Abstract
Hemangioblastoma are benign, vascularized cranial tumors caused by autosomal dominant inherited von Hippel-Lindau disease or can appear sporadically. This review will investigate current and emerging treatments for cerebral tumors. It will focus on the current and, more importantly, developing hemangioblastoma treatments. Surgical resectioning and radiotherapy are effective treatment options for cerebral tumors, whereas chemotherapies are not commonly used due to their limited ability to penetrate the blood-brain barrier. Recent chemotherapies have shown promise, but further research is needed to determine the efficacy as a treatment for hemangioblastomas. New advances in brachytherapy and immunotherapy are considered promising treatment options for hemangioblastoma. This review aims to offer valuable insights into the latest developments in hemangioblastoma treatments.
Keywords
Hemangioblastoma, cerebral tumor, von Hippel-Lindau, chemotherapy, radiotherapy, brachytherapy, immunotherapyIntroduction
Hemangioblastomas (HBs) are considered one of the rarest tumors that can occur, comprising only 1–2.5% of all intracranial tumors and accounting for 3% of all central nervous system (CNS) tumors and 7–8% of posterior cranial fossa tumors [1–4]. HBs are benign, highly vascular, and are mainly found in the cerebellum, followed by the spinal cord, brain stem, and retina [1, 2, 5, 6]. Cystic HBs are found more frequently in the cerebellar hemispheres, unlike the solid tumors found mainly in the brainstem and spinal cord [4]. Cystic HBs may develop sporadically or due to von Hippel-Lindau (VHL) disease, accounting for approximately 25% of all patients [4]. Retinal HBs are clinical symptoms of VHL, with a frequency of about 25% to 60% [7, 8]. Patients suffering from these cerebellar tumors experience prominent headaches, nausea, elevated intracranial pressure or cerebellar ataxia, and, in some cases, hearing loss [9]. HBs are more frequently diagnosed in males than females and tend to occur more frequently in patients between the ages of 30–60 but can occur in younger age groups with VHL [9, 10]. Studies have also suggested an association between higher birth weight and a higher risk of brain and CNS tumors in children [11].
The most reliable treatment method for these tumors is total resection, which ensures the best long-term survival outcomes [2, 5]. Even though there has been increasing evidence of stereotactic radiosurgery (SRS) as an effective treatment option for HBs, microsurgical resection is still the standard approach for most cases [4]. More specifically, the suboccipital keyhole approach for cerebellar HBs is safer and more effective in reducing complications and tissue damage than traditional surgical methods [5]. Historically, surgical removal was associated with unfavorable clinical outcomes, resulting in high morbidity and mortality rates of up to 50% for solid HBs in the posterior fossa [5]. Despite recent advancements in micro neurosurgical techniques, cerebellar HBs pose specific surgical challenges due to factors like the posterior fossa’s deep surgical corridor, difficulty debulking the tumor mass due to substantial vasculature, difficulty gaining early access to the feeding artery behind the tumor, and interference from large, tortuous draining veins during the resection [12]. Recently, there has been some success with targeting vascular endothelial growth factor (VEGF) using an antiangiogenic agent, bevacizumab, in patients suffering from multilocular HBs [13].
Although the underlying mechanism of HBs is unknown, it is often linked to VHL disease, an inherited multisystem disorder resulting from a mutation in the VHL gene [14]. Mutations in this gene are thought to initiate tumorigenesis by upregulating hypoxia-inducible factor (HIF) [15]. Efforts for developing new treatments targeting the pathobiological mechanisms underlying HB growth and proliferation may hold promise for achieving better outcomes with less invasive patient interventions.
Pre-clinical literature concerning HBs and cerebral tumors
Single-gene inherited disorders are the main risk factors for brain and CNS tumors [11]. Autosomal dominant disorders that can lead to a higher risk of brain and CNS tumors include VHL disease, Li-Fraumeni syndrome, neurofibromatosis type 1 and type 2, and Turcot syndrome [16].
Common brain tumor symptoms include headache, altered mental status, ataxia, nausea-vomiting, and muscle weakness [17]. These symptoms can present differently depending on the tumor’s location. HBs can appear pathologically different in various patients. For HBs found in the supratentorial region, the most common symptoms in patients include headache, visual disturbances, and endocrine changes [18]. When examining VHL patients, visual disturbances and endocrine changes were the most common symptoms [18]. The most common symptoms for HBs found in the brainstem were headache and/or dizziness, paresthesia, and pyramidal signs [19].
Juxta-papillary HBs are associated with the optic disc and can present as a fullness in the inner retina. Extra-papillary HBs, located elsewhere in the retina, can appear as a red or gray dot resembling a microaneurysm in the retina [7]. If left untreated, these lesions can lead to vision loss [7]. Though most HBs occur sporadically, around 25% of diagnosed HB cases are associated with VHL [20]. Patients with VHL tend to develop HBs at a younger age compared to those who do not have the disease [20]. One study found that patients with VHL had hemangioblasts expressing brachyury, a protein expressed during early development, suggesting that they may have a predisposition for HBs from embryologic development [21]. Patients with VHL also have a higher chance of tumor recurrence than non-diseased patients [22].
Solid tumors, which can spread through the body by displacing growth and metastasis, are more common across age groups. Aggressive cerebral tumors may invade surrounding tissues through infiltrative growth. Additionally, slower growth in cerebral tumors has been thought to grow by a displacement process called capillary fingering [23]. The immune system responds to these growths in various ways. For instance, patients with HB have higher levels of circulating endothelial progenitors that help to re-establish blood flow, which decreases upon tumor removal. In response to cerebral tumors, the immune system activates the heat shock response, characterized by an overproduction of heat shock proteins and chaperones. High levels of these proteins in tumor cells have been shown to lower tumorigenicity [24].
Some of the leading imaging methods for HBs include computed tomography, magnetic resonance imaging (MRI), magnetic resonance angiography, and contrast-enhanced ultrasound. Emerging ways to treat and understand HBs include µ-Doppler-imaging. An advantage of µ-Doppler ultrasound imaging is its ability to image without bolus contrast and its high sensitivity to vasculature flow. It has additionally been used to identify vascular and physiological anatomy details with better precision [25].
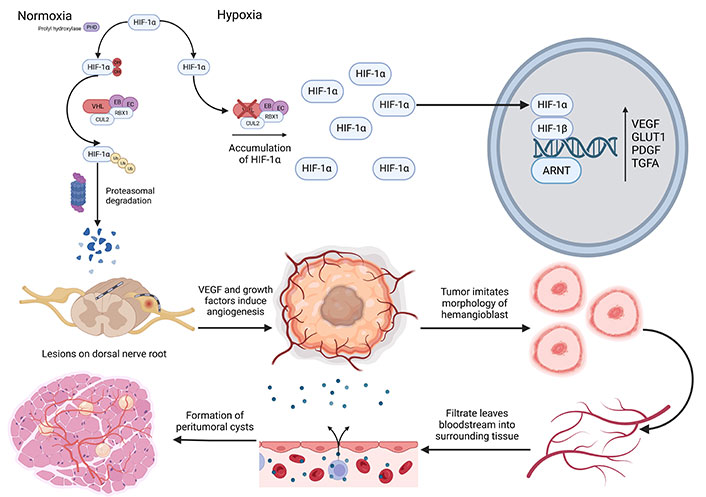
VHL-induced HB pathology. PHD: prolyl hydroxylase; OH: hydroxyl; CUL2: cullin-2; RBX1: RING-box protein 1; Ub: ubiquitin; EB: elongin B; EC: elongin C; ARNT: aryl hydrocarbon receptor nuclear transporter; GLUT1: glucose transporter 1; PDGF: platelet derived growth factor; TGFA: transforming growth factor alpha. The figure was created with BioRender.com
HBs often occur from developmentally arrested structural elements in the cerebellum’s molecular layer or in the spinal cord [16]. VHL germline mutations cause the build-up of HIF-1, subunit alpha (HIF-1α) due to the failure of the VHL complex and its inability to ubiquitinate HIF-1α where it undergoes proteasome degradation as seen in Figure 1 [26]. Following degradation, HIF-1α forms a complex with HIF-1 subunit beta (HIF-1β), triggering the activation of multiple genes such as VEGF, glucose transporter 1, platelet-derived growth factor, and transforming growth factor alpha [27]. These growth factors can increase reactive angiogenesis and form permeable HB vessels. These vessels allow the diffusion of plasma-ultrafiltrate, which primarily contains erythropoietin and forms peritumoral cysts [28]. Peritumoral cysts are associated with the neurological symptoms caused by HB [29].
Current treatments
Surgery
Maximal surgical resection remains the gold standard treatment option for cerebral tumors. The degree of resection has been significantly correlated with improved survival outcomes in patients with cerebral tumors. In a meta-analysis involving 117 distinct patients diagnosed with glioblastoma multiforme, a type of cerebral tumor, it was found that gross total resection (GTR) resulted in a significant reduction in mortality at one year compared to subtotal resection (STR), and at two years compared to early biopsy [30]. There was also an associated link between the likelihood of disease progression and the extent of resection, with GTR significantly decreasing progression at six months compared to STR [30].
Typically, a greater extent of resection is associated with greater risks of post-op complications in patients. While post-operative problems can vary depending on the anatomical location of the tumor, one of the main postoperative complications following resection is deficits in neurological function. Standardized scales are typically used to assess neurological outcomes following surgery. For example, the McCormick clinical grading scale is a conventional scale used for monitoring post-op neurological function following surgery, with a minimum score of I being normal and a maximum score of IV being severely deficient. The Karnofsky performance scale is another scale that evaluates the patient’s degree of autonomy over their daily life and quality of life after surgery [31]. Other complications following resection include cerebral spinal fluid leak, failed extubation requiring tracheostomy, epidural hematoma, wound dehiscence, superficial wound infection, and motor deficits; however, these symptoms can vary based on the tumor location [32].
Recent efforts have been made towards techniques that can be used alongside maximal resections to improve progression-free and overall survival outcomes. Intraoperative MRI (IMRI) techniques with compounds like 5-aminolevulinic acid (5-ALA), and fluorescein sodium (FS) have been shown to increase the success of surgical resection [33]. In high-grade gliomas (HGG), malignant glial cell tumors in the brain and spinal cord, intraoperative imaging using 5-ALA and FS led to higher rates of GTR compared to control [33], with 5-ALA resulting in greater rates of overall survival compared to FS [34]. IMRI was also associated with improved progression-free survival rates compared to FS and 5-ALA [33]. Intraoperative magnification with the combination of all three techniques was found to correspond to significantly higher rates of GTR in HGG [33]. While GTR has been significantly correlated to improved overall survival rates, further studies are needed to determine the association of these methods with the progression-free survival rate. Furthermore, while 5-ALA has been useful in identifying tumor margins, it has yet to show replicability in guiding surgical strategies through randomized clinical trials [35]. Additionally, visual fluorescence using 5-ALA in specifically intramedullary HBs is limited due to the morphological features of these tumors not collecting 5-ALA [35]. Indocyanine green (ICG) is a novel fluorescein dye being studied for its effectiveness intraoperatively. One study shows that ICG videoangiography is useful in locating unexposed tumors; however, further studies must be conducted [36].
A standard of care for managing HBs remains controversial, yet advancements in surgical techniques have been shown to improve patient outcomes. As a standalone treatment, GTR significantly increased progression-free survival rates compared to STR and radiotherapy in CNS HBs [37]. SRS has also been studied as a safe and effective HB treatment modality. Compared to GTR, SRS can lead to reduced tumor size with negligent long-term clinical outcomes, with local control rates ranging from 83–94% and 61–80% at 5 years and 10 years, respectively [38]. In a separate retrospective institutional review, an average of 56% of tumors decreased in size following SRS, with a mere 2% progressing at follow-up [39].
Chemotherapy
Cerebral tumors can occur through spontaneous growth or metastasis, with the latter being far more common [40]. Treatment options for cerebral tumors include surgery, radiation, and chemotherapy. Surgery and radiation are the preferred methods of treatment since delivering chemotherapeutic drugs is challenging due to the blood-brain barrier (BBB) [41]. The hydrophobic lipid bilayer, presence of tight junctions, and efflux transporters (e.g., the multidrug resistance transporter) render many conventional therapeutic drugs useless in the treatment of CNS cancer [42]. Historically, monoclonal antibodies have been the drug class of choice for treating CNS tumors [43]. These drugs have shown increasing success in cancer treatment as medical technology has advanced.
Interestingly, some studies have suggested that the BBB may be disrupted in CNS cancer. A study of murine models analyzing brain metastases found that albumin-bound Evans blue dye could cross the BBB when metastasis was present. However, this was not observed in the control group without metastasis [44]. These findings suggest that a broader spectrum of drugs may be viable in the case of brain metastasis. Further research is needed to analyze the feasibility of chemotherapies whose penetrance is normally limited by the BBB. Additionally, future studies will need to determine if the same drugs remain effective in the event of disease improvement in a patient or if the BBB renders the drugs ineffective as it typically would.
The current standard of care for HBs is surgery and radiation as detailed in Table 1 [45]. Given the sensitive nature of the CNS, surgical resection may not be possible, depending on the tumor’s location. In this instance, chemotherapy may serve as an option to provide some treatment to these patients. Unfortunately, little research has been done thus far regarding chemotherapy’s role in treating HBs. It has been hypothesized that given the highly vascular nature of these tumors, antiangiogenic therapy may have a role [46]. Case studies showed the success of other antiangiogenic agents like tyrosine kinase (anlotinib and pazopanib) and HIF-2α inhibitors (belzutifan) in managing HBs [47–49].
Standard treatment methods for HBs, diagnosis stage, and their success rates
Treatment | Stage | Success rate |
---|---|---|
GTR | At diagnosis, symptomatic lesions | 91%, 5-year survival rate [45] |
STR/Biopsy | At diagnosis, symptomatic lesions | 89%, 5-year survival rate [45] |
Whole brain radiation therapy | At diagnosis | 38.8%, 1-year survival [50] |
SRS (overall) | At diagnosis | 46.4%, 1-year survival [50] |
SRS (HB) | At diagnosis | 100%, 1-year survival [51] |
To enhance the detection and treatment of patients with gliomas, there has been a concerted effort to identify a specific biomarker that can effectively identify individuals with this condition. Understanding the pathological mechanisms by which gliomas induce an immunosuppressive response in tumor metastasis is crucial in identifying a biomarker. One of the key processes involved in this response is the production and upregulation of VEGF, which plays a significant role in tumor angiogenesis [28, 52]. A recent study by Seyedmirzaei et al. [52] has shed light on the relevance of VEGF in glioma patients. The researchers found that serum levels of VEGF in glioma patients were 0.0156 ng/L higher compared to control groups. There were also higher VEGF levels in the immunohistochemistry assessment of HGG in comparison to low-grade gliomas (LGG). Importantly, there was no notable distinction in serum levels between patients with LGG and HGG [52]. While the use of VEGF as a marker remains controversial, it is worth noting that certain VEGF gene polymorphisms have been linked to a poorer prognosis [52]. However, further studies are needed to establish the reliability of VEGF as a marker and to determine whether knowing the correlation between VEGF levels and poor prognosis can guide the choice between invasive or non-invasive treatments for improved patient outcomes. While these studies are promising, the use of antiangiogenic agents is still rather novel and requires further investigation to quantify the efficacy of antiangiogenic therapy as a potential treatment.
Radiotherapy
Approximately 200,000 patients yearly are treated with radiotherapy for primary or metastatic tumors in the United States alone [53]. Radiotherapy targets tumor DNA indirectly via free radicals produced through water ionization to damage genetic material, blocking a tumor cell’s ability to proliferate. Radiotherapy may also damage genetic material directly, causing double and single-strand breakage [54]. Damaging DNA may activate various cell death pathways, including apoptosis, mitotic catastrophe, necrosis, senescence, and autophagy [54, 55]. Radiation is commonly used for palliative care and extends median survival to 3 months to 6 months [56].
Whole-brain radiotherapy (WBRT) was the standard treatment for brain metastases for intact and resected tumors. However, a clinical trial involving 54 patients with metastatic brain tumors found that patients who received WBRT and surgical resectioning had a higher median survival length than those who received WBRT alone at 40 compared to 15 weeks [57]. Although WBRT alone has been shown to improve neurological function in 50% of patients [58], the efficacy of WBRT has been called into question due to the side effects like cognitive deterioration [59]. Intracranial radiotherapy has been found to lead to cognitive disabilities in 50–90% of patients, developing six months after irradiation [60].
As the next evolution to WBRT, SRS treats metastatic brain tumors without excessively irradiating surrounding tissue [61]. Neurological decline remains a concern using SRS, similar to WBRT. However, a recent meta-analysis found lower side-effect rates if SRS therapy was fractionated out [62]. Comparing the survival rates of SRS and WBRT, a study found that one year-survival rates were higher for SRS at 46.4% and 38.8% for WBRT [50]. Additionally, a longitudinal clinical study treating 56 HBs with a combination of SRS, stereotactic radiotherapy X-knife gamma irradiation reported local control of intracranial HBs at 98%, 88%, and 73% at 1, 2 and 6 years, respectively [63]. SRS remains a successful treatment for HBs by effectively controlling the tumors and reducing radiation-related side effects.
Another treatment called brachytherapy uses radioactive implants at the site of the tumor. It is a type of conformal radiation that spares the surrounding brain tissue, making it ideal for patients with superficial tumors. A 2021 study found that using mean brachytherapy apex doses of 144 Gy for HB improved the visual acuity of 40.5% of patients in the trial [64]. Still, multiple brachytherapy doses were needed to treat 20.5% of patients [64]. An older study found that HBs < 5.0 mm can be effectively treated with brachytherapy with a mean apex dose of 126 Gy [65].
Despite its perceived effectiveness, the integration of brachytherapy into the standard of care for treating cerebral tumors has been limited, likely due to the scarcity of data on treatment outcomes and concerns regarding the potential risks of radiation necrosis [66]. However, there has been a recent resurgence in brachytherapy with the development of GammaTile, an absorbable cesium-131-based brachytherapy [67]. GammaTile has been shown to cause minimal radiation necrosis and effective disease control, showing greater promise if used with surgical resectioning [68, 69].
Emerging HB treatments
While chemotherapies do not treat cerebral tumors effectively due to the impermeability of the BBB, there have been advances in designing chemotherapies. The most common chemotherapy drugs used are carmustine, nimustine, and lomustine [70, 71]. One potential advancement involved belzutifan, a promising anti-angiogenic HIF-2α inhibitor. Belzutifan works by inhibiting the interaction between HIF-1α and HIF-2β, thereby preventing the expression of HIF-1α growth factor gene targets. In a phase 2 clinical trial, researchers observed a positive response to belzutifan in approximately 30% of patients diagnosed with CNS HB [49].
Another example is temozolomide, an alkylating agent that has emerged as the most efficacious chemotherapy treatment for cerebral tumors, including HB. The efficacy of temozolomide can be attributed to its unique ability to penetrate the BBB [72]. Pazopanib, another promising chemotherapy option, exhibits mild adverse reactions such as transient diarrhea, oral mucosal ulcerations, and hypertension. Fortunately, the side effects associated with this therapy are relatively mild when compared to the significant benefits of treating HB and achieving clinical improvement [73]. Moreover, this therapy has been shown to enhance the patient’s quality of life by improving neurological function, swallowing ability, ambulation, and reducing dysarthria [73].
Somatostatin analog chemotherapy has also demonstrated efficacy in treating VHL-HBs. Octreotide, a commonly used somatostatin analog, reduces the viability of HB cells by promoting apoptosis through the B-cell lymphoma 2 (Bcl-2) and Bcl-2-associated X protein pathway. However, it does not downregulate growth factors, indicating the presence of an additional molecular target. In a preliminary clinical trial, patients undergoing treatment for nine months experienced a significant reduction in tumor cell volume, from 3.45 cm3 to 2.45 cm3 [74].
Immunotherapy has also emerged as a potential treatment option for HBs. One such immunotherapeutic agent is bevacizumab, a humanized monoclonal antibody vaccine that has shown promising clinical applications [75]. Theoretically, agents like bevacizumab can inhibit VEGF, disrupting the blood supply crucial for tumor growth [75]. A case study involving a man with surgically unresectable cervical cord HB, leading to quadriparesis, demonstrated significant tumor regression on follow-up MRI imaging after undergoing treatment with bevacizumab [76]. In a separate case, a female patient initially underwent an unsuccessful tumor reduction surgery and was subsequently prescribed dexamethasone and SRS. However, this treatment proved to be ineffective as the size of her HB continued to grow. As a result, bevacizumab treatment was initiated. This intervention led to significant clinical improvement, with the patient regaining her ability to ambulate and demonstrating radiographic reduction in tumor size [77].
Another group of promising therapeutic agents for treatment are β2 receptor antagonists, such as ICI-118,551 and PT2385. These antagonists interfere with HIF signaling, exhibit anti-angiogenic properties, and inhibit tumor cell migration. Notably, these agents offer an alternative to propranolol, another β-antagonist commonly used but limited to hypertensive individuals due to blood pressure-lowering effects [78, 79]. While this drug may show promising results, it is important to note that further research is necessary to determine its clinical effects. The potential benefits of treating cancer with these agents must be carefully weighed against the minimal side effects associated with the treatment, such as edema, fatigue, and anemia.
Summary of existing and novel HB treatments
HBs are highly vascularized, benign tumors most often seen in the CNS that are often associated with the formation of erythropoietin-filled cysts [15]. If left untreated, HB cells affect neurological function and, although rare, can lead to hemorrhage [15]. Current treatments for cerebral tumors include surgical resectioning, radiation treatment, and chemotherapies.
Surgical resectioning is one of the more successful treatments in treating cerebral tumors and HBs, as studies have shown it to decrease disease progression [37, 30]. The resectioning can also be assisted by intraoperative imaging like IMRI, 5-ALA, and FS has been shown to improve survival outcomes but requires more research [33]. A combination of surgical resectioning with radiotherapy demonstrates a two-fold increase in survival rates [57]. Specific radiotherapies like SRS also provide an advantage to their WBRT counterparts in improving survival rates and mitigating the risk of radiation necrosis as seen in Table 1 [50]. There is no difference in survival rates using the therapies alone or in conjunction, but using the SRS and WBRT together helps achieve better local control [80]. Brachytherapy, which is an invasive therapy that uses localized radioactive implants, has also shown effectiveness in treating HBs [64, 65]. However, it has not been adopted as the standard of care due to lack of data, inexperience in physicians, and risk of radiation necrosis [66]. A more recent and promising brachytherapy like GammaTile uses cesium-131 to mitigate the risk of necrosis and has a collagen shell around the radioactive material to allow the implant to dissolve into the body [67–69].
Chemotherapies are not widely used to treat either cerebral tumors or HBs, specifically due to the relative impermeability of the BBB to most therapeutic agents [41, 42]. However, promising results have been observed with anti-angiogenic drugs such as temozolomide, anlotinib, pazopanib, and belzutifan, which effectively inhibit VEGF and subsequently reduce the blood supply to tumors [47–49, 72, 73]. Another class of chemotherapies includes Somatostatin analogs, which promote apoptosis of HB tumor cells [74]. β-Antagonists like ICI-118,551 and PT2385, are alternative therapies that exhibit anti-angiogenic properties and disrupt tumor metastasis [78, 79]. Novel immunotherapies like bevacizumab can also treat HBs and have been shown to improve the quality of life for patients [75, 76, 81].
Conclusions
This review lays out three main treatments for cerebral tumors: surgical resectioning, radiotherapy, and chemotherapy, and enumerates their limitations. Recent research has turned to biodevices to overcome these limitations, like the impermeability of the BBB. A novel method that is being researched is to increase the efficacy of chemotherapies by using ultrasound technology to temporarily disrupt the BBB and make it more permeable to agents in the bloodstream. Researchers are currently testing a skull implant with nine ultrasound emitters to test how it may increase the efficacy of chemotherapy, influencing survival rates [82]. Another device that could be used to deliver drugs that cannot cross the BBB are microdevices that are released in the brain for a more targeted effect [83]. It could help treat specific areas of tumor growth to allow for a smoother resection or target tumors deep in the tissue. Research into HB treatments are ongoing, and scientists are still working on expanding standard care methods with novel chemotherapies, immunotherapies, targeted anti-angiogenic/somatostatin agents, and promising biodevices.
Abbreviations
5-ALA: |
5-aminolevulinic acid |
BBB: |
blood-brain barrier |
CNS: |
central nervous system |
FS: |
fluorescein sodium |
GTR: |
gross total resection |
HBs: |
hemangioblastomas |
HGG: |
high-grade gliomas |
HIF: |
hypoxia-inducible factor |
IMRI: |
intraoperative magnetic resonance imaging |
MRI: |
magnetic resonance imaging |
SRS: |
stereotactic radiosurgery |
STR: |
subtotal resection |
VEGF: |
vascular endothelial growth factor |
VHL: |
von Hippel-Lindau |
WBRT: |
whole-brain radiotherapy |
Declarations
Author Contributions
CS and MEM: Conceptualization, Writing—original draft, Writing—review & editing, Project administration. M McNulty, ER, MW, DTC, M Micunovic, JR, and JP: Writing—original draft, Writing—review & editing. BLW: Supervision, Conceptualization, Writing—review & editing.
Conflicts of interest
The authors declare that they have no conflicts of interest.
Ethical approval
Not applicable.
Consent to participate
Not applicable.
Consent to publication
Not applicable.
Availability of data and materials
Not applicable.
Funding
Not applicable.
Copyright
© the Author(s) 2023.