Abstract
Treatment of sepsis currently relies on eliminating the causal pathogen and supportive care, whereas almost no approaches to interfere with the defining event of a “dysregulated host response” are available. This review points to the striking correlation of two phenotypes of sepsis etiopathology with the concept of bipartite response patterns of higher organisms to microbial attacks. According to this concept, the phenotypes of sepsis can be interpreted as either resistance or tolerance responses to infection that got out of hand. This concept might allow focusing sepsis research and related patient studies on key conundrums of current sepsis research: how do resistance responses result in immunopathology and how can tolerance lead to systemic immunosuppression or even immunoparalysis? The heuristic vigor of these questions might inspire experimental efforts and clinical studies and ultimately advance the therapeutic armamentarium for sepsis care.
Keywords
Sepsis, immunopathology, immunosuppression, resistance, toleranceIntroduction
Different, partially contrasting patient symptoms represent a main trait of sepsis. Arriving in the intensive care unit, sepsis patients frequently exhibit a hyperinflammatory phenotype. Afterwards, significant immune suppression becomes apparent. In some patients, both phenotypes occur in parallel and are intimately interwoven.
Other divergent phenotypic characteristics are well documented, e.g., in the recent Medical Education for Sepsis Source Control and Antibiotics (MEDUSA) study, a randomized trial aimed to improve early sepsis diagnosis and treatment [1]. During the study period, clinical characteristics of more than 6,000 patients with severe sepsis including septic shock from 40 hospitals were documented. Notably, body temperatures showed a bimodal distribution pattern with a frequency peak at about 35.5°C and a bigger peak around 38.5°C [2]. As an overarching result, the study reveals that the majority of sepsis patients exhibit either hypothermia or fever (Figure 1), reminiscent of the Roman God Janus expressing two opposing faces.
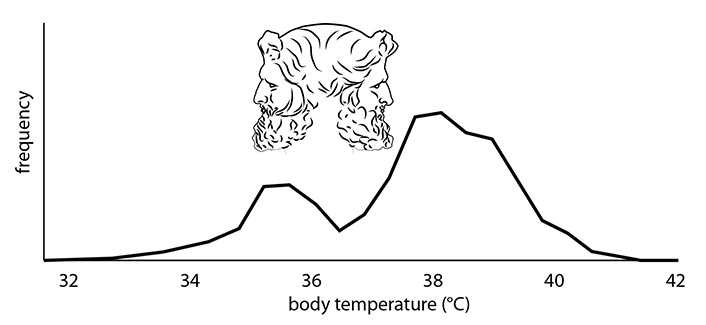
Frequency distribution of body temperature (°C) in sepsis patients
Note. Adapted from “Fever and hypothermia represent two populations of sepsis patients and are associated with outside temperature,” by Thomas-Rüddel DO, Hoffmann P, Schwarzkopf D, Scheer C, Bach F, Komann M, et al. Crit Care. 2021;25:368 (https://ccforum.biomedcentral.com/articles/10.1186/s13054-021-03776-2). CC BY.
Recent investigations support the idea of convergent immunological responses and thermal responses in severe infections [3, 4]. Both animal experiments [3] and patient studies [5] appear consistent with a link of fever to hyperinflammatory resistance responses and of hypothermia to immunosuppression. The balance between immune reactions and thermoregulation seems to be affected by energy trade-offs induced by infectious stress [3]. Based on these findings, two predominant phenotypes of sepsis patients might be postulated: one exhibiting fever and hyperinflammation, and another with hypothermia often accompanied by immune suppression. The bimodal temperature responses might be interpreted as indicators for differential reactions of the immune system.
Fitting to the aim of this special issue immediately, two obvious queries become apparent:
—How do these seemingly opposing phenotypes evolve?
—How are these differential patterns of sepsis interconnected?
To elaborate on these important questions, we initially suggest exploring the relationship of both postulated sepsis phenotypes to the regular response patterns of mammalian organisms to infection.
Infection-induced resistance and tolerance
Attacks by microorganisms are a daily routine during the lifetime of higher organisms. To prevent pathological effects of infectious stress, three basic strategies evolved: avoidance, resistance and tolerance [6, 7]. Whereas avoidance aims to hinder the penetration of pathogenic microbes into the organism, resistance responses attempt to detect and destroy intruding pathogens locally at the place of invasion. As spearheads of the defense machinery macrophages and granulocytes phagocytose invade microbes and kill them intracellularly by releasing a toxic cocktail of proteases and reactive oxygen species [8]. This potentially dangerous process is strictly controlled by cytokines, chemokines and other mediators. Hence, these cellular and humoral components of the immune system act as main players of resistance responses against invading microbes.
In contrast to resistance, tolerance responses by definition do not change the number of invading microbes but prevent damage to the affected tissue [6, 7]. To this effect, tolerance might deactivate toxic substances produced by microbes or activate maintenance and repair processes in the affected tissue. These regenerative deeds of tolerance responses specifically include controlled degradation of damaged cells and subcellular compartments and their subsequent renewal.
Importantly, resistance and tolerance responses act complementarily. Both response patterns to infection are spatially and temporally interwoven, with shifts of the balance in both directions supposedly in dependence on the energy status of vital cells involved. The actual balance of specific resistance and tolerance responses decides about the predominating (‘net’) phenotype (Figure 2).
As illustrated in Figure 2, the course of these specific organismic responses to infectious attacks is influenced by the invading pathogen species, by the entryway of the pathogens and most notably by the pathogen dose [9]. Whereas energy demanding resistance responses predominate at low pathogen load (immune cells on the left balance tray) at high pathogen load (microbes at the right balance tray), cells and organs shift to immunosuppressive responses.
Immunopathology and immunosuppression in sepsis
During sepsis, infection-induced immune responses are out of control [10–12]. Whereas resistance and tolerance during uncomplicated infectious attacks efficiently cooperate, these complementary immune reactions lose their adaptive potential and tissue damage emerges. The aberrant immune answer evades the local restrictions to the entrance site of the invading microbes, and molecular and cellular immune responses are becoming systemic and negatively affect the fitness of the host (Figure 3).
In sepsis, resistance mechanisms normally aimed to destroy pathogens become misdirected impairing cells and organs of the infected organism [9]. Hence, hyperactive immune cells release their antimicrobial weapons comprising reactive oxygen species and proteolytic enzymes and so damage cells of the surrounding parenchyma [11]. In addition, immune cells liberate large amounts of cytokines and chemokines, which might induce hyperinflammatory responses in the vascular system and contribute to remote organ failure [12]. During sepsis, humoral and cellular components of the immune system lose their adjustability and partially develop pathological self-destructive reaction patterns. In this regard, the tissue destructive impact of reactive oxygen species and proteases released by hyperactive immune cells appears of special importance.
The rampant resistance responses causing immunopathology in sepsis seem to show certain parallels to the transition between physiological tolerance responses and septic immunosuppression. Tolerance responses to infections are normally characterized by a coordinated interplay of catabolic and anabolic processes, which allows continuous regeneration of tissues affected by pathogens. In contrast, during immunosuppression, catabolic reactions predominate hindering the repair of tissue injury [13]. Regeneration of immune cells is restrained ultimately provoking lymphopenia. As a consequence, pathogen burden is significantly increasing leading finally to increased mortality [14].
Understanding the turn from resistance responses to immunopathology and comprehension of the conversion of tolerance to immunosuppression represents an outstanding challenge for sepsis research. To tackle this central conundrum of sepsis, exploration of related signaling processes and metabolic interrelations seems of central importance (Figure 3). Improved knowledge of these discrete routes of sepsis pathogenesis will predictably allow first insights into the interconnections of immunopathology and immunosuppression as the next level of the multifaceted disease progression.
Infection-induced signaling processes and metabolic adjustment
The molecular control of effective and failing organismic responses to microbial attacks is a matter of intensive research. These research efforts are aimed to find novel concepts for pharmacological treatment of immunopathological processes during infectious diseases. Promising targets for pharmacological therapy of these diseases are malfunctioning signaling mediators controlling the immune response towards invading microbes.
Effective responses of mammalian organisms to infectious attacks are tightly controlled by specific signaling mediators and pathways. The current knowledge on signaling events mediating resistance and tolerance responses to infections, emerges from investigations in cellular and animal models. Numerous studies reveal the crucial role of energy sensing signaling pathways in the control of adaptive responses to infectious stress [3, 4, 10, 15, 16].
Specifically, in vitro and in vivo data indicate the key role of the mechanistic target of rapamycin (mTOR) signaling pathway in the induction of resistance responses to infectious microbes [17]. mTOR is known as a central mediator of anabolic processes in a similar manner controlling energy demanding cell functions of immune cells such as proliferation or cytokine release [18]. The involvement of the signaling protein mTOR in immune responses to microbial infection becomes apparent by the strong immunosuppressive effects of the macrocylic compound rapamycin, which is the eponym inhibitor of mTOR signaling activities.
Signaling reactions that trigger the development of tolerance to infection are less well understood. Recent investigations reveal the signaling protein adenosine monophosphate (AMP)-activated protein kinase (AMPK) as a critical mediator of tolerance responses [19]. In contrast to mTOR, AMPK activity is induced at low cellular energy levels, which emerges at low intracellular concentrations of ATP and concomitant high concentrations of its hydrolysis product AMP. In pathogen-challenged immune and parenchymal cells, the signaling protein AMPK controls maintenance and repair reactions, including autophagy of cellular organelles [19, 20]. A key role of AMPK has been proposed for tissue damage control in parenchymal cells [19].
Together infection induced resistance and tolerance responses appear to be part of evolutionary conserved and interdependent reaction patterns of the organism to environmental stress (Figure 4). Whereas mTOR acts as a central mediator of resistance responses, the signaling protein AMPK predominates in control of critical maintenance and repair processes during tolerance to infection. The balance of complementary resistance and tolerance responses is strongly affected by the energy status of the cells involved. At the high level of ATP, resistance responses against invading microbes predominate, whereas at low ATP level and concomitant high AMP cells turn to tolerance.
How do the central response patterns of mTOR and AMPK change in sepsis? Experimental evidence indicates central roles of both signaling proteins in the etiopathology of the syndrome. Whereas mTOR has been shown to contribute to the development of sepsis-related immunopathology, AMPK seems to be involved in immunosuppression [17, 19, 20]. Understanding of malfunctions of related signaling pathways represents a major challenge for sepsis research.
A conceptual framework for the interplay of immunopathology- and immunosuppression phenotypes in sepsis provides the “metabolic reprogramming” observed during sepsis progression [21, 22]. mTOR-driven anabolic processes accompanying infection-induced hyperinflammation and immunopathology lead to increasing exhaustion of energy resources and finally turn to immunosuppression. In striking difference to the effective cooperation of resistance and tolerance responses during common infections, sepsis immunosuppression frequently exhibits irreversible traits and does not facilitate homeostasis. Hypothetically, this phenotype might be stabilized by epigenetic alterations, controlled by histone acetylases, histone deacetylases and other enzymes controlling gene transcription. As such, the immunosuppressed phenotype shares similar energy conserving traits comparable to hibernation states in mammalian organisms like bears or marmots during winter season [23].
Experimentally, sepsis-induced hyperinflammation and immunopathology have been effectively treated by drugs targeting mTOR. In septic mice, the mTOR inhibitor rapamycin significantly suppressed the hyperinflammatory response and decreased mortality [24]. Interestingly, the AMPK agonist metformin induces similar responses fitting to the proposed inhibitory effects of AMPK on mTOR controlled processes [25].
Hence understanding of the signaling pathways mediating failing immune responses in sepsis opens new prospects for sepsis treatment.
Relevance of the two phenotypes for diagnosis and therapy
During the past decades, the sepsis paradigm shifted from a systemic inflammatory response syndrome (SIRS) to an overarching disruption of immune balance, which includes a significant portion of patients exhibiting signs of immunosuppression. The paradigm shift is best reflected in current trials studying the potential of immunostimulatory therapies, such as check point inhibitors as adjunct options in sepsis treatment [13].
First “proof-of-concept” studies would be consistent with a partial reversion of immunosuppression by treatment with interferon γ (IFNγ) in vivo [15] (Figure 5). Immunostimulatory agents used in rodent sepsis models cover a broad spectrum of additional agents, including pathogenic yeast Candida albicans, its cell wall component-glucan [26] and also checkpoint inhibitors [27]. However, these approaches urgently need advanced patient stratification.
In line with the critical role of mTOR in resistance responses described above, hyperinflammation and immunopathology during sepsis can be restrained from a cell biology point of view by the immunosuppressive and antiproliferative agent rapamycin. Rapamycin has e.g., been shown to protect against acute lung injury in a mouse model of sepsis [24]. The current interest in repurposing of anti-inflammatory drugs derived from rheumatological diseases for coronavirus disease (COVID) associated hyperinflammation associated lung injury is also in line with this concept [28, 29]. Exemplarily a randomized clinical trial by Kyriazopoulou et al. [28] evaluated the efficacy and safety of anakinra, a recombinant interleukin-1 (IL-1) receptor antagonist that blocks the activity of the proinflammatory cytokines IL-1α and IL-1β. Anakinra as an established medication for the treatment of rheumatoid arthritis in the given clinical study has been employed for patients with COVID-19-related respiratory failure. In comparison to patients treated with placebo the anakinra group indeed exhibited a significant decrease in Sequential Organ Failure Assessment (SOFA) score, a decrease of twenty-eight-day mortality and a considerably shorter hospital stay. These data convincingly disclose the effectiveness of the concept of targeted inhibition of key signaling mediators of hyperinflammatory responses in sepsis, i.e. organ dysfunction due to infection.
Additional approaches to treat the hyperinflammatory phenotype of sepsis have been revealed in a recent experimental study, where the authors screened a drug library for candidates that affect inflammatory reactions in a macrophage cell line [30]. Anthracyclines—commonly applied for the therapy of solid cancers—were identified to prevent organ dysfunction in mouse models of polymicrobial sepsis. Apparently, low doses of anthracyclines induce DNA damage response and autophagy, which are assumed to be the mechanisms underlying tissue protection and anti-inflammatory effects. Interestingly, irradiation produced similar effects [30]. Very recent investigations also indicate reductions in inflammatory responses and decreased mortality of hospitalized patients with COVID-19-related pneumonia by treatment with low dose radiation [31].
Together, these data indicate that low doses of stressors, such as toxins or irradiation, stimulate cellular repair and thereby induce cross protection of the affected tissue. The anti-inflammatory effects of anthracyclines or radiation might be connected to the energy demands of these treatment approaches. Organismic responses to environmental stressors and microbial attacks compete for energy sources [3]. Specifically, protein synthesis and immune cell proliferation might be suppressed by stress induced by toxins or radiation [30]. Accordingly, treatment of cells with anthracylines or radiation seems to lower the immune response in favor of maintenance and repair reactions.
In fact, these novel approaches for treating hyperinflammatory processes might productively add to the ongoing efforts to apply steroids for the adjunctive modulation of the host response leading to sepsis. Large clinical trials testing hydrocortisone therapy in septic shock have produced conflicting results. Whereas a recent French study reported outcome benefit from a combination of hydrocortisone plus oral fludrocortisone [32], the pan-European Corticosteroid Therapy of Septic Shock (CORTICUS) trial and the 5-country ADRENAL trial found no survival effect from hydrocortisone alone [33, 34]. Subgroups may however benefit depending on their individual immune response suggesting strong dependence of the treatment attempts on patient stratification [35].
The given novel approaches for treating sepsis-related immunopathology or immunosuppression are likewise relevant for differential diagnosis of the disease. Hence diagnostic efforts might include signaling activities of key intracellular mediators of immunopathology and immunosuppression like mTOR and AMPK. In addition, metabolic parameters may be assayed in more detail. Considering the initially postulated relation of body temperature to the two sepsis phenotypes assays of patients’ temperature profiles get particular importance in sepsis diagnostics.
In spite of the inherent complementarity of resistance and tolerance responses to infection, the definition of two distinct phenotypes in sepsis progression appears fruitful for the development of novel treatment approaches. Together, these innovative interventions targeting both immunopathology and immunosuppression of sepsis might add to the therapeutic armamentarium in the infection-driven organ dysfunction, which discriminates sepsis from uncomplicated infection although significant hurdles regarding coexistence of both phenomena, e.g., in a cell- and tissue-specific manner remain to be overcome.
Conclusions
The present review emphasizes two seemingly opposing phenotypes of sepsis: immunopathology and immunosuppression. The proposed definition of immunopathology as out-of-control resistance response to infection and vice versa immunosuppression as unbalanced disease tolerance phenomenon allows to link the knowledge on (infectious) stress responses to sepsis research. As a feasible focus for future sepsis research, we suggest the investigation of the transition from physiological resistance responses to immunopathology and from tolerance to infection to immunosuppression. Related innovative experiments and clinical studies will probably advance therapeutic armamentariums to treat sepsis.
Abbreviations
AMP: | adenosine monophosphate |
AMPK: | adenosine monophosphate-activated protein kinase |
COVID: | coronavirus disease |
IL-1: | interleukin-1 |
mTOR: | mechanistic target of rapamycin |
Declarations
Acknowledgments
The authors are especially grateful to Margit Leitner for designing the figures.
Author contributions
Both authors have contributed to the conceptualization, writing and revising of this review. Both authors read and approved the final manuscript.
Conflicts of interest
The authors declare that they have no conflicts of interest.
Ethical approval
Not applicable.
Consent to participate
Not applicable.
Consent to publication
Not applicable.
Availability of data and materials
Not applicable.
Funding
MB is funded by the Deutsche Forschungsgemeinschaft (DFG, German Research Foundation) under Germany′s Excellence Strategy—EXC 2051—Project-ID 390713860. The funders had no role in study design, data collection and analysis, decision to publish, or preparation of the manuscript.
Copyright
© The Author(s) 2022.