Abstract
Natural antioxidants, such as phenolic compounds, carotenoids, vitamins, and microelements, are predominant in fruits, vegetables, herbs, and spices. The accretion interest of consumers in utilizing natural ingredients in food products, have accelerated the appeal for functional “natural” operations. Therefore, understanding how natural antioxidants especially nano-antioxidants, and their delivery systems when used in antioxidant polymers for food packaging are extracted from natural sources, would help prevent oxidation reactions. Given the increasing role of natural antioxidants in the daily lives of today’s communities, a continuous synthesis of relevant literature is pertinent. To supplement existing information, recent advances in nano-related natural antioxidants, their extraction methods and applications in the food industry are discussed in this current work. Insightfully positioning antioxidants within the nano-delivery systems, this current work reveals the potential nanotechnology provides in enhancing the absorption of antioxidants in human metabolic systems.
Keywords
Natural antioxidants, nano-antioxidants, novel extraction methods, active packagingIntroduction
The awareness of consumers about the health benefits of foods has increased recently. They have a good knowledge of food composition and the impacts of it on the human health. One of the most vital ingredients is antioxidants. Formerly, antioxidants were incorporated into food products to impede oxidation and extend shelf life; however, in contemporary times, their application has shifted towards leveraging their health-promoting properties. Natural antioxidants are bio-sources predominant in plants, edible vegetables, herbs, fruits, and spices, high in phenolics, carotenoids, vitamins, and microelements [1–3]. However, the antioxidant activity of natural resources varies by morphological component. The climatic/soil conditions, as well as the time of harvest of such natural products, contribute significantly to their activity, which impedes their product standardization. Moreover, natural antioxidants would prevent the oxidation processes and microbial proliferation from pathogenic ones like Salmonella spp. and Escherichia coli [4], given their increasing role as food packaging ingredients/product preservatives [5, 6]. Natural antioxidants would function as additives in meat products [7, 8] and even bakery products [9] to successfully replace artificial preservatives/stabilizers. Besides, more publications in recent years show the potential of natural antioxidants to help stabilize edible oils [10–12], carotenoid dyes, and the aroma of fruit juices [13]. The strategy to utilize processing industry by-products currently exhibits an intriguing trend [14, 15], which would be attributable to the increased bioactive substances, such as phenolic compounds from flavonoids (anthocyanins, catechins), polyphenols in apple/olive pomace [16], lycopene in tomato pomace [17], and phenolic acids/stilbenes either in grape skins [18] or citrus fruits [19].
An imbalance between antioxidants and free radicals can lead to oxidative stress, which would affect macromolecules like proteins, lipids, DNA, and subsequently cells/tissues [20]. Unsaturated fat oxidation and chemical oxidation can be significantly slowed by antioxidants, and the increased (antioxidant) capacity of various foods, therefore, makes such combination more effective [21]. The use of synthetic supplements in the food industry raises numerous concerns due to chemical contamination, the production of hazardous compounds, and toxic solvents. However, the application of natural antioxidants originating from organic resources is becoming prevalent [22]. For emphasis, antioxidants such as vitamins, phenolic compounds, and carotenoids, are abundant in spices, herbs, fruits, and vegetables, and are able to reduce cardiovascular disease risks and some types of cancer, which in turn improves health and quality of life [23, 24]. More so, natural antioxidants are classified as exogenous and endogenous. Specifically, endogenous antioxidants are made in the body like ubiquinone, metallothioneins, albumin, uric acid, and glutathione [25]. Exogenous antioxidants are originated from plants such as phenolic acids, flavonoids, stilbenes, carotenoids, lignans, coumarins, and vitamins. Polyphenols, like phenolic acids, flavonoids, stilbenes, and lignans are abundant in foods and herbs [26]. Endogenous antioxidants in foods can typically be significantly depleted during the production, processing, and storage, reducing their own ability to mitigate lipid oxidation. Moreover, antioxidants can scavenge free radicals in a variety of ways due to their positions (ortho, meta, and para) and active groups [27]. Further, antioxidants are also pro-oxidant quenchers, hydrogen peroxide decomposers, metal ion transistors, and radical scavengers. Indeed, antioxidants can be categorized as primary (free radical scavenger, chain breaker) or secondary (preventive) [28]. While primary antioxidants readily prevent oxidation by scavenging reactive oxygen species (ROS) and reactive nitrogen species (RNS), secondary antioxidants function by chelating different metal ions [29]. Moreover, exogenous antioxidants’ health benefits have been investigated through in vitro tests. Antioxidants’ high effectiveness involving in vivo conditions would not happen due to in vitro experiments. Additionally, antioxidant supplements may not always work very well and could cause harm if taken in large quantities [30, 31]. For instance, Eidelman et al. [32] reported the use of vitamin E to treat cardiovascular disease was ineffective. Elsewhere, such antioxidant supplements were reported as capable of exacerbating cancer [33]. Elsewhere also, an α-tocopherol β-carotene cancer prevention study group found that smokers were 18% more likely to develop lung cancer when they consumed β-carotene more than ten times per day [34]. Natural antioxidants are metabolized in vivo to a great extent due to the reduction of redox potential at a physiological extent [35].
Phenolic acids include cinnamic acid derivatives such as p-coumaric, caffeic, and ferulic, and benzoic acid derivatives like gallic acid (GA) and hydroxybenzoic acids. Caffeic acid is the most abundant phenolic acid, accounting for 75–100% of the total hydroxycinnamic acid content in numerous fruits, while ferulic acid is the most plentiful phenolic acid in cereals and represents about 90% of total polyphenols in wheat cereals. Flavonoids are abundant in the majority of edible fruits and vegetables. Flavonoid subclasses comprise flavonols, flavanones, flavones, catechins, isoflavonoids, and anthocyanidins. Flavonoids vary in concentration/type from one food source to another [36]. For instance, quercetin is generally the most plentiful flavonol in edible herbs. Onion is among promising source of quercetin. The presence of anthocyanins could be attributed to red, blue, or purple colors in foods such as berries, eggplants, and plums, including cyaniding, delphinidin, and pelargonidin. The isoflavonoids, like isoflavones, genistein, and daidzein, are primarily present in legumes, like soybean and soya-based products [36, 37]. The value of antioxidants has been shown in the treatment of neurological conditions arising from coronavirus disease 2019 (COVID-19) [38]. Indeed, the tackling of emergent infections from COVID-19 revealed the effectiveness of flavonoids like (+)-catechin, apigenin, quercetin, quercetin 7-rhamnoside, and luteolin on porcine epidemic diarrhea virus (PEDV), transmissible gastroenteritis virus (TGEV) [39–41]. Natural pigments (carotenoids) include β-carotene, zeaxanthin, lycopene, and lutein [42]. Moreover, β-carotene appears most frequently found in edible plants with the highest pro-vitamin A activity, like carrots, acerola, nuts, oil palm, and mango. Besides, lycopene can be sourced mainly from tomato products of soups, juices, ketchup, and sauces [43]. Therefore, it is pertinent to understand how natural antioxidants are extracted especially nano-antioxidants and their delivery systems, as well as how natural antioxidants would help prevent oxidation reactions, antioxidant polymers, etc. Given the increasing role of natural antioxidants in daily living across the communities of today’s globe, there is a need to continually perform a synthesis of relevant literature. To supplement existing information, recent advances in some nano-related natural antioxidants, their extraction methods and contributions to the food industry have been discussed in this paper, drawing from the following: (a) extraction methods to recover antioxidants from by-products; (b) nano-delivery systems and nano-antioxidants; (c) application of natural antioxidants to prevent oxidation in foods; (d) different types of antioxidant polymers; and (e) continued progress of antioxidant active food packaging.
Extraction methods of by-products to recover antioxidants
The first and most important step in studying plants’ natural antioxidants is extraction. The type and concentration of the extraction solvent, the extraction temperature, the extraction time, and the extraction pH are just a few of the extraction factors that have a significant impact on the efficiency of the extraction. Some challenges still confront the traditional methods of extraction, for instance, the lengthened completion time, laborious nature, and limited selectivity or extraction yields. Additionally, these traditional methods make extensive use of toxic solvents. Further, the chemical structure and polarity of the antioxidant composition have to be extracted in order to determine the solvent selection. The majority of the phenolics, anthocyanins, and flavonoids are antioxidants that dissolve in water. Extraction frequently involves the use of polar and medium-polar solvents like water, methanol, ethanol (ET), acetone, propanol, and their mixtures of water [44–47]. Non-conventional techniques like ultrasound, microwave, pulsed electric field, pressurized liquid, high-voltage electrical discharges (HVED), enzyme hydrolysis, supercritical fluids (SF), subcritical water, and high hydrostatic pressure (HHP) extractions have been investigated for the purpose of gaining antioxidants from plants in a way that is both cost-effective and environmentally friendly. The following subsections will tersely show some progress of the above-mentioned non-conventional methods.
Ultrasound-assisted extraction
Ultrasound-assisted extraction (UAE) can considerably aid the extraction operation by generating “cavitation effects” to break down the tissue or cell [48]; thus, the mass transfer between the solution and the cell is remarkably amended leading to a conservation of time and energy [49]. Utilizing both solvents and acoustic energy, the UAE method targets compounds from a variety of plant matrices. Besides, ultrasound irradiation has a significant impact on the diffusions that happen through the cell walls and washing out (rinsing) the contents of the cell after the walls are broken [50]. Further, the variables of time, temperature, intensity, and frequency of ultrasound can directly influence extraction efficiency/yield. Effective extraction also depends on the characteristics of the sample, such as its moisture content and particle size, as well as the types of solvents used [51]. Sonic cavitation is the main operating mechanism in the UAE, which is capable of generating a series of compression waves in the liquid solvent molecules, leading to the creation of bubbles due to changes in temperature and pressure. Many systems have been found to be implemented in the UAE as a whole. Separation resulting from collisions between particles and ultrasonic waves, results in a reduction in particle size and thus increases mass transfer [52]. Espada-Bellido et al. [53] used ultrasound to extract anthocyanins and phenolic compounds from the pulp of the mulberry (Morus nigra). A few extraction factors, including methanol focus (half 100%), temperature (10°–70°C), ultrasound plentifulness (30–70%), cycle (0.2–0.7 s), dissolvable pH (3–7) and dissolvable strong proportion [10:1.5 to 20:1.5, (v:v)] were enhanced to bring about the great extraction efficiency. For the extraction of anthocyanins and phenolic compounds, the parameters of most influence include extraction temperature and concentration of solvent. In addition, the progress of generating various bioactive components would favor UAE conditions. For instance, anthocyanins require a methanol concentration of 76%, a solvent-solid ratio of 12:1.5 (v:v), an extraction temperature of 48°C, a cycle time of 0.7 s, and a 70% ultrasound amplitude at pH = 3. Phenolic compounds require a methanol concentration of 61%, a solvent-solid ratio of 11:1.5 (v:v), an extraction temperature of 64°C, a cycle time of 0.7 s, and a 70% ultrasound amplitude at pH = 7. Under the UAE ideal circumstances, the augmented extraction yields of absolute anthocyanins and total phenolic compounds were 149.95 μg/g and 1,214.03 μg/g, separately. Bonfigli et al. [54] compared ultrasound extraction of grape pomace anthocyanins to conventional extraction. Water and 50% ET were combined. Both methods were evaluated, and it was found that UAE increased the mass transfer rate during the washing stage of anthocyanin extraction. In contrast, the UAE method extracts 90% of anthocyanins at the same time while conventional solvent extraction (CSE) extracts 80% of them in the first 600 s. The structure of materials, as well as their physical and chemical characteristics, are altered by ultrasound waves, which produce cavities within the cell wall(s). Consequently, releasing bioactive compounds, and increasing mass transfer should occur while decreasing extraction over time. As extraction yield increased with temperature, the role of UAE in generating polyphenols has been the subject of debate by many researchers. Numerously, ultrasound would enhance the extraction of bioactive compounds like polyphenols from various plant by-products [55–58].
Microwave-assisted extraction
The electromagnetic waves that make up microwaves typically operate at a frequency of 2.45 GHz. The microwave-assisted extraction (MAE) method incorporates the heating effect of microwaves, making the extraction temperature higher and resulting in faster transfer rates [59]. Microwaves can also cause direct/massive heating of the solvent body and sample matrix due to microwave penetration into certain materials to a certain depth and their interaction with polar properties [60]. When compared to traditional extraction techniques, MAE can speed up the extraction process [61–63]. In recent years, there has been ample progress in numerous studies about the MAE of antioxidants from plant materials [64–67]. Considering the thermal effect of microwave irradiation, MAE appears unable to adapt the extraction of thermally labile antioxidants, which could reduce the extraction yield. Additionally, MAE can only be used with extraction solvents that absorb microwaves. The treatment time, temperature, and microwave energy all have an impact on MAE efficiency. Hayat et al. [68] described the effects of microwave energy and treatment time on the extraction of phenolic compounds from citrus mandarin peel and pomace. While the bound phenolic content decreased, the concentration of free phenolics in the extracts significantly increased with microwave energy/time. By the microwave treatments, some bound phenolics in the tissue matrix appear cleaved and released, allowing their free-form presence in the extracts. However, some flavonol compounds would degrade when the treatment time progressed with microwave energy. More so, the few benefits of MAE might corroborate ordinary extraction strategies, for example, higher extraction yield, less dissolvable utilization, and more limited extraction time [69, 70]. Further, MAE has merits over traditional maceration or potentially strong/fluid extraction, for example, a substantial decrease of extraction time while acquiring comparative or higher extraction proficiency. The deterioration of cell layers in plant materials by pulse electric field (PEF) innovation, which was proposed as a non-warm extraction technique, could improve the extraction yield of bioactive particles from plants. The pulsed electric fields method uses short pulses with a high electric field intensity (0.1–50 kV/cm) at room temperature [71].
PEF
In PEF, a material situated between two cathodes reflects the areas of strength for a field. Pore formation can occur if the membrane is challenged with sufficient stress from the electrical field. Depending on the PEF treatment conditions, such as electric field strength, number of pulses, and pulse duration, pore formation can be reversible or irreversible. Cell permeability is increased when pores form [72]. Other workers, like Zderic and Zondervan [73] showed the addition of temperature would not surpass 10°C during the extraction of polyphenol from new tea leaves utilizing PEF. Corrales et al. [74] showed that non-thermal conditions provided by pulsed electric field processing increased slightly the temperature. When PEF served as a pre-treatment at 25°C/min alongside conventional thermal extraction at 70°C/h, the concentration of anthocyanins in the red grape by-product showed a 60% increase. The extraction of 10% more polyphenols was shown from white grape skins treated with PEF at 20°C [75]. Although industrial-scale equipment is still in improvement and the procedure does not utilize solid products, PEF appears to be a potential method for pre-treatment in the extraction of polyphenols from by-products [76]. Besides extraction of antioxidants from food and medicinal plants, like Norway spruce bark’s polyphenols [77], the PEF technology has the capacity to improve extraction efficiency, for instance, anthocyanin from by-products of blueberry processing [78]; ellagic acid and quercetin from the juice of Emblica officinalis [79]; betanins from beetroot (Beta vulgaris L.) [80]; carotenoids from date fruit (Phoenix dactylifera L. Sukkari) [81]; carotenoids from tomato (Solanum lycopersicon) [82]; and chlorophylls from custard apple (Annona squamosa) leaves [83]. Solute extraction relies heavily on the strength of the electric field. Ozkan et al. [84] examined the effects of high-pressure processing (HPP: 200 MPa, 400 MPa, and 600 MPa at 5/15 min) and PEF (3 kV/cm, 5 kJ/kg, 10 kJ/kg, and 15 kJ/kg) treatments on the antioxidant capacities and total phenolic, total flavonoid, and total anthocyanin content of cranberry purees. Expanded pressure levels and longer length times in HPP, and expanded explicit energy input values in PEF are not entirely set in stone to prompt higher absolute phenolic and complete flavonoid levels. In any case, the PEF treatment performed at 15 kJ/kg at a specific energy input level yielded greater qualities compared to the crude puree although a slight, but not statistically significant trend occurred. The considerable phenolic compound in cranberry bush fruit, chlorogenic acid, increased in both the HPP and PEF treatments by 6–7% and 5.6–11%, respectively. Although HPP did not significantly alter antioxidant volume, PEF treatment at 15 kJ/kg significantly increased antioxidant volume, which may be attributable to the samples’ greater amounts of phenolics and flavonoids. It was hypothesized that the improved extractability of phenolics as a result of progressed cell penetrability and the potential inactivation of the endogenous deteriorative enzymes were the causes of the slight increments that were observed in HPP-treated and PEF-treated samples.
Pressurized liquid extraction
Pressurized liquid extraction (PLE) is another name for accelerated solvent extraction (ASE), and both employ solvents at higher temperatures/pressures to make for a more effective/sped-up extraction process. PLE may offer a more efficient alternative to solvent-based methods for the extraction of anthocyanin and other bioactive compounds from plant sources. Specifically, the liquid solvent is subjected to high temperatures and pressures of 4 MPa to 20 MPa in the PLE method. Even when the solvent is heated beyond its boiling point, this pressure maintains its liquid state [85, 86]. In a pressurized environment, pores allow the solvent to diffuse into plant tissues and come into contact with the desired molecules, increasing extraction recovery [87]. In a study conducted by Truong et al. [88], the researchers investigated the extraction of anthocyanins from different varieties of purple-shed sweet potatoes using PLE. To extract anthocyanins, acidified methanol was used on freeze-dried powder obtained from purple-eyed sweet potato. The PLE condition included the use of acetic acid and methanol with a water mixture of 7%:75%:18% (v:v:v). The highest recovery of anthocyanins was obtained at temperatures ranging from 80°C to 120°C using this extraction condition. On a dry and weight basis, the anthocyanin contents of 335 genotypes ranged from 0–663 mg/100 g and 0–210 mg/100 g, respectively. Blackberry, jabuticaba, blueberry, and black bean have all been successfully recovered using PLE [89–92]. Nevertheless, anthocyanin degradation may occur during the extraction process due to the high temperatures of the process. However, the absence of oxygen limits the occurrence of oxidation reactions during PLE because it is carried out in a closed system. Garcia-Mendoza et al. [93] utilized four distinct solvents which were assessed in PLE: 99.5% ET; ET and water (1:1, v/v) in an acidified mixture with a pH of 2 (EW), distilled water (DW), water with an acidic pH of 2 (AW). While pressure (10 MPa) and flow rate (1.5 mL/min) remained constant, three distinct temperatures (40°C, 60°C, and 80°C) were examined. By increasing their diffusion and solubility in various solvents, the temperature rises in PLE enhanced the recovery of phenolic compounds. Low extraction yields were the consequence of anthocyanins being thermally broken down at temperatures higher than 40°C. There were significant correlations between the total phenolic content (TPC) and the PLE extracts.
HVED
The application of HVED has improved the extraction of soluble materials from water. Vegetable materials such as sesame seeds, grape stems, sugar beet pulp, and peanut shells have been used to apply this technology [94–97]. When a high voltage is applied between two electrodes, the electrons are accelerated and gain sufficient energy to excite molecules of water. Following that, a streamer, or electron avalanche, is created. If a sufficient electric field is applied, the streamer travels from the positive electrode to the negative one. The electrical breakup occurs when one of the streamers reaches the negative electrode. High-amplitude pressure shock waves, bubble cavitation, and liquid turbulence are all produced by electrical breakdown. Particle fragmentation and damage to the cell structure are the results of these phenomena that speed up the process of extracting compounds from within cells. HVED is a green extraction method because it can increase the rate of compounds extracted in a shorter amount of time and with less energy, temperature, and input [96]. Boussetta and Vorobiev [98] investigated the effects of HVED on grape (Vitis vinifera L.) residue fluid polyphenol extraction at mild temperatures (20°–60°C). The results showed that in comparison to the yield of 30% obtained after 240 min without HVED extraction, 70% of the extracted solutes from fresh grape residues after 40 min of HVED extraction were more than twice as huge. Removing HVED after 1 h also increased the polyphenol yield (Yieldpolyphenols = 0.44% ± 0.07%) in comparison to the extraction without HVED after 4 h (Yieldpolyphenols = 0.26% ± 0.06%). Nevertheless, there may be side effects to this approach. According to Boussetta et al. [99], using HVED can result in the production of extremely minute fragments, which can make the subsequent step of separating liquid from solid more challenging.
Enzyme-assisted extraction
Due to the low extraction conditions and minimal environmental impact, enzyme-assisted extraction (EAE) is a possible green extraction technique [100], which favors compounds’ release being trapped, and dependent on enzymes’ capacity to either degrade or disorder complex substances within the cell wall. The basic elements impacting extraction proficiency and yield are the categories of enzymes, incubation temperature, concentration, incubation time, particle size, pH, and liquid-to-solid ratio [101]. The extraction efficiency is affected by the concentration of the various enzymes that degrade various substrates. According to Garg et al. [102], pectinases are a group of enzymes that catalyze the breakdown of pectic polymers in plant cell walls. Cellulases depolymerize cellulose into fermentable sugars [103], and tannase hydrolyzes ester and depside bonds in hydrolysable tannins to produce glucose and GA [104]. As a result, it is anticipated that each enzyme action will result in a selective enhancement of distinct phenols in the extract. Additionally, different enzymes have different pH requirements for enzymatic hydrolysis. The decomposing of some bioactive components, like anthocyanin, is influenced by the pH as well as the activity of enzymes. In addition, during processing, the temperature, incubation period, and liquid-to-solid ratio should all be taken into account. Thus, the optimal extraction parameters for different plant materials and enzymes vary. In one study, pectinase, α-amylase, protease, and β-glycosidase were combined to extract phenolic compounds from watermelon skin using an EAE technique. Four factors were investigated in order to obtain the maximum polyphenol yield: pH (6–9), temperature (25°–75°C), treatment time (30–90 min), and enzyme concentration (0–6.5%). Under optimal conditions (2.24% enzyme concentration, 51.8°C, pH = 6.58, 3 min), optimized EAE produced levels of 173.7 mg GA equivalents (GAE)/g fresh weight (FW, total phenolics), 279.96 mg trolox equivalents (TE)/g FW [trolox equivalent antioxidant capacity (TEAC)], and 112.27 mg/mL [2,2-diphenyl-1-picrylhydrazyl (DPPH) radical scavenging ability, half maximal inhibitory concentration (IC50)] that were three times greater than those produced by CSE [105]. Possibly, the response surface methodology method would have the capacity to maximize enzyme-aided phenolics and extraction yield. Indeed, optimizing the simultaneous enzymatic treatment could improve extraction of antioxidant extraction by above 60% and its recovery by about 80%. Indeed, besides the distinct enzyme effects in the extract, while gallic and syringic acid phenolic extract is enhanced by tannase, it is enriched in p-coumaric acid and malvidin-3-O-glucoside by cellulose [106]. Depending on the phenolic compound, the combination of the enzymes can result in either positive or negative effects. When it is necessary to selectively enrich particular compounds, this fact must be taken into account. For further increasing extraction efficiency, there is another technique known as EAE linked with ultrasound, microwave, alongside HHP extraction. For extracting tricin, a flavonoid, from rice hull, there is an enzymatic hydrolysis and HHP method [107].
SF extraction
A fluid becomes a highly critical fluid when it is heated to a pressure and temperature higher than its critical point. Under these circumstances, the fluid’s characteristics lie halfway between those of a gas and a liquid. SF extraction (SFE) has gained a lot of traction in recent years as an environmentally friendly and sustainable technology. To extract specific components from several matrices, SFE makes use of the exceptional physicochemical properties of SF. There are basically two major steps in SFE: the supercritical solvent is used to first extract the plant material’s soluble compounds, and then these compounds are separated from the supercritical solvent by quickly decreasing pressure, increasing temperature, or both [108]. SFE of caffeic acid derivatives, phenolic compounds, etc., obtained from food and medicinal plants have been reported [109–115]. Talmaciu et al. [116] investigated the effects of SFE, UAE, and traditional ethanolic extraction on the polyphenol extraction process from spruce bark, a waste product of the forestry industry. SFE was performed at 1,200 psi for 60 min with pure CO2 and 70% ET as a co-solvent. UAE was performed using 70% ET in an ultrasonic bath at 40°C for 60 min. Ethanolic extraction was achieved using 70% ET in an oven for 13 days at 40°C. Compared to UAE (33.48 mg GAE/g) and ethanolic extraction (14.38 mg GAE/g), the yield of total phenolic compounds from SFE was significantly higher (122.41 mg GAE/g). Furthermore, high-performance liquid chromatography (HPLC) analysis of compounds revealed that SFE extracts had a higher extraction yield of phenolic compounds than UAE extracts, including sinapic acid (35.05 mg/L), p-coumaric acid (260.41 mg/L), and catechin (171.18 mg/L).
Subcritical water extraction
Extraction with hot water and pressure has lately emerged as a useful alternative to traditional methods. PLE is typically referred to as subcritical water extraction (SWE), superheated water extraction, pressurized hot water extraction, or pressurized low-polarity water extraction, when only water is utilized as a solvent [117]. In order to keep water in a liquid state, SWE is performed with hot water (between 100°C and 374°C, the water critical temperature) and high pressure (typically between 10 bar and 60 bar). Depending on the temperature, the required pressure to maintain water in its condensed state is: for extraction at 300°C, a minimum pressure of 8.5 MPa is required, while a minimum pressure of 1.5 MPa is required for 200°C [118]. Of all the thermodynamical characteristics of water, the dielectric constant is one that changes significantly with increasing temperature. Dielectric constants are reached at 200°C that are similar to those of organic solvents such as methanol [119]. Hydroethanolic blends are the other fluid frequently utilized in PLE of bioactive components. The advantages of both can be used with solvent mixtures. For instance, one solvent, ET, can make the solute more soluble, and another, water, can help the solute desorb from the matrix [120]. The utilization of ET diminishes the boiling temperature and influences the solvent polarity. As a result, the amount of ET has a significant impact on the number of polyphenols that can be extracted [121]. The extraction yield is also influenced by temperature. Both the mass transfer and extraction rates can be increased by using a higher temperature. Monrad et al. [122], who found that a temperature of at least 80°C and a pressure of 6.8 MPa were necessary for an efficient procyanidin extraction from red grape pomace using PLE, validated the need for a high temperature. Due to the application of high temperatures (approximately 140°–150°C), certain compounds, such as Maillard reaction products and 5-(hydroxymethyl) furfural (HMF), can be generated de novo when using PLE [123, 124]. Additionally, sequential procedures can be utilized to increase extraction yields. For instance, SWE at 100°C, trailed by an extraction at 150°C was viewed as ideal to remove flavonols and GA [125]. Briefly, when extracting polyphenols from by-products, temperature, type of solute, and extraction medium were reported to be of major importance. Elmi Kashtiban and Esmaiili [58] discovered that SWE’s most effective variable for extracting the polyphenolic compounds from the grape skin was temperature. Additionally, they employed UAE as a pretreatment and discovered that the application of ultrasound as a pretreatment at 150°C, 40 bar, and 30 min yielded the highest levels of total phenolics, total flavonoids, antioxidant activity, and chroma.
HHP
Food products are subjected to pressures ranging from 100 MPa to 15,000 MPa with HHP technology. The mass transfer rate is increased and solvent penetration into cells through ruptured membranes is accelerated by the large differential pressure that develops between the interior and exterior of cell membranes under high pressure. Accordingly, the extraction yield will increase and more components will be released the higher the hydrostatic pressure [125]. The extraction efficiency can be improved by using more energy (higher pressure), but it can also cost more. Currently, industrial scale pressures up to 600 MPa are technically limited [126]. When extractions were aided by HHP, higher caffeine extraction yields from coffee and higher carotenoid content in tomato puree were demonstrated [127, 128]. Shouqin et al. [129] demonstrated that the use of HHP to extract flavanols from propolis is advantageous. The air gaps in fruit tissues are partially filled with liquid during HHP-assisted extraction. After that, the pressure is let go, and the air that was blocked in the pores escapes, causing damage to the plant cell membrane [130].
Corrales et al. [131] revealed that the highest antioxidant capacity was obtained at 70°C, 50% ET concentration, and 600 MPa high pressure, with anthocyanin recovery at 50°C being 23% higher than that of untreated treatments. Pomegranate juice’s anthocyanins produced a higher yield when they separated at 400 MPa and 25°C for 10 min as opposed to 500 MPa and 25°C for 5 min [132]. HHP was applied to the pulp of the raspberry puree in order to protect the anthocyanins and keep their color stable under a range of storage conditions. When HPP was applied to raspberry pulp at 200 MPa and 800 MPa, it preserved the pulp’s high color stability and anthocyanin concentration at 4°C as opposed to 30°C storage and ambient conditions [133]. Lonicera caerulea berry pulps had higher antioxidant capacities, phenol content, and anthocyanin content when low HHP was used for a longer period of time (400 MPa/20 min) as opposed to high HHP for a shorter period of time (600 MPa/10 min). At 200 MPa for 5 min and 10 min, the anthocyanin content increased by 6.84% and 14.35%, respectively. As a result, the extraction of anthocyanins could benefit from the improved long-term retention of bioactive compounds at low HHP [134]. Xi et al. [135] employed the HPP extraction method to extract polyphenols from green tea leaves. A variety of factors were assessed, such as the liquid/solid ratio [10:1 to 25:1 (mL:g)], pressure (100–600 MPa), holding time (1 min to 10 min), ET concentration (0% to 100%), and various solvents (acetone, methanol, ET, and water). The ideal extraction yield of polyphenols (13%) was obtained with 50% ET concentration, a liquid-to-material ratio of 20:1, and 500 MPa of HHP for 1 min. These conditions were similar to heat reflux extraction for 45 min, ultrasonic extraction for 90 min, and extraction at room temperature for 20 h, respectively. These data conveyed that the HHP extraction is more efficient contrary to the conventional extraction methods. In Table 1, the pros and cons of the different novel extraction methods are shown.
Comparison of advantages and disadvantages of different novel extraction methods
Extraction method | Advantages | Disadvantages | Reference |
---|---|---|---|
UAE | -Reduction of energy, time, and solvent consumption;-Safe, does not produce toxic compounds;-Higher penetration of solvent into cellular material and enhanced release in the medium. | -Increasing temperature by cavitation;-Mechanical forces generated by shockwaves and micro-streaming;-High power consumption;-Difficult to scale up. | [136] |
MAE | -Reduced extraction time;-The use of fewer chemical solutions. | -The need for special equipment;-Low selectivity;-Unavoidable reaction in high temperature. | [137] |
PEF | -Short processing time;-Increased efficiency;-Reduced energy input;-Preservation of the structure;-Higher quality of the end products. | -Dependence on the conductivity of the matrix composition;-High cost of the equipment. | [138] |
PLE | -Faster processes;-Less volume or organic solvent;-The possibility of automation;-Higher extraction yields. | -Higher initial investment cost and maintenance. | [139] |
HVED | -Simplicity of the HVED design;-Low power requirements reduce capital and operating costs;-It can be used to treat both solid and liquid foods. | -A large number of free radicals would be produced which leads to oxidative cell damage;-The produced radicals may also oxidize the target compounds and consequently decrease the yield. | [140] |
EAE | -Shorter extraction time with a high percentage of oil recovery;-Simplicity of process;-Cost-effectiveness. | -Enzymes are relatively expensive for large industrial production;-Available enzymes cannot break down the plant cell walls completely;-Enzyme-assisted extraction is not always feasible to be applied on an industrial scale because enzymes’ behavior is limited by environmental conditions rigidly. | [137] |
SFE | -Green extraction technique;-No need for organic solvent, maintain the quality of the final product;-Low operating temperatures (40°–80°C);-Very effective because of its low viscosity and high diffusivity;-Fast and high yield. | -No polar substances are extracted;-High power consumption;-The high cost of both the equipment and the supercritical fluid. | [136] |
SWE | -Extraction solvent (water) is green, cheap, and readily available;-High selectivity (polar, moderately polar, low-polar, and non-polar compounds);-Short extraction time and high efficiency. | -Dehydration or evaporation is needed;-Thermal degradation may occur at higher temperatures. | [141] |
HHP | -High pressure is not related to time/mass;-Does not break covalent bonds, so, the natural flavor of the products maintains. | -Food enzymes and bacterial spores are very resistant to pressure and very high pressure is required for their inactivation. | [136] |
Nano-delivery systems and nano-antioxidants
The consumption of antioxidants is an important issue that people nowadays are paying attention to and increasing their intake [142]. Many antioxidants have certain drawbacks, including low permeability, poor water solubility, instability during storage, and degradation in the digestive tract for example the acidic pH of the stomach’s mucosal lining causes to reduction in the absorption of antioxidants [143]. Given the various benefits antioxidants provide for the human body, it is important to explore novel methods of enhancing their absorption [144]. The application of nano-antioxidants is one of the new techniques that scientists can develop thanks to nanotechnology [145]. The potential uses of nano-antioxidants in medical treatments and food preservation have been investigated. By halting oxidation reactions that cause food products to spoil, nano-antioxidants can be used to increase their shelf life [146]. In medicine, they could potentially be used to treat diseases caused by oxidative stress such as cancer and heart disease. Furthermore, nano-antioxidants could be used as targeted drug delivery systems for therapeutic interventions inside the body [147]. In 2011, Sharpe et al. [148] proposed for the first time the nano-antioxidant term. These artificial antioxidants should be at the nanoscale level and with this feature they can penetrate cells more easily than conventional antioxidants. The chemical composition in addition to the surface and its physical properties such as charge types, surface-to-volume ratio, and the coating material of the nano-antioxidants are important physiochemical characteristics of nano-antioxidants [143].
Nano-antioxidants can be in many forms, for instance, lipid-coated nano-antioxidants, polymeric nano-antioxidants, metal oxide nano-antioxidants, dendrimers, quantum dots, and fullerenes [144]. A lipid-coated nano-antioxidant usually consists of an oil or fat-soluble antioxidant encased in a liposome. Polymer is generally made up of polyethylene glycol and can also include antioxidants [145]. Metal oxide nano-antioxidants, which can absorb ultraviolet radiation, are commonly titanium dioxide or zinc oxide [146]. Dendrimers are complex molecules with antioxidants on their surfaces, while quantum dots are semiconductor nanocrystals that fluoresce when illuminated [147]. Numerous techniques have been established for the synthesis of nano-antioxidants. The most common techniques applied for the preparation of nano-antioxidants are templating, SF, emulsion/solvent evaporation, and solvent displacement [134]. Nano-antioxidants’ tunable catalytic and redox properties, as well as their ability to oscillate between various oxidation states, are responsible for their ability to act as antioxidants [148].
Melanin, metal oxides, lignin, and carbon have all been used to make nano-antioxidants [149]. Until now nano-antioxidants made with different materials like metal, metal oxide, carbon nanotubes (CNTs), and polymers [150]. Recent studies showed that antioxidant encapsulation is the most effective way to increase their absorption because encapsulation controls their release and has low toxicity [151]. Several studies have shown that selenium nanoparticles (NPs), due to their small size and high surface-to-volume ratio, possess greater bioavailability than larger particles. This has been seen to activate selenoproteins in both in vitro and in vivo environments with antioxidant activity [152]. By reducing the generation of ROS and inflammation in rats, nano-selenium has been shown to mitigate the effects of nicotine-induced nephrotoxicity. Two antioxidant enzymes that gain from this increased bioavailability are glutathione peroxidase (GSH-Px) and thioredoxin reductase, which use selenium in selenoproteins for hemostasis regulation and redox system preservation [153].
For dose-dependent antioxidant activity zinc oxide NPs derived from Polygala tenuifolia root extracts have become a popular choice for medical applications due to their antibacterial and anticancer properties [154]. Moreover, plant-derived silver NPs have free radical scavenging activity, which is an effective tool in preventing oxidative stress-mediated toxicity. Because of their captivating electron affinities, single-walled CNTs in particular are thought to have remarkable antioxidant properties [155].
Because of their strong affinity with electrons and antioxidant properties, carbon-based NPs have been found to have extreme cleaning activity. Single-walled CNTs have been confirmed to be particularly effective in the oxygen radical absorbance capacity assay [156]. Additionally, other types of NPs, such as those coated with CNTs or metal/metal oxide particles, also have antioxidative properties [157]. Additionally, cerium oxide NPs (CONPs) have exhibited multi-enzymatic reactive nitrogen and oxygen species scavenging abilities and can be used to reduce lipoperoxidation [158]. Liposomes are also utilized in delivering antioxidants to target areas due to their biocompatibility; chitosan (CTS) is often included in this process in order to protect the molecules from harmful free radicals [159]. Finally, while pH and oxidative stress levels of inflamed tissues are adjusted, curcumin-based nanocarriers (Figure 1) can effectively encapsulate antioxidant molecules and regulate their release by lipopolysaccharide-stimulated macrophages [160].
A summary of some nano-antioxidants, highlighting their unique qualities, functionalization techniques, and important features are provided in Table 2. Nano-antioxidants have various uses in the field of nanotechnology, including food preservation and medicine. The connections between material science and biological applications by describing the engineering of each type of nano-antioxidant to enhance its antioxidant properties are demonstrated by Table 2.
Nano-antioxidant types, functionalization strategies, and notable features
Nano-antioxidant type | Antioxidants and functionalization strategy | Significant features | References |
---|---|---|---|
Silica (SiO2) nano-antioxidant | GA on SiO2 NPs | Precise control and GA functionalization for improved scavenging. | [161] |
Aurum (Au) NPs nano-antioxidant | Trolox, cellulose fiber immobilization | Therapeutic, improved antioxidant properties with immobilization or functionalization. | [162] |
Argentum (Ag) NPs nano-antioxidant | Green synthesis, bio-functionalization | Green synthesis, enhanced antioxidant activity. | [163] |
Hematite (Fe2O3) NPs nano-antioxidant | Surface coating, poly(GA) functionalization | Tailored strategies, improved antioxidant properties with surface functionalization. | [164] |
CONPs | CNPs with curcumin | Antioxidant enzyme-mimetic, enhanced anticancer properties with curcumin. | [165] |
Dual NPs | Quercetin, GA-mediated Ag-selenium (Se) bimetallic NPs | Enhanced antioxidant properties, and improved efficiency with dual-functionalized NPs. | [162] |
Polymeric nano-antioxidant | PCL, bacterial cellulose, silymarin, zein | Biodegradable nanocarriers, improved antioxidant activities in polymeric nanocomposites. | [162, 166] |
Application of natural antioxidants to prevent oxidation in foods
Antioxidants can be present in foods naturally also called “Endogenous antioxidants” (e.g., polyphenols in tea, catechins, quercetin, and anthocyanins in grape, rosmarinic acid, and carnosol in rosemary, beta-carotene in carrot, and etc.) or added as a form of naturally extracted or synthetic ones also called as “exogenous antioxidants” [167]. The incorporation of antioxidants in food offers multifaceted advantages, categorizable into two primary domains. Firstly, from a health perspective, natural antioxidants are instrumental in disease prevention and mitigating adverse cellular reactions. Secondly, they contribute significantly to maintaining the high quality of food products [168]. The discourse surrounding antioxidants extends to both natural and synthetic variants, with a plethora of studies highlighting the potential toxicological risks associated with synthetic antioxidants. This has precipitated a heightened emphasis on leveraging natural antioxidants within the food industry, underscoring their superior safety profile and efficacy [169].
The main purposes of the usage of antioxidants in foods are summarized below:
Preventing lipid oxidation.
Preventing cholesterol oxidation.
Preventing protein and DNA damage.
Reducing microbial growth.
Extending the shelf-life of the product.
There are different mechanisms that have been attributed to natural antioxidants’ capacity to achieve all the above-mentioned purposes, for example, reducing oxidative damage, free radical scavenging rate, and modulating the activity of superoxide dismutase (SOD) and glutathione peroxidase (GPX) in the biological cellular functions [169], as well as preventing lipid oxidation by scavenging chain-carrying peroxyl radicals/suppressing the formation of free radicals [170]. Since plants are abundant sources of natural antioxidants, animal-based products like meats, dairy, and egg products are required to be fortified with antioxidants. Further, it is essential to add antioxidants to oils obtained from plants due to their high potential for lipid oxidation. Moreover, synthetic antioxidants such as butylated hydroxyanisole (BHA) and butylated hydroxytoluene (BHT). Propyl gallate and tert-butylhydroquinone (TBHQ) are used widely in the poultry industry [171, 172].
The combination of natural and synthetic antioxidants can result in reducing oxidative stress. Moreover, they can cut the redox reaction chain associated with ROS. The formation of ROS is linked either to the suppression of the innate antioxidant defense systems or the overproduction of oxygen species. Both mechanisms are common, considering that oxygen is ubiquitous in the environment, especially in biological processes involving aerobic organisms. The visual illustration suggests that free radical scavenging ability is predicted by chemical structure and active components [173]. To understand the main effects of oxidation on the quality of foods, it is important to mention both lipid and cholesterol oxidation.
Lipid oxidation
Lipid oxidative stability is one of the main parameters in food quality and sensory evaluation during shelf life. Lipid oxidation can occur in almost most food products specially based on the lipid and oil content amounts [174]. For instance, in meat products lipid oxidation is known as a quality control and spoilage factor because of the susceptibility of meat products to oxidative degeneration [175]. Lipid oxidative processes are influenced by (1) the presence of polyunsaturated fatty acids; (2) iron; (3) pro-oxidants; (4) endogenous antioxidants (endogenous antioxidants include enzymes (e.g., SOD, catalase, GSH-Px, glutathione reductase, coenzyme Q10) and non-enzyme components, such as nutrient-based antioxidants (e.g., tocopherols and tocotrienols, carotenoids, and lipoic acid) and metal-binding proteins (e.g., albumin, ferritin, ceruloplasmin, and lactoferrin) [176]; (5) the action of oxygen; (6) the addition of salt; (7) mechanical processes; (8) different animal species and their muscle structure. For instance, there is a series between different animal species based on stability: beef > pork > chicken > turkey > fish. Also, within a species of poultry, the dark (thigh) meat is more susceptible to oxidation than the white (breast) meat [175, 177]. Oxidation in meat products can occur before cooking (raw meat products) and also after cooking (cooked meat products) which in both systems needs to be controlled [175].
The mechanism of lipid oxidation starts as free radicals on cellular metabolism and lipid peroxidation reactions commensurate with the substantial damage to cells. The membranes of cells are composed of unsaturated (double bonds) fatty acids, which are very sensitive to peroxidation reactions. In fact, docosahexaenoic and arachidonic acids are among the major substrates of peroxidation in the membrane. Membrane properties like fluidity and permeability would depend on its structure and stability. Therefore, as a result of lipid peroxidation structure and functions can be destroyed. In biological cells, proteins and DNA are also sensitive targets for ROS [178]. Chelation of transition metals is an effective manner by which to inhibit lipid oxidation in food emulsions. Proteins like casein are known to have iron-binding ability, and many studies have reported the participation of phosphoryl groups in iron binding. Antioxidants also have been reported to have an ability to chelation of metals which causes and promotes oxidation and damage [179]. Other ways to escape oxidations are listed below:
Escaping from in photo-oxidation, wavelengths that are nearly ultraviolet A (UVA, 340–400 nm) and visible light (400–700 nm), along with an appropriated sensitizer such as chlorophyll and riboflavin, together with triplet oxygen (3O2), lead to oxidative degradation of cholesterol through photodynamic reactions.
Preferring cold press than hot process during oil production.
The bioactive compounds present in plant extracts possess similar or even higher antioxidant effects.
Adding natural (natural antioxidants from spices and herbs) or synthetic antioxidants. Antioxidants prevent free radical-induced cell and biological target damage by preventing the formation of radicals, scavenging them, or promoting their decomposition [174, 180].
Cholesterol oxidation
Foods of animal origin such as beef, poultry, fish, and dairy products are vulnerable to oxidation due to their high content of unsaturated compounds, e.g., fatty acids, cholesterol, etc. Further, the oxidation of cholesterol-containing foods appears non-enzymatically yet auto-oxidative through complex chain reactions based on the formation of free radicals. Additionally, critical factors in these auto-oxidation yet complex degradation processes include room temperature, light, and the presence of oxygen. Essential antioxidant enzymes naturally produced are known as the primary antioxidants, qualified by the donation of electrons or hydrogen to the free radicals formed within the initiation and propagation phases of lipid oxidation. The mechanism is based on the conversion of free radicals into either thermodynamically stable products and/or lipid-antioxidant complexes. The cholesterol in the food matrix is another molecule that may oxidize during the process when exposed to heat, free radicals, and oxygen. More so, the formation of cholesterol oxidation products (COPs) requires to be controlled and reduced [180]. Natural extracts (NEs) often contain high concentrations of phenolic compounds that have either strong H+ donating activity or high radical-absorbance capacity. Major phenolic constituents are acids (gallic, caffeic, and rosmarinic acids), diterpenes (carnosol and carnosic acid), flavonoids (quercetin, catechin, apigenin, kaempferol, naringenin, and hesperetin), and volatile oils (eugenol, carvacrol, thymol, and menthol). Phenolic acids inhibit the formation of free radicals and inactivate radical species. Besides acting as metal chelators, there are both initial and propagation stages of oxidative processes [167, 168, 171].
Polyphenols present in cocoa do inhibit oxidation effects, which could help prevent vascular ailments like arteriosclerosis, cardiovascular disease, and myocardial infarction. There was research on the antioxidant properties of cocoa. Polyphenols in cocoa could treat model rabbits with high cholesterol wherein low-density lipoprotein (LDL) contents in the blood decreased significantly. Also, polyphenols in cocoa could help reduce arteriosclerosis in mice. Furthermore, the cholesterol/lipid oxidation [detected by thiobarbituric acid reactive substances (TBARS)] contents reduced oxidative stress in tissues significantly [167, 181]. Moreover, natural antioxidants such as anthocyanins as intelligent packaging indicators could permit easier monitoring of packaged products during transportation/storage. To reiterate this, the design of the active packaging (AP) and intelligent system is shown in Figure 2, revealing the various components like wrap plastic low-density polyethylene (LDPE) film, intelligent packaging sensor, etc. [175]. Notably, conventional polymers (polyester, cellulose, polyethylene, etc.) applied to encapsulated antioxidant molecules would benefit agricultural/food packaging applications [173]. The functionality of natural antioxidants which are immobilized in a cover matrix is basically a color changer and indicators like anthocyanins from saffron petals in CTS nanofibers and methylcellulose matrix [175]. By extending indicator functionality, natural antioxidants can help prevent the oxidation process and same time, monitor inside intelligent packaging films.
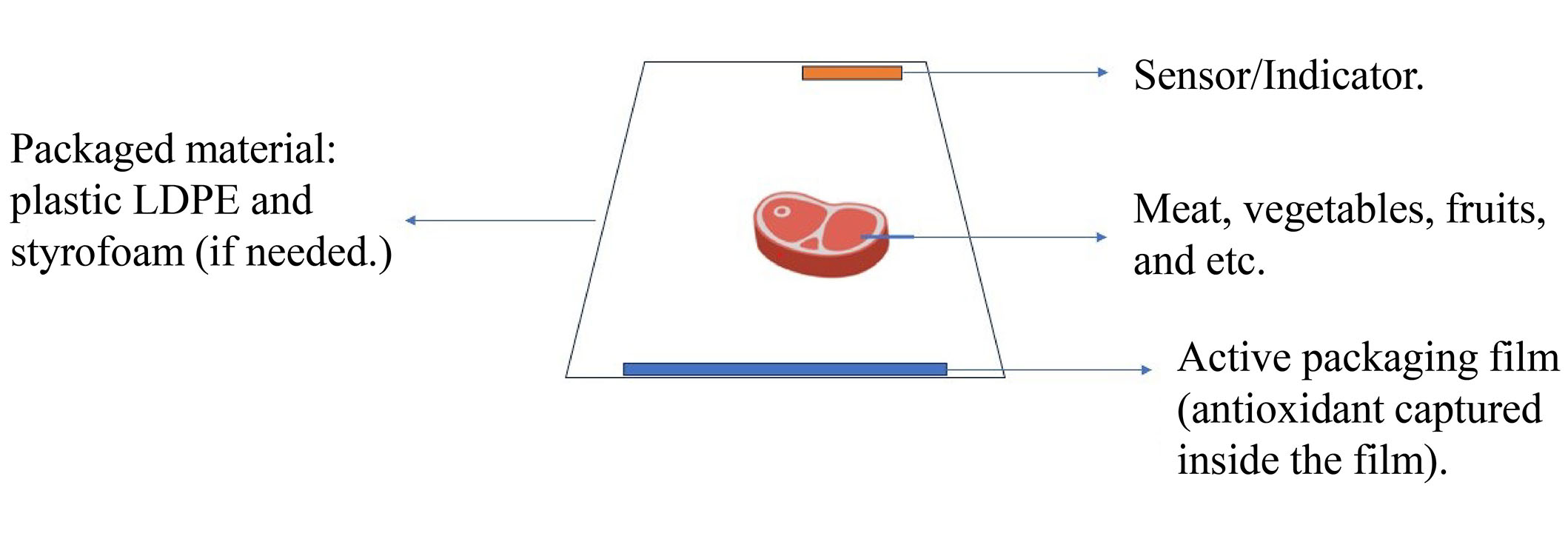
Design of the AP and intelligent system
Note. Adapted from “Application of an intelligent sensor and active packaging system based on the bacterial cellulose of Acetobacter xylinum to meat products,” by Dirpan A, Djalal M, Kamaruddin I. Sensors (Basel). 2022;22:544 (https://doi.org/10.3390/s22020544). CC BY.
Different types of antioxidant polymers
Polymeric antioxidants (synthetic or natural) are a low-weight molecular group obtained from enzymatic reactions and conjugated bounds with strong pharmacology application potential. Nowadays, polymeric antioxidants are utilized in various sectors of the food industry largely as (dietary) additives/supplements, which fortify and preserve food quality during transportation/storage. Further, polymeric antioxidants offer unparalleled capabilities in tumor-targeted drug delivery, biodegradation of polymers, and antimicrobial treatment. Polymeric antioxidants are able to affect some enzyme systems involved in the pathogenesis of some diseases [182, 183]. Besides natural antioxidant polymers like pro-anthocyanidins, some main polyphenolic components in the human diet include natural phenols (flavan-3-ols), which are available in many food types like cocoa, red wine, orange, different types of tea, onions, olives, broccoli, and all colorful berries [173]. By the formation of hydrophobic and hydrogen bonds with digestive enzymes, high molecular weight polyphenols could control and interact with lipase and amylase. Some polymeric antioxidants were found as chelating agents. These compounds are important in food processing because of their potential to stabilize food aroma and flavor and also color pigments by bonding with metal ions [182].
The growing environmental awareness continues to energize the transition to green/biodegradable materials. Therefore, different natural antioxidant polymers like lignin and polyphenol adduct polysaccharides would help develop green, biocompatible, and biodegradable antioxidant polymers useful in packaging, textiles, and agricultural sectors. Additionally, food wastes are known as the main sources of natural antioxidants. For example, poly-furfuryl alcohol and its derivatives can be directly isolated from agricultural wastes [173]. A brief classification of polymeric antioxidants and their properties are given below:
Poly-lactones and polymer NPs integrated with living polymerization techniques, poly(b-malic acid) derivatives. Also, edible flavors such as vanillin (phenolic aldehyde) can be polymerized into an antioxidant co-polymer known as poly(vanillyl alcohol-co-oxalate) (PVAX) which is utilized in biomedical applications. Vitamin C and other poly vitamins with potent antioxidant properties can synthesized by the acyclic diene metathesis (ADMET) polymerization method. Moreover, certain natural essential oils (EOs) such as limonene can be polymerized into renewable poly-limonene while preserving their antioxidant properties [173].
Phenol and substituted phenols containing monomers: phenol, ethyl phenol, catechol, p-ethyl phenol, o-chlorophenol, pyrogallic acid, p-cresol, and so others [183]. Polymeric phenols are a group of polymers consisting of synthesized large phenols by enzyme catalysis or other chemical reactions. Indeed, they can be obtained naturally from different fruits and vegetables. Polymerization can affect antioxidant potential activity and antioxidant application. For instance, pro-anthocyanins have a higher antioxidant activity compared to natural antioxidants such as vitamins C and E. Dopamine and quercetin when polymerized can encapsulate cancer treatment medicines. Catechin-acetaldehyde poly-condensates could also control the lipid peroxides in human (post-exercise) plasma and prevent ailments such as atherosclerosis, gastric ulcers, and bowel cancer [182].
Flavonoids monomers: (+)-catechin hydrate, rutin, hesperidin, epigallocatechin gallate, (–)-epicatechin, quercetin, resveratrol, GA, daidzein, formononetin, (–)-catechin, taxifolin, kaempferol, and arbutin and generate [171], just like phenolic components, flavonoids can be synthesized and formed antioxidant polymers or can be present naturally in foods.
Natural antioxidant polymers such as lignin, inulin, dopamine, melanoidins, etc. Lignin is a natural polymer and biomacromolecule that supports the connective tissues in plants. Lignin-based compounds can be utilized for polymerization of dopamine, inulin, quercetin (poly-quercetin and quercetin copolymers), and terpene polymers, which effects final antioxidant properties [173].
Continued progress of antioxidant-active food packaging
It is important to reiterate that oxidation may decrease the quality of various food components [184]. In fact, the oxidization of proteins would make, for example, meat products, become tougher and less juicy [185, 186]. When sulfur-containing amino acids are irradiated, oxidation reactions occurring in meat products could show an off-flavor [187]. Following microbial proliferation, the primary cause of (food) spoilage would be lipid oxidation particularly susceptible to the deterioration of foods high in lipids/unsaturated fats. The development of off-flavors, typical of rancidity, caused by the lipid oxidation in foods leads to an unpleasant product [188]. Meanwhile, decreasing lipid oxidation entails adding antioxidants to foods or designing a packaging technology that works well. Direct addition of antioxidant compounds on food surface may consume active compounds (ACs) in the reaction, negate its protection and rapidly decrease its quality.
The merging of high-barrier packaging components with vacuum or modified-atmosphere food packaging appears to be another promising methodology [189–191]. Even though it is technically difficult to eliminate all oxygen, this process can diminish the amount of oxygen in the package [192]. Antioxidants can be added to polymeric packaging films by either physically or chemically adding them. The produced films have also been strongly indicated for possible use in antioxidant-active food packaging to varying degrees, despite the fact that each of these procedures has its highlights and challenges. Active food packaging is a relatively new concept. Antioxidant AP systems are currently under development. In order to increase the stability of food products that are susceptible to oxidation, this innovative alternative packaging technology is predicated on the inclusion of antioxidant agents within the package [193–196]. There are different designs for AP inside a food package, which can include: (A) utilizing the active packs (oxygen and moisture absorbers, and ET vapor maker); (B) coating an AC onto the polymer (heat-sensitive active agents or those that are incompatible and immiscible with the polymer matrix); (C) immobilizing the active ingredient on the surface of the polymer (the two components must both have functional interacting groups). ACs are able to slowly diffuse into food because of the strong bonds they form with polymers; (D) direct inclusion into the polymer matrix, which ensures high resistance to processing conditions of the polymer, no adverse effect on the polymer properties, and slow release to food; (E) the use of bioactive polymers in coatings or composites, like CTS with inherent antimicrobial properties [197, 198].
The fundamental idea of controlled-release packaging (CRP) is to extend the shelf life of food by slowing down its deterioration by enclosing it in a package that contains a different concentration of an active ingredient. The package functions as a carrier in CRP systems, enabling the AC to be mixed into the formulation, held in reserve, and released when needed. The AC is only kept in the package for a short time. Consequently, the AC’s arduous paths are stifled or retained within the composition or by a barrier layer or coating. CRP is another generation of utilitarian bundling, going about as a conveyance framework for the AC delivery (antimicrobials, cell reinforcements, flavors, aromas, and plant development chemicals, for example, 1-methylcyclopropene, to influence natural product maturing; beginning or delaying) in a controlled manner to enhance safety. Even in antimicrobial and antioxidant packaging, the term “retaining quality” was used to describe a wide range of food products during storage. However, the terms are different [199, 200]. Moreover, CRP has been used extensively and successfully in the pharmaceutical, drug and food industries. The application of BHT as an antioxidant to increase the shelf life of breakfast cereal is already applied to market products [201]. However, the use of BHT is still up for debate [202].
Three types of antioxidants remain actioned in active food packaging: (a) plant extracts; (b) filtered compounds from regular sources; and (c) synthetic materials. EOs and plant extracts have continued to emerge as the primary alternatives to synthetic additives [203–206]. Phenolics, terpenoids, and aromatic compounds make up the majority of EOs and NEs. However, the part of the plant (such as the flower, fruits, leaves, seeds, stems, and others), the plant’s edaphoclimatic properties, and the extraction method can all significantly alter their composition [207, 208]. However, the use of EOs comes with a significant disadvantage in that the majority of them have a strong odor. Because of this, it is crucial to detect the available EO concentration without affecting the organoleptic characteristics of the meat [209]. The viability of active films appears to depict either rosemary as an EO or extract as a natural antioxidant. Barbosa-Pereira et al. [210] generated an LDPE film in which rosemary extract (3–20%) was consolidated by coating procedure. Then, beef samples were packed with this film in headspace/modified atmosphere packaging (MAP) and in direct contact, and the film’s effect on storage was observed. In this instance, the amount of extract used to generate the film inhibited lipid oxidation more, and it was also greater in samples that were in direct contact with the active film than in samples where antioxidants were released into the headspace.
One more regular natural antioxidant utilized in the development of films comprises extracts obtained from various assortments of tea. Nisa et al. [211] generated a film containing 5% green tea as an antioxidant and potato starch as a matrix biopolymer and kept in close contact with fresh beef. Sesamol-based CRP, which contains volatile antioxidants, is also impressive. For instance, in the case of oat cereals’ lipid oxidation [212]. When compared to nonvolatile compounds, volatile compounds are more effective at slowing microbial growth and oxidation because of their mobility. The deficiencies are reduced by protecting the volatile compounds with an encapsulation and barrier layer. One more necessity is the similarity of the packaging material with the AC. Antioxidants’ loading and release from the packaging material are both affected by this [213, 214]. Purified small molecular compounds found in fruits and vegetables, in addition to plant extracts, have been utilized in the creation of antioxidant packaging films. One model is ascorbic acid, which is a characteristic compound found in vegetables and citrus organic products. With or without Moringa oleifera Lam. leaf extract, the films containing ascorbic acid successfully slowed the browning of pear samples [215]. Although most of the active ingredients used in food packaging films for cell reinforcement originate from natural sources, animal-derived antioxidants have also been accepted. Melanin, a natural color found in most living things’ skin, eyes, hair, and brains, is a true model [216, 217]. Eumelanin and pheomelanin are components of natural melanin. Both come from the dopaquinone precursor, which is made by enzymatically oxidizing tyrosine. As of late, melanin NPs have been utilized in filmmaking for the age of a cell reinforcement food packaging film [218]. Some examples of antioxidant applications in active food packaging are shown in Table 3.
Antioxidant applications in active food packaging
Antioxidants | Polymer matrix | Food sample | Effects of antioxidant on food packaging | Reference |
---|---|---|---|---|
Citric acid | Corn starch | Vacuum-packed ground beef | The films showed higher biodegradability, reduction of the total bacterial count (TBC), and a beneficial effect on the color of the meat; maintenance of the cherry-red color of meat. | [219] |
Mango leaf extract | Polyethylene teraphthalate (PET) + polypropylene (PP) | Fruits and vegetables | High phenolics load, antioxidant capacity. | [220] |
Banana peel extract | CTS | Apple | Improving the postharvest quality of apple fruit. | [221] |
Moringa oleifera Lam. leaf extract | Fish skin gelatin film | Gouda cheese | Inhibited the microbial growth and retarded the lipid oxidation of cheese. | [222] |
Bamboo leaf antioxidant | Konjac glucomannan (KGM)/CTS/citric acid composite film | Food simulants | Significantly improved the oxidation resistance, anti-UV-visible, and mechanical properties of the materials. | [223] |
Fresh blood orange (citrus sinensis) peel extract | Gelatin | Food simulants | Modified the mechanical and thermal properties of gelatin films, higher antioxidant properties. | [224] |
Rice straw extract | Starch | Food simulants | Improved the oxygen barrier properties without negatively affecting the thermal and the water vapor barrier properties. | [225] |
Purple-fleshed sweet potato extract | CTS | Food simulants | Increase the thickness, water solubility, UV-visible light barrier property, and thermal stability of CTS film. Scavenge free radicals in dose- and time-dependent manners. | [226] |
Pineapple peel extract | Polyvinil alcohol/Corn starch | Food simulants | Increase thickness and water vapor permeability, thermal stability as the degradation occurred above 300°C, and the antioxidant properties. | [227] |
Orange peel extract | Gum arabic/Cr2O3 NPs | Food simulants | Significant increase in water vapor permeability, weight loss, tensile strength, Young’s modulus, thermal stability, the antimicrobial properties. | [228] |
Conclusions
This paper has attempted to demonstrate the recent advances in some nano-related natural antioxidants, their extraction methods and contributions to the food industry. Besides understanding the various extraction methods of by-products employed to recover antioxidants, this study has highlighted how nano-delivery systems and nano-antioxidants function, as well as how the application of natural antioxidants could prevent oxidation in foods. It was relevant to mention the different types of antioxidant polymers, as well as the continued progress of antioxidant-active food packaging. Nowadays, the consumption of antioxidants remains crucial in the daily diet of many. Indeed, different extraction methods of natural antioxidants are still avail such as UAE, MAE, PEF, PLE, HVED, EAE, SFE, SWE, and HHP, as highlighted in this current review.
Nano-antioxidants are used in the food and medicine industries. Also, both natural and synthetic antioxidants decrease lipid oxidation. Natural antioxidants should be consumed more often in the diet to avert oxidative stress. The accretion interest of consumers in utilizing natural ingredients in food products has brought about an acceleration in appeal for functional capacity acquired application “natural” operations. Undoubtedly, natural antioxidants will continue to occupy an increasing role in daily living across communities globally. The direction of future literature reviews should critically assemble the product development progress, challenges, and pathways of nano-related natural antioxidants, especially the evolvement over time as this would help reveal the various thriving milestones. Further reviews especially at the level of meta-analysis, together with new experimental works, would help reveal more novel data that would demonstrate the impact and potential application prospects of using nanotechnology for natural antioxidants. Indeed, the utilization of nanotechnology would have high promise to enhance the absorption of antioxidants in human metabolic systems.
Abbreviations
ACs: | active compounds |
AP: | active packaging |
BHT: | butylated hydroxytoluene |
CNTs: | carbon nanotubes |
CRP: | controlled-release packaging |
CTS: | chitosan |
EAE: | enzyme-assisted extraction |
Eos: | essential oils |
ET: | ethanol |
GA: | gallic acid |
GAE: | gallic acid equivalents |
HHP: | high hydrostatic pressure |
HPP: | high-pressure processing |
HVED: | high voltage electrical discharges |
LDPE: | low density polyethylene |
MAE: | microwave-assisted extraction |
NPs: | nanoparticles |
PEF: | pulse electric field |
PLE: | pressurized liquid extraction |
ROS: | reactive oxygen species |
SF: | supercritical fluid |
SFE: | supercritical fluid extraction |
SWE: | subcritical water extraction |
UAE: | ultrasound-assisted extraction |
Declarations
Author contributions
AEK, AK, and SZ: Conceptualization, Investigation, Writing—original draft. CORO: Writing—review & editing.
Conflicts of interest
The authors declare that they have no conflicts of interest.
Ethical approval
Not applicable.
Consent to participate
Not applicable.
Consent to publication
Not applicable.
Availability of data and materials
Not applicable.
Funding
Not applicable.
Copyright
© The Author(s) 2024.