Abstract
Sepsis is a life-threatening condition caused by dysregulated host immune response to infection, leading to persistent inflammation followed by immunosuppression. Sepsis represents a substantial global health problem owing to protracted inflammation, immune suppression, and susceptibility to nosocomial infections. Despite continuing progress in the development of antibiotics, fluid resuscitation, and other supportive care therapies, no specific immunomodulatory drugs or immunotherapeutic adjuncts for the treatment of sepsis are available to date. The advances in tertiary care facilities and patient care have improved the survival of sepsis patients in the initial hyper-inflammatory phase of sepsis. However, the majority of sepsis patients succumb later due to prolong immunosuppression. The sepsis-induced immune dysregulation and its long-term effects on mortality are under meticulous investigations that are still poorly defined. Sepsis leads to the impaired functions of the innate and adaptive immune systems. The exhaustion of T cells, reduced expression of human leukocytes antigen (HLA)-DR on monocytes, and induced uncontrolled apoptosis of immune cells have been reported as hallmark features of sepsis. Sepsis-induced immune cell apoptosis of immune cells is a primary contributing factor to the immunosuppression in sepsis. Preclinical studies have identified several new therapeutic targets for therapy in sepsis, including monoclonal antibodies (Abs) and anti-apoptotic agents to reduce T cells exhaustion, immune cells apoptosis, and restoring immune cells functions. Recent studies have centered on immune-modulatory therapy. The review article will focus solely on sepsis’ effects on innate and adaptive cells functions that contribute to immunosuppression. Finally, it is discussed how immune cells responsible for immunosuppression might be directly targeted to provide potential therapeutic benefits in treating sepsis and improving long-term survival.
Keywords
Sepsis, immunosuppression, innate and adaptive immunity, multiple organ failureIntroduction
Sepsis is a leading cause of mortality and morbidity across the globe [1]. It is a systemic inflammatory response induced by trauma, burns, and infection. Bacterial infections are a major cause of sepsis [2]. When sepsis is not recognized early and treated promptly, it can lead to septic shock, multiple organ failure (MOF), and death [3]. Those patients who have recovered from sepsis are not free from danger; only half will completely recover, and the rest will die within one year of recovery or be burdened by long-term disabilities [2]. About 30% of diagnosed patients with sepsis die yearly in the United States [4]. In 2017, about 49 million cases of sepsis were recorded worldwide, and 11 million people succumbed due to sepsis; many of them were children [4]. Sepsis represents a significant cause of maternal and childhood mortality and morbidity in low- and middle-income countries [5]. New-born babies, pregnant women, and people living in low-resource settings are more vulnerable to sepsis-associated mortality and morbidity [6]. Despite the advancement in the tertiary care facilities, early resuscitation, surgical interventions, ventilator management, and antibiotic therapy, sepsis-associated mortality remains the leading cause of death in the intensive care unit (ICU) [7].
Antimicrobial resistance is a major challenge in sepsis treatment for clinicians [8]. The escalating cost of treatment for sepsis patients in the ICU is alarming. More than 100 therapeutic clinical trials have been conducted in the murine model of sepsis, and the few are under the different phases of the trial; no Food and Drug Administration (FDA)-approved treatment options currently exist for sepsis [9]. Recent advancements in understanding immune pathophysiology have resulted in improved survival due to sepsis, but long-term sepsis-associated mortality rates remain at 20% to 50%. Several studies have proposed that impaired cellular metabolism, tissue oxygenation, immune dysfunctions, and coagulopathy are plausible causes of sepsis-associated mortalities and morbidity in long-term settings [2, 3, 10]. However, impaired immune dysfunction in sepsis is a most accepted postulate associated with persistent inflammation, immunosuppression, and long-lasting immune disturbance [11]. The defective immune homeostasis during sepsis allows recurrent infections with commensal and invading pathogens, leading to the MOF and death [10]. The sepsis-induced immune dysregulation and its long-term effects on mortality are under meticulous investigations that are still poorly defined.
The initial injuries that occur due to trauma or burn lead to the production of inflammatory cytokines by systemic inflammatory response syndrome (SIRS). In order to over the inflammatory response, anti-inflammatory cytokines secretions get started by the process of compensatory anti-inflammatory response syndrome (CARS) and mixed antagonist response syndrome (MARS) [12]. The induced inflammatory and anti-inflammatory responses due to trauma, injuries, or burns are protective for the host to prevent invading pathogens. When the balance of these counter-regulatory processes is maintained, immune homeostasis will be readily restored [13, 14]. However, induced exaggerated inflammatory or anti-inflammatory responses due to trauma or burns are deleterious to the host defense system [15]. When the process is significantly dysregulated; the consequence may lead to sepsis and sepsis-associated complications in the ICU. Sepsis patients are unable to maintain the immune homeostasis due to long-lasting immune disturbance, immunosuppression, and persistent inflammation. The majority of sepsis patients are died due to defective immune homeostasis [15]. The reason for sepsis-induced dysregulated immune responses and defective immune homeostasis is under meticulous investigation that is not yet defined. The review article will focus solely on sepsis effects on innate and adaptive cells functions that contribute to immunosuppression. Finally, we discussed how altered immune cells functions lead to immunosuppression might be directly targeted to provide potential therapeutic benefits in treating sepsis and improving long-term survival. How sepsis leads to the defective functions of immune system and subsequently causes the MOF is shown in Figure 1.
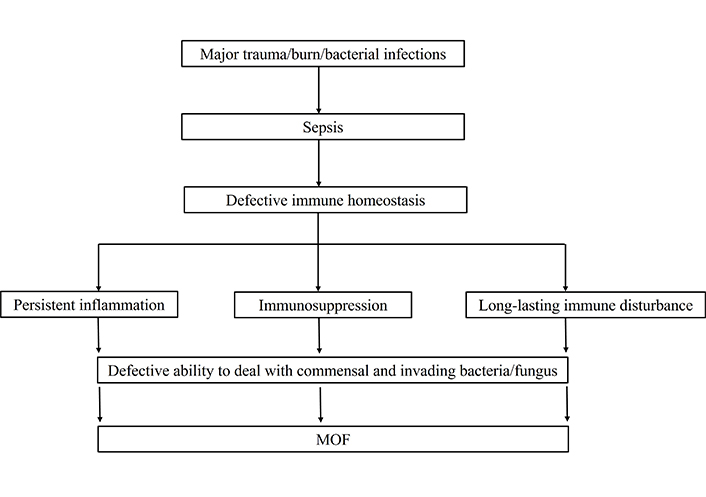
How sepsis leads to the defective functions of immune system and subsequently causing the MOF
Immune suppressive players of sepsis
Sepsis adversely affects both arms of immunity, innate, and adaptive immunity [16]. Innate immunity is also called inborn immunity, which protects the host against a plethora of pathogens non-specifically. The innate response includes the complement system, dendritic cells (DCs), innate lymphoid cells, and highly interactive responses, including white blood cells (neutrophils, monocytes, and macrophages). The major players of innate and adaptive immunity whose functions are primarily altered during sepsis are shown in Figure 2.
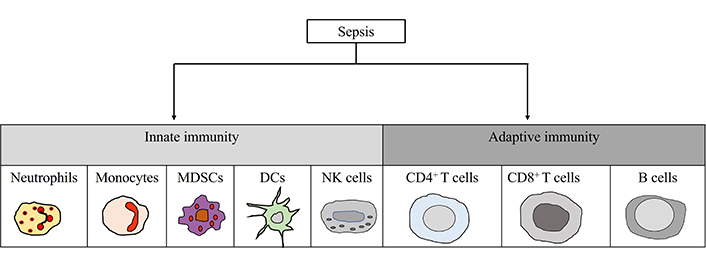
The major immunosuppressive players of innate and adaptive immune system during sepsis. MDSCs: myeloid-derived suppressor cells; NK: natural killer
The innate immune system relies on germ-line encoded diverse cell surface receptors and secreted proteins to recognize pathogen-associated molecular patterns (PAMPs), as well as proteins, lipids, RNA, and DNA structures associated with a microbial infection [17]. The invading pathogen encounter first with host innate immune system. The innate immune cells sense pathogens by recognizing conserved PAMPs through an array of pattern recognition receptors (PRRs) and they get activated [18]. PRRs are expressed on a variety of host cells, including cells of epithelial, myeloid, and endothelial origin. Major classes of PRRs include toll-like receptors (TLRs), nucleotide-binding oligomerization domain (NOD)-linked receptor (NLRs), C-type lectin receptors (CLRs), retinoic acid-inducible gene-1 (RIG-1)-like receptors (RLRs), and receptor for advanced glycation end (RAGE) products [18]. Unlike PAMPs, damaged associated molecular patterns (DAMPs) and alarmins are produced by injured tissues and organs, such as histone, mitochondrial DNA and nucleosides, high mobility group box-1 (HMGB-1) protein, ATP, adenosine, and protein S100 A. Recognition of PAMPs or DAMPs by host PRRs leads to the activation of innate immune cells through a complex set of signaling events that induce a host-protective response. The induced-cellular response against PAMPs or DAMPs has protective functions such as prevention or elimination of host stress from microbial infection or tissues damage, activates complement cascade or phagocytosis, and induces local inflammation [19]. All these cellular events have an important role in preventing the systemic dissemination of microbial infection during its early phages while the adaptive immune response is being developed.
Neutrophils
Neutrophils represent 50% to 70% of circulating leukocytes and are a fundamental component of innate immunity. It is essentially required for protection against microbes and is the first responder to foreign invaders [20]. Once a neutrophil recognizes a pathogen, it gets activated and phagocytoses the pathogen and produces reactive oxygen species (ROS) in a controlled fashion within the phagolysosome. Neutrophils also kill pathogens and eradicate them by non-oxidative mechanisms by secreting granules containing digestive proteases and perforins [21]. Neutrophils also recognized the pathogens and eliminated them by forming neutrophil extracellular traps (NETs). In sepsis, the maturation and functions of neutrophils are severely affected, leading to immune dysfunction and inflammation persistence [22]. Sepsis-induced, a state of delayed maturation of neutrophils, increased secretion of immunosuppressive mediators, reduced production of ROS, and impaired NETs formation that is associated with defective oxidative burst and microbial eradication [23]. The immune-suppressive functions of neutrophils during sepsis are shown in Table 1. Most of the patients who died from sepsis have pronounced derangement in neutrophils functions [24]. Several studies suggest that defects in neutrophil-mediated microbial containment and eradication could serve as therapeutic targets to improve the maturation, differentiation, and function of neutrophils.
Role of innate and adaptive arms of immunosuppressive players in sepsis
Cells | Normal functions | Immunosuppressive functions |
---|---|---|
Players of innate immunity | ||
Neutrophils | Neutrophils are potent phagocytic cells showing the antimicrobial activity through oxidative killing and granule product-mediated killing |
|
Monocytes | Monocytes are the source of other vital elements of the immune system, such as DCs and macrophages. Monocytes recognize the pathogens through the PRRs and phagocyte them. They work as APCs, secrete cytokines and chemokines, and activate Th cells |
|
MDSCs | MDSCs are a heterogeneous population of cells of myeloid origin. They are differentiated into granulocytes, macrophages, and DCs. MDSCs are potent suppressors of T cells proliferation and activation. They are primarily beneficial for restoring homeostasis after inflammation. Because of their ability to suppress adaptive immunity, MDSCs can also ameliorate autoimmune diseases and semi-allogenic responses |
|
DCs | DCs are professional APCs and play important roles in regulating the innate and adaptive immune responses |
|
NK cells | NK cells are effector lymphocytes having cytotoxicity and cytokines secreting effector functions. NK cells control several types of microbial infections and tumors by limiting their spread and subsequent tissue damages |
|
Players of adaptive immunity | ||
CD4+ Th cells | Th cells are major components of adaptive immunity, recognize the pathogens through class II MHC, and activate the B cells to secrete Abs and macrophages to destroy the ingested microbes. They also activate the cytotoxic CD8+ T cells during inflammation and help in recruiting the PMNs, basophils, and eosinophils to the loci of infection and inflammation |
|
CD8+ Tc cells | Cytotoxic CD8+ T cells kill the infected and cancerous cells much like the NK cells do. They recognize the antigens, processed and presented them through class I MHC molecule, and get activated. The activated CD8 T cells secrete perforins and granzymes, and eliminate the intracellular pathogens and cancerous cells |
|
B cells | B cells fight against extracellular pathogens by secreting too specific Abs against antigen. B cells also perform the role of APCs and activate the Th cells in clearing the infected cells and cancerous cells |
|
Abs: antibodies; APCs: antigen-presenting cells; HLA: human leukocytes antigen; IL-10: interleukin-10; MHC: major histocompatibility complex; PMNs: polymorphonuclear neutrophils; Tc: cytotoxic T; Th: T helper; Treg: T regulatory
Monocytes and macrophages
The impact of sepsis on monocyte maturation, differentiation, and functions has been the subject of intensive investigations. The immunosuppressive functions of monocytes that occurred during sepsis are highlighted in Table 1. The reduced mononuclear cell HLA-DR expression and diminished capacity of monocytes to secrete pro-inflammatory cytokines in response to TLRs agonists are hallmark properties of sepsis [25]. Paradigm shifts in the secretion of pro-inflammatory cytokines to anti-inflammatory cytokines are significantly associated with the severity of sepsis, nosocomial infections, and poor outcomes [26]. A recent study shows the disparity in expression and function of monocytes subsets during sepsis [27]. These findings support the concept of monocytes reprogramming in sepsis. The mechanism responsible for endotoxin tolerances, diminished expression of HLA-DR, excessive production of immunosuppressive mediators, and decreased antigen presentation by monocytes during sepsis is not fully understood [25, 28]. Recent studies highlighted that epigenetic reprogramming in monocytes during sepsis is an epicentre of monocyte anergy [29]. However, the functional impact of these epigenetic alternations is not yet known.
MDSCs
MDSCs are a heterogeneous population of cells of myeloid origin that are differentiated into granulocytes, macrophages, and DCs [30]. Several studies conducted in the murine model of sepsis and humans showed a significantly elevated level of MDSCs in sepsis. The immunosuppressive functions of MDSCs during sepsis are shown in Table 1. In sepsis, these cells have been shown to block T cells proliferation and function [31]. These cells suppress the host adaptive immune response by inhibiting the production of IL-2 and interferon-γ (IFN-γ) by T cells. Due to the lack of universally accepted phenotypic markers, it is difficult to investigate the genesis of MDSCs and their functions in disease [32]. The role of MDSCs in the pathogenesis of sepsis and its association with disease severity are not fully understood.
DCs
DCs are professional APCs and are essentially required to regulate both arms of immunity: adaptive and innate immunity [33]. DCs recognize the exogenous antigens, process them, and present them to helper T cells for the activation and proliferation of T cells. DCs are required to recognize the pathogens and eradicate them through cell-mediated immunity [34]. In sepsis, the immunosuppressive functions of DCs are associated with increased sepsis-induced apoptosis of DCs and reduced surface expression of HLA-DR on DCs [35]. DCs secrete immune suppressive cytokines (IL-10) and help in expanding the Treg cells during sepsis [36]. All the immunosuppressive functions of DCs occurred in sepsis are shown in Table 1. Recent studies showed that preventing sepsis-induced DCs apoptosis or augmentation of DC function in the murine model of sepsis enhances sepsis survival [34]. The immune-suppressive function of DC has been ameliorated by treating models of sepsis with growth factor FMS-like tyrosine kinases three ligands (FLT3L). Treatment with FLT3L in models of burn sepsis improved DCs cytokine secretions and functions of T cells [37]. These observations suggested that improvement in DCs number and functions may be primary targets for therapeutic innervations in sepsis.
NK cells
NK cells work as a rudimentary killer of cells infected by the viruses or lacked self-identifications [38]. NK cells are effector lymphocytes having cytotoxicity and cytokines secreting effector functions [39]. They protect the host from several microbial infections and tumors by limiting their spread and subsequent tissue damages. The functions of NK cells have not been fully understood in the consent of human disease due to their preferential location in tissues and low numbers in peripheral blood [40]. In sepsis, the levels of NK cells and their effector functions are markedly decreased. Like monocytes, NK cells become tolerant to TLR agonists in sepsis [41]. Impaired productions of cytokines in response to TLR agonist and cytotoxic functions have been reported in polymicrobial sepsis [42]. The molecular mechanism leading to the impaired cytotoxic function of NK cells, diminished productions of cytokines, and enhanced apoptosis of NK cells during sepsis are under meticulous investigation.
Generally, the innate immune cells recognize the invading pathogens and eliminate them, but sometimes the pathogen prevails, and the host response can become unbalanced and deleterious. The adaptive immune response is crucial for effective protection of the host against specific pathogenic microorganisms. Unlike the innate immune response, the adaptive immune response recognizes the pathogens too specifically, precisely differentiates self- and non-self-antigens, generates immunological memory against pathogens, and takes time to trigger the host immune response [43]. Ideally, the adaptive immune response recognizes and eliminates the infectious agent rapidly during re-infections with the same pathogen. The cross-talk between cell-mediated immune response (T-cells mediated) and humoral immune response (B cells mediated) immune response is required for effective protection of the host against specific pathogens [44].
CD4+ Th cell
About 15–30% of total leukocytes are T cells in human peripheral blood. The proportion of CD4+ Th cells and CD8+ cytotoxic T cells of total T cells accounts for 60–65% and 35–40%, respectively [45]. Th cells are a vital component of adaptive immunity which recognizes the pathogen through class II MHC and activates the B cells to secrete Abs and macrophages to destroy the ingested microbes [46]. The activation of CD4+ T cells results in polarisation into Th cells subsets Th1, Th2, Th17, and Treg cells. The difference among the subsets of Th cells is the cytokines and transcription factors that induce their differentiation and the cytokines they produce [47]. Th1 subset is essential for activating CD8+ T cells and for the formation of memory T cells via IL-2 secretion. The Th1-mediated cellular immunity is crucial to recognize the intracellular infection of a virus or bacteria and eliminate them by inducing phagocytosis or complement activation [48]. Th2 cells secrete IL-4, IL-5, and IL-13 cytokines upon activation and mediate class switching of B lymphocytes [49]. The Th2 cells mediated immunity is critical to protect against parasitic infections. Th17 cells are effector cells that secrete the cytokines IL-17 and IL-22 primarily in response to extracellular fungal and bacterial pathogens [50]. Treg cells are an essential subset of helper T cells that regulate the activated immune response once the pathogen is eradicated. Treg cells produce immunosuppressive cytokines [IL-10 and transforming growth factor (TGF)-β] and are essential in preventing autoimmunity [51].
The sepsis-induced apoptosis of CD4+ Th cells is the most notable cause of defective adaptive immunity. The presence of a significantly higher level of caspase 8 and caspase 9 in T cells of sepsis patients indicates that apoptosis occurs by intrinsic and extrinsic pathways [52]. Further, overexpression of pro-apoptotic proteins on T cells in sepsis patients was confirmed by several studies [53]. The apoptosis of T cells and increased expression of pro-apoptotic proteins contribute to defective adaptive immunity and immune suppression in sepsis. T-cell exhaustion and shift in polarity of Th cells from Th1 to Th2 are other significant causes of immune suppression in sepsis [54]. Marked reductions in the transcription factors and cytokines required for the polarisation and differentiation of Th cells subsets support the notion that CD4+ functions are suppressed during sepsis [50, 55]. The sepsis-induced immune suppression is relegated to Th1 and Th2 subsets and Th17, Treg cells, and other helper T cell subsets. The diminished cytokines secretion by Th17 cells has been reported in sepsis [56]. The level of Treg cells was reported significantly higher in sepsis patients as compared to non-sepsis patients. Further, levels of Treg cells were remained elevated in those patients who died due to sepsis. A recent study reported that the increased Treg cells and their ratio with Th17 (Treg/Th17) are associated with sequential organ failure (SOFA) score in post-traumatic sepsis patients [54]. Treg-associated immune dysfunctions and poor outcomes among trauma patients have been shown by several studies [57]. Recent research studies show that DCs secrete immune suppressive cytokines (IL-10 and TGF-β) and help in expanding the Treg cells during sepsis [36]. In an animal model of sepsis, it was shown that the adenosine was largely responsible to enhance the expression of forkhead box protein P3 (FoxP3, a major transcription factor of Treg differentiation) and leads to immunosuppression [58]. Treg-associated defective immune functions in sepsis have also been linked to increased solid tumor growth, probably from diminished T cells mediated effector functions and mononuclear cells effects [59]. Therefore, the notion exists that sepsis induces elevation of Treg cells that dysregulates the functions of the immune cells, contributes to poor outcomes, and may serve as the primary targets for immune modulation.
Although Treg cells can contribute to immune suppression in sepsis, Treg depletion in the murine models of sepsis does not improve mortality rates. In fact, the findings of one study in mice deficient in Treg cells showed that animals could not fight the initial sepsis infection [60]. Therefore, it is clear that a subtle balance of Treg cells is required to maintain immune homeostasis in sepsis.
CD8+ cytotoxic T cells
CD8+ T cells are also called killer T cells or cytotoxic T cells that express CD8 glycoprotein on the cell membrane as their T cell receptor (TCR). The primary function of CD8+ T cells is to kill the virus-infected cells and tumor cells through cell lysis by degranulation or apoptosis [61]. CD8+ T cells recognize the antigenic peptide of pathogens through MHC class I and get activated. Upon activation, CD8+ T cells secrete cytokines, primarily tumor necrosis factor (TNF)-α and IFN-γ, and release cytotoxic granules to mediate the killing of virus-infected cells and tumor cells [62]. Several studies have confirmed the sepsis-induced apoptosis of CD8+ T cells [63]. The expression of caspases and pro-apoptotic proteins has been reported higher in CD8+ T cells of sepsis patients, especially in non-survivors compared to non-sepsis patients. The diminished production of cytokines and cytotoxic granules is the most notable cause of immune cell dysfunctions during sepsis.
γδ T cells
γδ T cells relatively represent a deficient proportion of T cells. It is a distinct subset of T cells primarily present in the intestine and mucosal surface where they recognize the pathogens and mount an innate-like immune response by releasing cytokines such as IFN-γ, IL-17, and chemokines. γδ T cells exhibit innate and adaptive immunity features and play a pivotal role in host defense against invading pathogens, immune surveillance, and homeostasis [64]. The circulating level of γδ T cells was reduced in patients with sepsis. The reduced level of γδ T cells in sepsis patients is associated with sepsis severity and mortality rate [65, 66]. This is probably due to the apoptosis-induced loss of γδ T cells in the intestinal mucosa. The reduced level of γδ T cells and diminished production of cytokines by γδ T cells are primarily associated with immune defects during sepsis [65]. These findings show that preventing γδ T cells apoptosis and restoring the effector functions of γδ T cells could attenuate inflammatory responses during sepsis and prevent poor outcomes.
B cells
Like T cells, B cells also play an essential role in inducing innate and adaptive immune responses [67]. B cells could be differentiated into Abs secreting plasma cells and long-lasting memory cells upon activation. B cells also work as APCs and activate the effector T cells [68]. Several studies have reported the reduced circulating B cells and their function during sepsis [69]. Reduced production of Abs by B cells and the development of memory plasma B cells are associated with the severity of sepsis and mortality rate [70]. The relative proportion of B cells is reduced in sepsis patients, indicating that the apoptosis of B cells was induced. Depleting memory B cells contribute to the suppressed immune state experienced by septic patients. These observations confirm B cell dysfunction in sepsis.
Immune modulators and immunotherapies in sepsis
Sepsis with subsequent MOF remains the leading cause of mortality in the ICU. Sepsis-induced apoptosis of immune cells and dysregulated immune system are pivotal to immunosuppression [15]. Immune suppression is primarily associated with disease severity and high mortality rates in sepsis. Despite the high mortality rate due to sepsis and sepsis-associated complications, no specific treatment modality or drugs are available to date [14]. Preclinical and clinical studies reveal that sepsis-induced immunosuppression is reversible. Several therapeutic adjuncts and immunostimulatory treatments are already available, but limited studies have evaluated the efficacy of these drugs and their impact on the reversal of immune suppression [11]. Below, we discuss the most promising immunotherapeutic agents that target the innate and adaptive immune systems.
Cytokine therapy
IL-7
The role of pro-inflammatory cytokines as a potential to reverse sepsis-induced immunosuppression has been evaluated in preclinical and clinical studies. IL-7 has emerged as a promising therapeutic agent because it mediates T cell and B cell function [71]. Data from animal models of sepsis have approved the use of recombinant IL-7 in sepsis clinical trials. Ex vivo stimulation of lymphocytes obtained from septic patients with recombinant IL-7 induces lymphocytic proliferation and increases the expression of anti-apoptotic proteins [71]. A recent study conducted in humans showed that administration of recombinant IL-7 increased lymphocytes numbers, restored T cells functions, and restored the delayed-type hypersensitivity response to recall antigens in sepsis [72]. IL-7 improves multiple functional aspects of T cells, including diversification of TCR repertoire, reducing the proportion of Treg cells, decreasing programmed cell death-1 (PD-1) expression, and increasing the expression of cell adhesions molecules, thereby facilitating the trafficking of T cells to sites of infection [73].
IFN-γ
IFN-γ is also called lymphokines because lymphocytes primarily secrete it. IFN-γ is a key cytokine that induces innate immunity by enhancing antigen presentation and microbial killing [74]. The immunological defect in sepsis is associated with decreased production of IFN-γ [75]. Several small clinical trials have investigated the effect of exogenous IFN-γ administration on innate immunity functions [76]. Impaired functions of monocytes have been restored after administration of recombinant IFN-γ in mice model of sepsis. Ex vivo stimulation of monocytes obtained from septic patients with recombinant IFN-γ restored the expression of HLA-DR on monocytes and reduced amounts of TNF-α production [77]. Similarly, immunological restoration of monocytes function has been observed in a small cohort of sepsis patients by monocytes ex vivo stimulated with lipopolysaccharides (LPS) [78]. A recent study reported that adjunctive treatment with recombinant IFN-γ restored innate immune function in patients with fungal sepsis [79]. A recent trial conducted in patients with human immunodeficiency virus (HIV) who had cryptococcal meningitis treated with IFN-γ showed enhanced clearing of fungal infections from cerebrospinal fluid [80]. Although IFN-γ restored the impaired functions of monocytes in sepsis, it cannot correct the fundamental defect of T cells, a primary pathological abnormality in sepsis.
Granulocyte-macrophage colony stimulation factor and granulocyte-colony stimulating factor
The hallmark of sepsis is neutropenia and diminished functions of neutrophils. Granulocyte-macrophage colony stimulation factor (GM-CSF) and granulocyte-colony stimulating factor (G-CSF) were shown to decrease the incidence of sepsis in patients with neutropenia [81]. The immunostimulatory effects of GM-CSF are mainly associated with the enhancement of phagocytosis and cell survival. Administration of GM-CSF in patients with sepsis restored ex vivo production of TNF-α that resulted in fewer nosocomial infections [82]. A meta-analysis of GM-CSF and G-CSF clinical trials conducted in sepsis did not show a positive outcome [81]. Hence, GM-CSF and G-CSF as the adjunct therapy in sepsis are currently not supported.
Checkpoint inhibitors
PD-1 is upregulated on T cells in sepsis and cancer; it is often associated with T cells exhaustion, which results from prolonged exposure to antigens [83]. T cells exhaustion has been associated with immunosuppression during sepsis. The prolonged duration of sepsis and disease severity are often associated with high antigen loads and increased levels of anti-inflammatory cytokines [10]. A potential link between T cells exhaustion and upregulation of PD-1 on T cells is associated with sepsis’ morbidity and mortality [84]. Animal studies have shown that blockage of PD-1 improved survival in several clinical studies and restored T cells exhaustion in sepsis [85]. PD-1 ligand and PD ligand 1 (PD-L1) are expressed on monocytes, macrophages, DCs, epithelial cells, and endothelial cells. Anti-PD-1 and anti-PD-L1 treatments have shown positive responses and improved cancer survival in small clinical trials [83]. These data suggest that checkpoint inhibitors can be a promising treatment for sepsis. A recent study conducted in the model of sepsis that T cells obtained and treated with anti-PD-1 or anti-PD-L1 decreased apoptosis of T cells and enhanced T-cell function [86]. Altogether, these preclinical and clinical studies of several small trials of checkpoints inhibitors demonstrate significant restoration of T cells exhaustion. We speculate that anti-PD-1 and anti-PD-L1 might be advantageous in activating T cells immunity and reducing the apoptosis of T cells in sepsis. Cytotoxic T-lymphocyte antigen-4 (CTLA-4) is also known as CD152. CTLA-4 is one of the critical inhibitory regulators of early T cell activation and proliferation stages. CTLA-4 is a member of the immunoglobulin superfamily, which is expressed on the surface of T cells and transmits an inhibitory signal to CD4 and CD8 T cells. The inhibitory signal sent by CTLA-4 reduces T cells activation and proliferation [87]. Several small clinical trials were conducted in the animal models of cancer to block the inhibitory signal of CTLA-4 by Abs. Dose-dependent response of anti-CTLA-4 Ab therapy was reported in cancer; at the low dose, it improved the survival from cancer, but at high dose worsened the outcomes [88]. A recent study reported the positive response of anti-CTLA-4 therapy to the model of sepsis [85]. It reduced the sepsis-induced apoptosis of lymphocytes and restored T cells effector functions. However, anti-CTLA-4 therapy has a positive response in several small sepsis clinical trials but is not extensively investigated. Hence, further evaluation of checkpoints inhibitors in a large sepsis cohort will be required to assess the relative contribution of immune cell subsets to improved patient prognosis.
Conclusions
Supportive care is the standard of care for patients with sepsis. Despite its curative intent, most patients with sepsis died in the ICU due to MOFs. Early recognition of sepsis patients and providing them appropriate supportive care are paramount until novel drugs or inventions are established to be effective. The present understanding of the immune response to sepsis is controversial. Some studies postulate that sepsis-induced organ failure results from persistent inflammation due to hyperactivation of the immune system. In contrast, other studies proclaim that it results from long-term immunosuppression. We support the idea that sepsis-induced immunosuppression with impaired monocyte function and T cells exhaustion is a notable anomaly. As discussed in the review, sepsis induces multiple defects in immunity that cause persistent inflammation, immune suppression, susceptibility to nosocomial infections, and death. Therefore, it is vital to identify when patients have developed a sepsis-induced immunosuppressive phase. Detecting particular defects in immunity will enable the application of immunomodulatory drugs or therapeutic adjuncts that have shown great promise in animal models of sepsis and preclinical studies. Cytokine therapies and checkpoints inhibitors have promising results in reversing immune defects in sepsis that subsequently lead to improved survival in small clinical studies. Considering the organized and complex immune dysregulation and the prolonged immunosuppression in sepsis, we believe that single-agent immune-modulatory intervention is not enough to target sepsis. All the past trials that attempted in sepsis with a single immune-modulatory target were probably failed. Therefore, immunotherapy targeting multiple aspects of immunity would be the next significant advance in treating sepsis.
Abbreviations
Abs: | antibodies |
APCs: | antigen-presenting cells |
CTLA-4: | cytotoxic T-lymphocyte antigen-4 |
DAMPs: | damaged associated molecular patterns |
DCs: | dendritic cells |
G-CSF: | granulocyte-colony stimulating factor |
GM-CSF: | granulocyte-macrophage colony stimulation factor |
HLA: | human leukocytes antigen |
ICU: | intensive care unit |
IFN-γ: | interferon γ |
IL-10: | interleukin-10 |
MDSCs: | myeloid-derived suppressor cells |
MHC: | major histocompatibility complex |
MOF: | multiple organ failure |
NETs: | neutrophil extracellular traps |
NK: | natural killer |
PAMPs: | pathogen-associated molecular patterns |
PD-1: | programmed cell death-1 |
PD-L1: | programmed cell death ligand 1 |
PRRs: | pattern recognition receptors |
ROS: | reactive oxygen species |
Th: | T helper |
TLRs: | toll-like receptors |
TNF: | tumor necrosis factor |
Treg: | T regulatory |
Declarations
Author contributions
DLG wrote the manuscript, designed the study, and conceptualised the study. TS helped in conceptualising the study and did the referencing. RP wrote the section of manuscript and helped in preparing the figures of manuscript. SB and DNR contributed to correcting the grammatical mistakes, read and approved the manuscript for the submission.
Conflicts of interest
The authors declare that they have no conflicts of interest.
Ethical approval
Not applicable.
Consent to participate
Not applicable.
Consent to publication
Not applicable.
Availability of data and materials
Not applicable.
Funding
Not applicable.
Copyright
© The Author(s) 2022.