an established source of infection (pneumonia, cellulitis, etc.) based on institutional management guidelines for that particular syndrome. Providing source-directed therapy aims to reduce the adverse effects of broad-spectrum antibiotics while not compromising care in the critically ill or immune compromised hosts. Holding off antibiotic therapy in patients without sepsis and no identified source of infection during the initial evaluation avoids unnecessary use of antibiotics in low-risk patients and addresses the poor specificity of the SIRS criteria to identify systemic bacterial infections.
Conclusions
Sepsis remains a challenging condition to diagnose and manage, portending high mortality, particularly among patients with septic shock. The definitions of sepsis have evolved overtime, with improved diagnostic capacity and understanding of pathophysiology. However, the dogmatic application of outdated definitions and standardized responses has not produced the hoped-for improvement in outcomes. In order to respond to patients with all due rapidity and aggressiveness, while not compromising important antimicrobial stewardship efforts, practice modifications are necessary. Just as important, globally accessible definitions and standards should be implemented, allowing the diagnosis and immediate response to sepsis to be universally available, regardless of locale or level of medical resource. Utilization of precision medicine concepts and patient-specific risk factors for infections due to resistant bacteria improve the benefit-to-risk ratio of empirical antibiotic therapy across the spectrum of sepsis.
Abbreviations
BSI: | bloodstream infection |
C. difficile: | Clostridioides difficile |
ESBL: | extended-spectrum beta-lactamase |
ICU: | intensive care unit |
MRSA: | methicillin resistant Staphylococcus aureus |
PBS: | Pitt bacteremia score |
qPitt: | quick Pitt bacteremia score |
qSOFA: | quick sequential organ failure assessment |
RR: | respiratory rate |
SEP-1: | Septic Shock Early Management Bundle |
SIRS: | systemic inflammatory response syndrome |
SOFA: | sequential organ failure assessment |
WBC: | white blood cell |
Declarations
Author contributions
MA contributed to the conception of the article and literature review. ND created the figure and supplemented the literature review. Both authors were involved in the drafting and editing of the manuscript.
Conflicts of interest
Both authors declare no potential conflicts of interest related to this work.
Ethical approval
Not applicable.
Consent to participate
Not applicable.
Consent to publication
Not applicable.
Availability of data and materials
Not applicable.
Funding
Not applicable.
Copyright
© The Author(s) 2022.