Abstract
Diet plays a complex role in the management of inflammatory bowel disease (IBD), significantly influencing the microbiome and metabolome. Three key metabolites implicated in IBD are short chain fatty acids, bile acids and tryptophan, all of which can be modulated through diet. This study analyses the impact of various diets on these metabolites. Despite the anti-inflammatory effects of short chain fatty acids, their levels do not increase during successful remission with exclusive enteral nutrition. Additionally, changes in tryptophan and bile acids are non-specific across different diets, suggesting these metabolic shifts are secondary to dietary efficacy in IBD. Dietary therapies vary in efficacy across individuals, as the established microbiome may not produce the desired metabolites. This variability is further compounded by differences in immune responses influenced by genetic factors and disease duration. Furthermore, inflammation and symptom resolution do not always coincide, revealing a discrepancy in dietary impacts on IBD. These limitations highlight the need for a deeper understanding of the interconnectedness of disease heterogeneity, dietary effects, the microbiome, and their influence on the mucosal immune system to develop more personalised dietary therapies. While no single diet is universally effective for all IBD patients, future research should focus on establishing a more rigid definition of dietary interventions for IBD and their long-term effects on clinical outcomes.
Keywords
Inflammatory bowel disease, Crohn’s disease, ulcerative colitis, diet, tryptophan, bile acids, microbiomeIntroduction
Inflammatory bowel disease (IBD) is characterized by inflammation of the gastrointestinal tract (GI) with symptoms such as bloody diarrhea, weight loss, abdominal pain, vomiting, fever, and tenesmus. There are two subtypes of disease: Crohn’s disease (CD) and ulcerative colitis (UC). UC manifests only in the large intestine where inflammation is continuous, whereas CD can occur throughout the GI tract and can, in addition to inflammation, show up as skip lesions [1]. The prevalence of IBD has been rising globally, with approximately 6.8 million cases worldwide in 2017 [2]. Particularly across Europe and North America where the highest prevalence rates are seen (> 0.3%) [3].
Both genetic and environmental factors such as exercise, diet and stress can contribute to the development of IBD, and its severity [4]. In particular, the adoption of a Westernized diet, often referred to as the standard American diet (SAD), has been linked to an increased risk of IBD [5]. The SAD is characterized by a high intake of processed foods, refined sugars, saturated fats and a low intake of dietary fiber [6]. The rising incidence of IBD seen in immigrant populations is suggested to be a result of the adoption of a SAD [7]. In parallel or possibly linked to the manifestation of IBD, a loss of microbial diversity in second-generation immigrants living in the US associated with changes in dietary patterns and other lifestyle factors of a Western lifestyle has also been seen [8].
Epidemiological studies have suggested that a high intake of fat, poly-unsaturated fatty acids (PUFAs), and meat are associated with increased risk of IBD [9]. The impact of diet on various immune-mediated inflammatory diseases such as rheumatoid arthritis and psoriasis has been reviewed and specific diets including the low fermentable oligo-, di-, mono-saccharide and polyol (FODMAP) diet (LFD) and the Mediterranean diet (MD) have shown beneficial effects on inflammation [10]. For IBD specifically, numerous reviews have shown there is a therapeutic effect from following restrictive diets [11–14]. Recommendations for different food components were reviewed by the International Organisation for the Study of IBD (IOIBD) and IOIBD differentiated between recommendations for UC and CD [15].
Current IBD treatments primarily focus on reducing inflammation by suppressing the immune system and targeting the inflammatory pathways directly. The first line of therapy is treatment with biologicals, e.g., anti-tumor necrosis factor alpha (anti-TNF-α), non-steroidal anti-inflammatory drugs (NSAIDs), and corticosteroids. Anti-TNF-α is one of the main biological agents used [16]. However, up to 30% of patients will be primary non-responders to anti-TNF-α and show no improvement in clinical symptoms, and up to 50% experience a loss of response or require a higher dose over time [17, 18].
Recently, mucosal healing has been recognized as a necessary therapeutic outcome for inducing and maintaining remission in IBD patients [19]. This approach is crucial because IBD is a progressive disease and even without additional clinical symptoms, intestinal damage may worsen. It has been suggested that mucosal healing can be achieved through microbiome modulation [20].
Various bacterial metabolites have been implicated in IBD pathogenesis. Among them are bile acids (BAs) [21], short chain fatty acids (SCFAs) [22], and tryptophan (Trp) [23]. Through microbiome modulation, dietary therapy may enhance the metabolome to improve a disease course. In this review, we have analyzed the effects of diet on the microbiome and the treatment of IBD with respect to the related microbiome and the major microbial metabolites as a likely mechanism for their efficacy. From this perspective, the differential effect of diets on CD and UC is considered.
Pathophysiology of IBD
IBD arises from an abnormal immune response in (genetically) susceptible individuals, triggered by environmental factors such as gut dysbiosis, antibiotic use, or viral infections [24, 25]. Normally, the immune system safeguards the body against pathogens, but in IBD, it launches an immune response against the gut epithelium leading to the characteristic inflammation. The gut epithelial lining serves as a protective barrier separating the intestinal contents from the underlying tissue. In IBD, the integrity of this barrier is compromised, exposing the tissue to luminal contents (including gut microbiota and their metabolites) to the immune cells of the intestinal mucosa. Increased infiltration of immune cells into the lamina propria is the hallmark of inflammation in IBD.
CD4+ T-cells are primarily implicated in the development of IBD. Specifically, various subsets of T-helper (Th) cells including Th1, Th2 and Th17 as well as T-regulatory (Treg) cells (FoxP3+ cells) have been identified for their role in regulating inflammation [1]. Constant regulation of these T-cell subsets is necessary for the maintenance of immune homeostasis and prevention of excessive inflammation in IBD. One key aspect contributing to the inflammatory response is pronounced activation of immune pathways, leading to elevated levels of pro-inflammatory cytokines. The presence of these cytokines exacerbates inflammation and damages the epithelial cells in the intestines. Pro-inflammatory cytokines associated with IBD include TNF-α, interleukin-1β (IL-1β), interferon-γ (IFN-γ) and IL of the IL-23/Th17 pathway [26, 27].
While the mechanisms underlying CD and UC share similarities, there are notable differences in their pathophysiology. Tissue studies show distinct cytokine patterns trending towards a Th1-dominated immune response in CD and a dominated Th2 response in UC [28]. Treg cells suppress the activation of other immune cells, including autoreactive T-cells, and modulate immune responses through the release of anti-inflammatory cytokines such as IL-10 and transforming growth factor-beta (TGF-β) [29].
Microbiome
The bacterial gut microbiome is mainly composed of 4 phyla: Firmicutes (64%), Bacteroides (23%), Proteobacteria and Actinobacteria [30]. In IBD, dysbiosis exists characterized by a decrease in microbial diversity, specifically in Bacteroidetes and Firmicutes phyla (especially Clostridium IV and XIV clusters) [31] and an increase in Actinobacteria and Proteobacteria (such as E. coli) abundance [32, 33].
Differences in the composition of the microbiome have been observed between UC and CD [33]. Species such as Campylobacter, Escherchia, Helicobacter and Mycobacterium have been implicated in CD [34]. Loss of microbial diversity, especially the loss of SCFA-producing bacteria such as Faecalibacterium prausnitzii and increase in invasive pathogenic bacteria E. coli are characteristic of IBD [35]. In UC, a higher abundance of Clostridium spp, Campylobacter, Helicobacter, Veillonella, and Bacteroides eligens among others are most commonly increased [36].
Differences in microbiome composition are also seen between IBD patients in remission compared to those with active disease. Patients with active IBD show increased levels of Bifidobacterium and Lactobacillus species [37] as well as lower diversity compared to those with inactive disease [38]. A study comparing microbial diversity in CD patients with mucosal healing and ulceration found a difference in α diversity, with an increase of Faecalibacterium, Lachnospira, Paraprevotella, Streptococcus, and Clostridium in the mucosal healing group as compared with the ulceration group [39]. Bacterial dysbiosis leads to a change in the metabolites, which can ultimately have an impact on the intestine and beyond.
Bacterial metabolites
Metabolites produced by the gut microbiome play crucial roles in host immune system modulation and gut epithelium integrity [40]. Notably, BAs, SCFAs, and Trp metabolites have been implicated in IBD pathogenesis [41]. The metabolome is closely associated with the composition of microbial communities [42], and in IBD, dysbiosis is linked to alterations in the bile acid and short-chain fatty acid pool in the colon [43].
Bile acids
BAs are secreted by the liver into the small intestine, where they facilitate the absorption of fatty acids, monoglycerides, fat-soluble vitamins, and cholesterol. The primary BAs (PBAs), namely cholic acid (CA) and chenodeoxycholic acid (CDCA), form the majority of the bile acid pool in humans. Remarkably, 95% of PBAs are reabsorbed in the ileum and recycled back to the liver, while the remaining 5% reach the large intestine where they are modified by gut bacteria into secondary BAs (SBAs). SBAs include lithocholic acid (LCA) and deoxycholic acid (DCA) (Figure 1) [21].
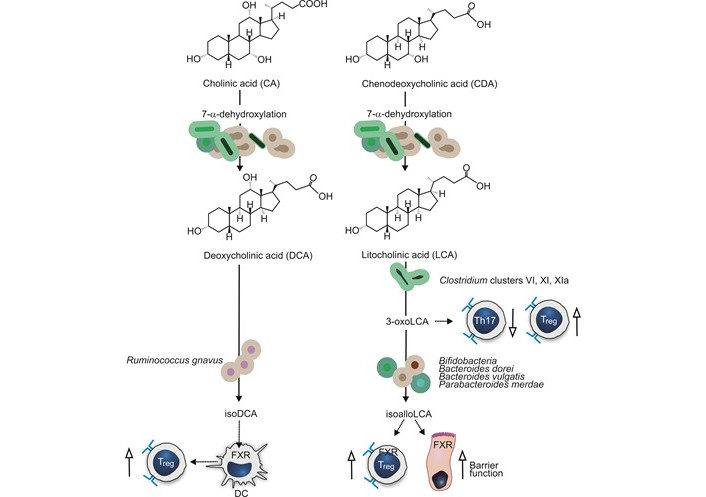
Bile acid metabolism by gut bacteria. Conversion of the primary bile acids (CA and CDA) by gut microbiota into secondary bile acids (DCA and LCA) is indicated by solid arrows. Bacteria involved in further conversion of secondary bile acids 3β-hydroxydeoxycholic acid (isoDCA), 3-oxoLCA, and isoallolLCA are indicated. The effects of bile acids on host cells are indicated with dotted arrows. Stimulating effects are indicated by up arrows with open arrowheads, inhibiting effects with down arrows. Treg: T-regulatory; DC: dendritic cell; FXR: farnesoid X receptor (further explanation is given in the text)
BAs interact with mucosal cells and modulate autophagy, inflammasome activity, and apoptosis through specific cell interactions [21]. Disruption of the bile acid system may contribute to IBD pathogenesis through these mechanisms [44]. Metagenomic profiling revealed an increase in PBAs and a reduction of SBAs in patients with IBD during flare-ups, suggesting diminished SBA-metabolizing bacteria. Moreover, an increased amount of sulphated BAs was present during flare-ups. While SBAs possess an anti-inflammatory effect by inhibiting IL-1β induced IL-8 secretion, their sulphation hampers this effect [45].
Bile acid metabolites can also modulate the immune system by balancing Th17 and Treg cell responses. 3-oxoLCA, a derivative of LCA, has been shown to decrease Th17 differentiation and promote Treg cell differentiation in mouse models [46]. IsoalloLCA enhances the differentiation of Treg cells, suggesting that these BAs and the responsible bacteria are important in maintaining immune homeostasis in the gut. Bacteria responsible for the formation of isoalloLCA from 3-oxoLCA are members of the Bacteroidetes phylum and include Bifidobacterium, Bacteroides dorei, Bacteroides vulgatus, and Parabacteroides merdae [47].
Through the interaction with the farnesoid X receptor (FXR), BAs can modulate the immune system and improve epithelial barrier function [48]. The FXR is present in many different cell types including those of the immune system [49]. isoDCA, an SBA, is suggested to increase the abundance of colonic RORγt expressing Treg cells by diminishing the activation of dendritic cells [50]. These findings emphasize the complex interplay between BAs, the gut microbiome, and the immune system in IBD.
Short chain fatty acids
Dietary fibers can be converted into SCFAs by bacteria present in the gut. The three major SCFAs- acetate, propionate and butyrate- account for 95% of the SCFAs in the gut and play essential roles in gut health and immune regulation [31]. SCFA act as ligands for the G-protein coupled receptor 41 (GPR41) and GPR43, which are involved in the release of chemo- and cytokines, promoting immune and inflammatory regulation. Butyrate and propionate inhibit histone deacetylases (HDACs) [51] and acetate has been shown to reduce the proliferation of Th1 and Th17 cells and decrease levels of inflammatory cytokines such as IL-1β and IL-6 in diabetic mice [52, 53] as depicted in Figure 2.
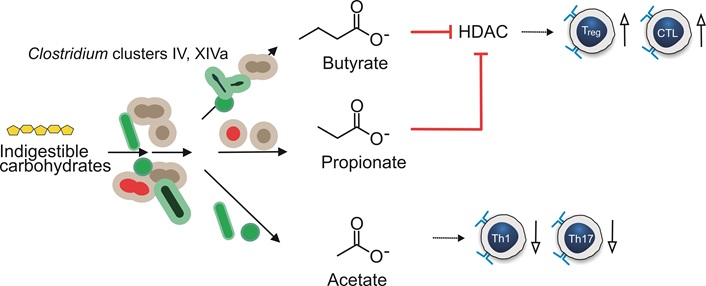
Generation of short-chain fatty acids by gut bacteria. Biochemical conversion of indigestible carbohydrates by gut microbiota is indicated by solid arrows. The effects of short chain fatty acids on host cells are indicated with dotted arrows. Blunt arrows in red indicate inhibition. Stimulating effects are indicated by up arrows with open arrowheads and inhibiting effects with down arrows. HDAC: histone deacetylase; CTL: cytotoxic T lymphocyte
Certain species, like Clostridium and Bifidobacterium metabolize dietary fiber into SCFAs, serving as an energy source for colonocytes. The main SCFA metabolizers belong to the Bacteroidetes and Firmicutes phylum [22]. These SCFAs have a vital role in promoting growth and differentiation of cells along the crypt-villus axis, aiding in epithelial restitution and repair [54]. Moreover, SCFAs have been shown to influence the differentiation of mucosal T-cells and facilitate the induction of Tregs, which are crucial in immune regulation and maintaining gut homeostasis [55].
Butyrate, the main energy source of colonocytes [56], reinforces the mucosal barrier by stimulating mucus production from goblet cells [57] and decreases luminal pH, reducing bile acid solubility [31, 58]. SCFA may influence inflammation through epigenetic regulation by inhibiting HDAC and NFκB action [59, 60]. Clostridium species, specifically clusters XIVa and IV, are prominent butyrate producers and have the ability to modify BAs and Trp (see also next paragraph) [61]. Furthermore, SCFAs, along with Trp, are dietary modulators of the aryl hydrocarbon receptor (AhR), which is under-expressed in colitis and facilitates multiple anti-inflammatory actions [62–64].
Tryptophan
Trp is an essential amino acid involved in different metabolic pathways in the gut, including the indole pathway, serotonin pathway and kynurenine (Kyn) pathway (Figure 3). Indole metabolites derived from Trp, such as indole-3-ethanol, indole-3-pyruvate, and indole-3-aldehyde (I3A), have shown protective effects against colorectal cancer and colitis in mouse models by maintaining the integrity of the apical junction complex through binding to AhR [65]. Indole and Kyn interact with the AhR and activate T-cell differentiation, reduce Th17 and Th1 responses and produce anti-inflammatory metabolites [66]. Lower Trp serum levels and higher levels of quinolinic acid (a Kyn metabolite) are associated with IBD, suggesting greater degradation of Trp in IBD [67]. Substrates of Trp metabolism such as I3A, indole-3-carboxaldehyde (3-IAld), and indole-3-propionic acid (IPA) have shown immune mediating properties through immune cell signaling and epithelial barrier renewal [68].
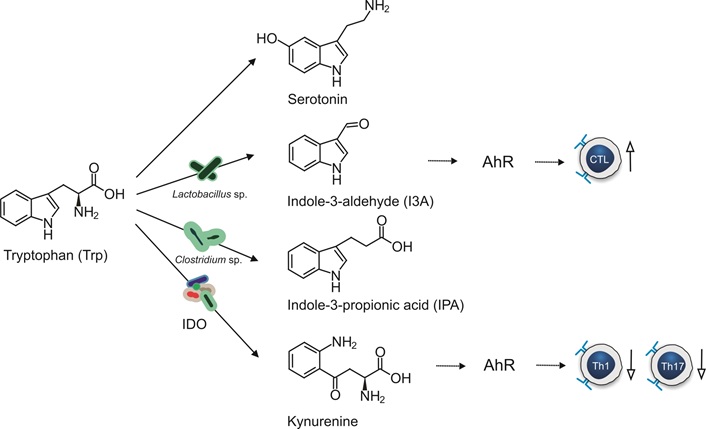
Tryptophan metabolism by gut bacteria. Stimulating effects are indicated by up arrows with open arrowheads and inhibiting effects with down arrows. Biochemical conversion of Tryptophan by gut microbiota is indicated by solid arrows. The effects metabolites on host cells are indicated with dotted arrows. IDO: indoleamine 2,3-dioxygenase; AhR: aryl hydrocarbon receptor; CTL: cytotoxic T lymphocyte
Trp promotes intestinal mucosal healing by inhibiting the production of pro-inflammatory cytokines and enhances mucosal protection by increasing the number of goblet cells, mucins, and anti-microbial peptides in mouse models [69]. Trp has also been shown to modulate the composition of the microbiome by increasing the abundance of SCFA-producing bacteria such as Acetivibrio. Furthermore, Trp contributes to intestinal homeostasis by regulating autophagy responses [69].
Lactobacillus species convert Trp into I3A, while Clostridium sporogenes and Clostridium cadaveris produce IPA. These metabolites have been shown to reduce intestinal permeability [70]. Trp supplementation has been linked to enhanced expression of tight junction proteins, such as claudin-3 and zonula occludens-1 in the jejunum of pigs [71]. 3-IAld has demonstrated anti-inflammatory effects by inhibiting the TLR4/NF-κB/p38 pathway and decreasing TNF-α, IL-6 and IL-1β in dextran sulphate sodium-induced colitis mice [72]. I3A acts on the AhR of intestinal immune cells and ensures commensal balance through IL-22 regulation [73]. Additionally, the use of recombinant enzyme aminoadipate aminotransferase has been shown to protect against colitis in mouse models through the activation of the AhR by metabolites of the Kyn pathway [74].
Analysis of diets for IBD
In the above sections, the impact of the microbiome and metabolites on the regulation of the immune system was discussed. The following section will focus on the effect of current diets for IBD and the impact of dietary interventions on the microbiome and metabolites.
Exclusive enteral nutrition
Exclusive enteral nutrition (EEN) is a liquid formula diet used in the induction of remission in CD patients [75]. This therapy involves the exclusion of all solid foods and substituting with a liquid formula for a period of 6–8 weeks. This is the first line of treatment in pediatric patients because use of corticosteroids increases protein loss impacting growth [76]. EEN is the only clinically established dietary therapy currently used in IBD and has been shown to be equally as effective at inducing remission as corticosteroids [75, 77], with improvements in endoscopic and histological scores and even improvements in growth [78–80]. This therapy may also be beneficial in adults [81], but adherence is hindered by the social implications and the unpalatable nature of the formulas [18].
The use of EEN in UC is limited but has potential as an adjunctive therapy as shown by the reduction in hospitalization of people with acute to severe UC in a randomized control trial (RCT) [82]. It is theorized that EEN may nourish the presence of beneficial microbiota, enhance bile acid metabolism, and reduce the quantity of dietary microparticles, improving disease progression and immune activity [83].
EEN reduces the diversity of the microbiome, with the most frequent shifts seen in the reduction of Firmicutes, particularly Faecalibacterium, Proteobacteria, and the Bacteroides/Prevotella ratio, while increasing Bacteroidetes (Alistepes) [84]. This disruption is believed to create an opportunity for the re-establishment of the microbiome by altering the CD-associated microbial communities and allowing other beneficial bacteria to regain their presence. However, it has been shown that this decrease in diversity is temporary. Levine et al. [85] demonstrated that the initial microbiome shift reverted to pre-treatment levels after re-introducing the habitual diet, but sustained changes were observed on a CD exclusion diet combined with EEN. Microbiome shifts observed in this study include a decrease in Haemophilus, Veillonella, Anaerostipes, and Prevotella, and increases in Roseburia and Oscillibacter.
Levels of SCFA decrease during EEN [86–88], but reintroduction of solid food reverts these changes, increasing SCFA [87], which is mirrored by an increase in Firmicutes [88]. Additionally, Verburgt et al. [88] found a decrease in PBA levels after six weeks of EEN, which returned to baseline after reintroducing a regular diet. Lv et al. [89] reported an increase in SBAs, aligning more closely with healthy controls, alongside a decrease in PBAs. They also noted an increase in Firmicutes.
Diederen et al. [90] observed an increased abundance of Faecalibacterium and Roseburia among responders to EEN. While they found no significant changes in bile acid profiles, they observed a normalized bile acid hydrophobicity in remission patients at follow-up [90].
In terms of Trp metabolites, Ghiboub et al. [91] noted a decrease in Kyn and quinolinic acid alongside an increase in serotonin metabolites, particularly melatonin and N-acetylserotonin, which were strongly associated with maintained remission [92].
Crohn’s disease exclusion diet
The CD exclusion diet (CDED) aims to mimic the exclusion of food components similar to EEN by removing foods that negatively affect the gut microbiome and are pro-inflammatory. Food groups excluded include wheat, dairy, animal fat, processed foods, and red meat. The diet includes five mandatory foods: chicken, eggs, potatoes, bananas, and apples to ensure a sufficient intake of lean protein and fiber [93].
The diet is designed with a 12-week induction phase and a maintenance phase where a gradual introduction of more foods is allowed every six weeks [94]. The induction phase stage involves a restrictive diet with a lower intake of fiber for 6 weeks; after this, during the maintenance phase, more foods that may have deleterious effects, such as bread, legumes, and red meat are reintroduced.
CDED in combination with partial enteral nutrition (PEN) has been shown to induce and maintain remission in both adults and children with CD [94, 95]. Levine et al. [85] demonstrated the superiority of CDED + PEN over EEN in children with CD, with improved tolerance and induction of remission (75% with CDED + PEN and 59% with EEN). Sigall-Boneh et al. [96] also studied the effects of CDED on patients who lost response to therapy with biologicals. Thirteen of twenty-one patients (61.9%) achieved clinical remission with CDED, showing the possibility of dietary treatment for non-responsive patients. Adherence to this diet is generally better than EEN. However, rigid protocols limit the potential for this to become a long-term maintenance therapy [14].
Levine et al. [85] showed a decrease in Haemophilus, Veillonella, Anaerostipes, and Prevotella, and an increase in Oscillibacter and Roseburia in a study comparing CDED + PEN with EEN. In CDED, this distribution continued to change over the 12 weeks, but in EEN, the diversity reverted to the original baseline microbial distribution upon reintroduction of solid foods.
Induction of remission correlated with a reduction of components of the Kyn pathway and an increase in metabolites of the serotonin pathway. This was shown in a study assessing the efficacy of CDED + PEN and EEN [92]. However, changes in the concentration of indole metabolites could not be correlated with disease outcome [92]. Verburgt et al. [88] observed a decrease in PBAs during EEN treatment but no change in any BAs with CDED + PEN. They also observed no significant change in SCFA concentrations [88]. They identified increases in Firmicutes, especially Clostridiales, Oscillibacter, Clostridia, Roseburia, and Ruminococcuss and decreases in Gammaproteobacteria.
Partial enteral nutrition
PEN includes the use of liquid formula diets for less than 100% of the daily caloric intake and a solid diet for the remaining; this varies between studies. This diet is aimed at improving tolerance of EEN by allowing some solid food consumption, providing a less restrictive version of EEN. Induction of remission with PEN has been successful [97, 98]. However, PEN has been shown to improve clinical symptoms, but not inflammatory markers or mucosal inflammation compared to EEN and anti-TNF-α treatment [99]. Additionally, CDED + PEN has shown better results than PEN alone, suggesting that there are components of a normal diet that influence inflammation and mucosal healing. Urlep et al. [100] demonstrated that PEN in combination with an anti-inflammatory diet could be just as effective as EEN.
PEN usually includes the use of another exclusionary diet. In a study assessing CDED + PEN, reduction of components of the Kyn pathway and an increase of metabolites of the serotonin pathway was seen [92].
Low fermentable oligo-, di-, mono-saccharide and polyol diet
The LFD restricts and/or excludes fermentable oligosaccharides, disaccharides, monosaccharides, and polyols, including fructose, lactose, fructans and galactans, sorbitol, mannitol, and xylitol [101]. These poorly absorbed carbohydrates are fermented in the small intestine which could lead to symptoms like bloating, diarrhea, and abdominal pain [102].
Foods high in FODMAPs include lactose products, some types of fruits and vegetables (see Table 1), legumes, and foods containing gluten. By eliminating and gradually reintroducing these foods, individuals can identify their specific sensitivities, enabling better management of diet-related symptoms.
Fruit and vegetable restrictions in the low FODMAP diet
Food group | Include | Exclude |
---|---|---|
Fruits | Strawberries, pineapple, grapes | Apples, pears, watermelon, peaches, plums |
Vegetables | Lettuce, carrot, potatoes, zucchini | Avocado, cauliflower, asparagus, broccoli |
FODMAP: fermentable oligo-, di-, mono-saccharide and polyol
The use of the LFD in IBD is not a treatment for the disease itself but for the functional gastrointestinal symptoms (FGS) related to IBD and inflammatory bowel syndrome (IBS). Symptoms of IBS are most commonly present in patients with IBD, but also in patients with quiescent IBD [103]. Both patients with IBS and IBD reported improvement of symptoms from the LFD after a mean follow-up time of 16 months [104].
Meta-analyses support the beneficial effects of the LFD in reducing FGS in patients with quiescent IBD [105]. However, evidence regarding mucosal healing and stool consistency improvement is lacking. The LFD demonstrates positive effects on symptom management for CD, but no significant effect on UC or fecal calprotectin (FC) [106]. Contrary to this, Bodini et al. [107] demonstrated a decrease in FC with LFD.
In studies investigating the impact of the LFD on gut microbial communities, it was shown that adherence to the LFD has been associated with a decrease in abundance of Bifidobacteria [108] with no changes in SCFA. Valeur et al. [109] observed an overall decrease in SCFA after the intervention. Furthermore, Cox et al. [110] found that after following the LFD, the relative abundance of Bifidobacterium longum, Bifidobacterium adolescentis, and Faecalibacterium prausnitzii decreased compared to a placebo diet, without impacting inflammatory markers or T-cell count.
Halmos et al. [111] in a study conducted in Australia, showed a decreased abundance of Clostridium cluster XIVa and Akkermansia muciniphila and increased Ruminococcuss torques in the LFD compared to a standard diet. LFD showed lower BA levels and higher IPA than a placebo diet [112].
Specific carbohydrate diet
The specific carbohydrate diet (SCD) excludes complex carbohydrates such as grains, as well as lactose and sucrose, while permitting fresh fruits and vegetables, unprocessed meats, and selected dairy products such as hard cheeses. It excludes various grains and starchy vegetables such as potatoes, whose polysaccharides can lead to increased bacterial fermentation in the colon resulting in FSG.
A retrospective study of pediatric patients following SCD showed improved disease activity indices for both CD and UC [113]. Suskind et al. [114] observed improvement of symptoms and inflammatory markers in CD patients, noting better inflammatory outcomes with more restrictive versions of the diet. A systematic review of eight studies concluded that SCD provides clinical benefits for CD management, including symptom improvement, biomarkers and successful remission induction and maintenance. However, several of the studies have methodological weaknesses [115].
Analysis of the microbiome after treatment with the SCD found an increase in Roseburia, Lachnospiraceae and Blautia species. Other species included F. prausnitzii, Anaerobutyricum and Eubacterium eligens. These changes also differed from individual to individual, showing that specific changes are not clearly associated with remission [114]. A study comparing a low residue diet to SCD found an increase in microbial diversity after the SCD and decrease in diversity in the low residue diet. They found an increase in F. prausnitzii and Clostridium species for those following the SCD [116]. In a small study assessing the effectiveness of SCD for CD in pediatric patients, a decrease in Proteobacteria was seen in most patients after 2 weeks of treatment with SCD. However, all patients had unique dysbiosis at baseline so the results are difficult to generalize [117]. Bacteroides and Parabacteroides had the largest decrease; Eubacterium, Ruminoccocus and Subdoligranulum had the largest increase.
Mediterranean diet
The MD is defined as a diet high in fruits, vegetables, nuts, legumes, and olive oil (rich in unsaturated fats), while reducing red meat intake and including moderate amounts of fish, poultry, eggs and cheese. It emphasizes consumption of whole, unprocessed foods and provides 33 g of fiber per day [118].
Studies suggesting the role of MD in the management of inflammation have highlighted the potential for this diet to be used in IBD [9, 119–121].
An observational study showed a link between adherence to an MD to lower C-reactive protein (CRP) levels and a positive correlation with the IBD Questionnaire (IBDQ), indicating better disease-related quality of life [122]. Strisciuglio et al. [123] demonstrated a correlation between adherence to MD and FC in pediatric IBD patients. Additionally, in Canadian UC patients in remission, the MD decreased FC and increased SCFA compared to a standard diet [124].
Adherence to MD has consistently shown positive effects on microbiota composition and metabolites. De Filippis et al. [125] demonstrated higher levels of SCFA with a higher adherence to MD and positive correlation between plant-based diets and Roseburia, Lachnospira and Prevotella abundance [125]. Increase in SCFA with MD was also shown by other studies [124, 126, 127]. Haskey et al. [124] found a positive association between MD and abundance of Ruminococcuss bromii and Flavonifractor plautii. A study comparing the effects of a fast-food diet and an MD demonstrated an increase of fiber-fermenting bacteria Lachnospiraceae and Butyricicoccus in the MD group. BAs did not change but Trp metabolites differed between the MD and fast-food group [128]. In patients with CD, Marlow et al. [129] observed that adherence to MD for six weeks resulted in a shift toward a more normalized gut microbe composition. This was characterized by an increase in Bacteroidetes and Clostridium clusters along with a decrease in Proteobacteria and Bacillaceace. Furthermore, adherence to MD resulted in an increase in F. prausnitzii, Roseburia, Lachnospiracea, and Clostridiales taxa and a decrease in Ruminococcus torques and Ruminococcus gnavus. This study also found a decrease in total BAs with adherence to MD, the decrease of which was associated with a decrease in Bilophila wadsworthia. Interestingly, this study did not find a significant SCFA increase despite the increase in SCFA-producing bacteria but did recognize a reduction in branched chain fatty acids (BCFA) (e.g., valerate, isobutyrate) [130].
Plant-based diet (semi-vegetarian diet)
The plant-based diet (PBD) for IBD includes daily consumption of fruits, vegetables, yogurt, potatoes, and legumes. Described as an ovo-lacto-vegetarian diet with the consumption of fish once a week and meat every 2 weeks, this diet has mainly been studied in Japan with the aim of counteracting the effect of SAD [131]. Consumption of fiber is highly emphasized in this diet with an average consumption of 32 g of fiber per 2,000 kcal [132].
Chiba et al. [133] have demonstrated the efficacy of PBD in the maintenance of remission for CD, 94% of patients who chose PBD maintained remission after a year. Additionally, 60% of those still on PBD showed normalized CRP levels. Combined induction of remission with biologics and PBD have also shown promise in CD [134].
In a long-term follow-up study, Chiba et al. [135] examined the use of PBD as an induction therapy in patients with UC. Those with moderate or severe disease were also given anti-TNF-α. Over a period of six years, patients who adhered to a PBD demonstrated lower rates of relapse. However, this study lacked a control group which limits the ability to fully interpret the results and draw definitive conclusions about the use of PBD in UC management [135].
A 3-months, ovo-lacto-vegetarian diet study revealed an increase in Roseburia, Ruminococcus and Streptococcus thermophiles with a decrease in Alistipes and Proteus mirabilis [136]. An association between a long-term vegetarian diet, a lower T-cell diversity, and a reduction in inflammatory genes was observed. Similarly, another study found that a vegetarian diet correlated with a higher ratio of Bacteroides thetaiotaomicron, Clostridium clostridioforme and Faecalibacterium prausnitzii and lower ratio of Clostridium cluster XIVa [137].
As previously mentioned, a vegetarian diet was associated with higher levels of SCFAs (butyrate, propionate and acetate) compared to an omnivorous diet. This was correlated with levels of Prevotella and Firmicutes (Roseburia and Lachnospira) [125]. Lower fecal BAs were also observed in a vegan diet compared to an omnivorous diet [138] and concentrations of Kyn metabolites in serum were shown to be reduced in vegetarians compared to omnivores [139]. In another study, Trp concentrations were highest in vegetarians and fish eaters [140].
The overview of the diets which have been analyzed for this paper is shown in Table 2.
Restrictions of exclusionary diets and their effects on the microbes
Diet | Food inclusion | Food exclusion | UC or CD | Microbial changes | SCFA | BA | Trp | References |
---|---|---|---|---|---|---|---|---|
EEN | Liquid formula | Solid food | CD | Reduced diversityDecrease in Bacteroides/Prevotella ratio↑ Firmicutes↑ Alistepes↓ Faecalibacterium↓ Proteobacteria↑ Roseburia↑ Oscillibacter↓ Haemophilus↓ Veillonella↓ Prevotella↓ Anarostipes | Decrease in SCFA (returned to baseline with reintroduction of solid food) | Decrease of PBA and increase in SBA (returned to baseline after reintroduction of solid food) | Decrease in kynurenine and quinolic acidIncrease in serotonin metabolites↑ Melatonin↑ N-acetylserotonin | [84–92] |
CDED | Include: chicken, eggs, potatoes, bananas, and apple (these are mandatory) | Exclude: wheat, dairy, animal fat, processed foods, and red meat | CD | ↑ Firmicutes ↑ Clostridiales↑ Clostridia↑ Ruminococcuss↑ Roseburia ↑ Oscillibacter↓ Haemophilus↓ Veillonella↓ Prevotella↓ Anarostipes↓ Gammaproteobacteria | No changes in SCFA | No changes in BA | Decrease in kynurenine and quinolic acidIncrease in serotonin metabolites↑ Melatonin↑ N-acetylserotonin | [87, 91, 92] |
PEN | Liquid formula and solid food | N/A | CD | N/A | N/A | N/A | Decrease in kynurenine and increase in serotonin | [91, 92] |
FODMAP | Include: certain fruits and vegetables | Exclude: certain vegetables and fruits, wheat products, mushrooms, and honey | CD and UC | ↑ Ruminococcus torques↓ Bifidobacterium (Bifidobacterium longum and adolescentis↓ Clostridium cluster XIVa↓ Akkermansia muciniphila↓ Faecalibacterium prausnitzii | Decrease in SCFA and no changes seen | Decrease in BAs | Higher concentration of 3-indole propionic acid | [108, 109, 112] |
SCD | Include: fruits, meat and vegetables | Exclude: grains, potatoes, corn, processed meat, and dairy | CD and UC | ↑ Roseburia ↑ Eubacterium eligens↑ Blautia↑ Lachnospiraceae↑ Eubacterium↑ Ruminoccocus ↑ Subdoligranulum↓ Bacteroides↓ Parabacteroides↑ F. prausnitzii↑ Clostridium | N/A | N/A | N/A | [114, 116] |
MD | Include: vegetables, fruit, whole grain, nuts, olive oil, and fish | Exclude: high red meat intake, processed foods, sugar, and processed meat | CD and UC | ↑ Roseburia↑ Lachnospira↑ Prevotella↑ Ruminococcuss bromii↑ Flavonifractor plautii↑ Butyricicoccus↑ Faecalibacterium prausnitzii↑ Clostridium cluster↑ Bacteroidetes↓ Proteobacteria↓ E. coli↓ Proteobacteria↓ Bacillaceae↓ R. torques↓ R. gnavus↓ Bilophila wadsworthia | Increase of SCFADecrease in BCFA | Decrease in BAs | Decrease of tryptophan and increase of IPA, ILA, IAA [132] | [128, 130] |
PBD | Daily intake of fruits, legumes, vegetables, potatoes, and yogurt | Avoid sweets | CD and UC | ↑ Roseburia↑ Ruminococcus↑ Streptococcus thermophiles↑ Bacteroides thetaiotaomicron↑ Clostridium clostridioforme↑ F. prausnitzii↑ Prevotella ↑ Lachnospira↓ Clostridium cluster XIVa↓ Alistepes↓ Proteus mirabilis Clostridium XIV↑ Bacteroides↑ Prevotella | Higher levels of SCFA | Lower BAs | Lower kynurenine in serumHigh levels of Trp | [125, 136, 139, 140] |
EEN: exclusive enteral nutrition; CDED: Crohn’s disease exclusion diet; PEN: partial enteral nutrition; FODMAP: fermentable oligo-, di-, mono-saccharide and polyol; SCD: specific carbohydrate diet; MD: Mediterranean diet; PBD: plant-based diet; UC: ulcerative colitis; CD: Crohn’s disease; SCFA: short chain fatty acids; BCFA: branched chain fatty acids; BA: bile acids; Trp: tryptophan; PBA: primary bile acids; SBA: secondary bile acids; IPA: indole-3-propionic acid; ILA: indole-3-lactic acid; IAA: indole-3-acetic acid. ↑: increase in relative abundance; ↓: decrease in relative abundance; N/A: not applicable
Discussion
The role of diet in clinical management of IBD is multifaceted and complex, involving the modulation of the microbiome and metabolome. Here, we explored how the different dietary approaches impact IBD, their impact on gut microbiota composition and functionality, and modulation of the mucosal immune system.
SCFA plays a crucial role in modulating immune functions and exerting anti-inflammatory effects in IBD [141]. Increasing fiber intake is a logical approach to promote SCFA production. However, SCFA are not confined to a single metabolic pathway or metabolized by a specific bacterial strain [70]. This complexity means that a diverse intake of fiber will support a broader range of beneficial bacteria and SCFA production. Nonetheless, this recommendation contradicts patient experiences. Patients report adverse effects from fiber, especially those with UC [142]. Furthermore, different types of fiber affect gut function and IBD differently [143]. The production of beneficial metabolites like SCFAs depends on the composition of the gut microbiota and their fermentation pathways [144]. Dysbiosis can significantly impact the generation of these metabolites in IBD patients. This can be further confounded by geographical differences in the microbiome of IBD patients, which are beyond the scope of this paper [145]. For further review, Wu et al. [146] analysed the metabolomic differences between vegans and omnivores, discussing the influence of geographical location on the microbiome and the limitations this creates for the production of desired metabolites.
EEN does not increase SCFA production but rather leads to a decrease [85, 86, 88]. This suggests that SCFA might not be the mechanism through which EEN induces remission. In contrast, solid exclusion diets show increased SCFA and associated bacteria, albeit with varying effectiveness.
Heterogeneity of IBD, influenced by factors such as genetic differences and duration of illness, contributes to drug unresponsiveness and loss of response over time [147]. This variability is further given the diverse effects of dietary components based on individuals’ unique microbiomes. Research has demonstrated this variability, particularly in the context of glycemic response, developing predictive models considering bacterial activity [148]. Moreover, disease heterogeneity extends to the nature of inflammation, such as in colitis, where IL-18 exhibits a dual role, with pro-inflammatory effects in later stages of disease and anti-inflammatory effects in early stages [149]. This suggests there may be different mechanisms for inducing and maintaining remission. Furthermore, the genetic and microbiota differences between UC and CD imply that distinct dietary requirements may be necessary for the treatment of these diseases [150, 151]. Therefore, understanding the interconnectedness of disease heterogeneity, dietary effects, the microbiome, microbial metabolites and their effect on the mucosal immune system of the host is crucial for developing personalized approaches to treatment and dietary management in conditions like IBD. Additionally, reducing inflammation (or inflammation biomarkers) may not lead to symptom resolution and vice versa, leaving a discrepancy in the effects of diets on IBD.
It is interesting to note that dietary therapy appears to be less studied in UC despite the significant role of diet in microbiome modulation in the large intestine. Specific modulation of gut microbiota, such as with probiotics, has been effective in the management of UC [152]. Additionally, a recent study using butyrate capsules showed efficacy in the maintenance of remission of UC [153], highlighting the potential benefits of targeting the microbiome for UC treatment.
Recommendations from the European Society for Clinical Nutrition and Metabolism (ESPEN) advocate for a diet rich in fruits and vegetables, as well as n-3 fatty acids, while minimizing intake of PUFAs as part of strategies for IBD prevention [154]. These dietary recommendations align closely with the composition of the MD, which emphasizes the consumption of fruits, vegetables, and sources of n-3 fatty acids. This suggests that adhering to the MD may offer benefits for individuals at risk of or living with IBD. The MD has already shown promise in reducing FC levels and preventing the onset of pouchitis in post-operative UC [155]. Future studies should employ more rigorous methodologies to better understand the potential therapeutic effects of the MD in managing IBD.
A high-fat diet increases bile acid production in the colon, which has been linked with colorectal cancer [156]. IBD diets limit fat intake, this is reflected in the decrease of BAs seen. EEN showed a decrease in PBAs and an increase in SBAs. SBAs may have a dual action as they can stimulate cancer cell proliferation [157] but inhibit intestinal inflammation through the activation of FXR [158]. Additionally, BAs act differently in different parts of the GI. In the jejunum, they slow down digestive transit [159], whereas they speed transit time in the large intestine [160]. This supports the idea of a different mechanism being at play between CD and UC. Metabolomic profiles differ between ileal and colonic CD, with colonic CD resembling UC and CD showing increased bile acid levels and bacteria associated with bile-rich environments such as Gammaproteobacteria and Blautia sp. [161]. Modification of BA pools could be a mechanism for the effectiveness of diet. This requires further research and differentiation between disease location and effectiveness.
The ideal diet for IBD does not exist, each of the diets discussed in this paper has its limitations. For instance, remission achieved with EEN is not long lasting, with approximately 60–70% of patients experiencing relapse within the first 12 months after the intervention [84]. However, EEN is not intended for long term maintenance therapy due to its restrictive nature, cost and other limitations [162]. Adherence to EEN formula is affected by how palatable it is and the social implication it has for patients, especially adults, as it restricts intake of solid food [163]. Therefore, adherence to this diet is quite low [164]. For this reason, patients prefer solid food diet alternatives [165].
Furthermore, the diets previously analyzed are not effective for all patients. However, biological therapy combined with EEN or PEN has been shown to be more effective at inducing remission than adalimumab monotherapy [166]. Therefore, research into dietary intervention as an adjunctive therapy to pharmacological treatment could benefit this patient population.
Additionally, the restrictive nature of the diets described above may prevent patients from benefiting from certain dietary components. For example, the LFD restricts fructans, which have been suggested to have therapeutic effects on CD by modifying dendritic cells to release IL-10 and increase Bifidobacteria [167]. Luteolin is a flavonoid present in foods such as celery, broccoli, and chicory. Luteolin has shown potential in the regulation of ILC3 lymphoid cells, decreasing disease activity in DSS colitis rats [168]. Restriction of foods containing this compound, such as when following the LFD could be depriving patients of the beneficial effects of luteolin.
The necessity for other dietary substances in the metabolism of the discussed metabolites should be considered a limitation when assessing the direct effects of diet on metabolite production [169].
Patients believe diet to be a crucial aspect of disease management, some even considering this a more important tool than medication [170]. Many patients avoid certain foods believing them to be a risk factor in relapse [171]. Roncoroni et al. [172] found that patients choose to eliminate gluten and dairy from their diets as they believed these exacerbated their symptoms. Campmans-Kuijpers and Dijkstra [173] have analyzed evidence for the effects of different food groups on human and animal IBD models. From this analysis, they synthesized a new dietary plan for IBD, called the Groningen anti-inflammatory diet. This diet is still in the testing phase for efficacy in IBD.
To enhance our understanding of optimal dietary strategies for IBD, more rigorous research is needed. This includes having more clearly defined dietary specifications, larger sample sizes, and follow-up studies to verify the lasting effects of these diets on agreed upon clinical parameters.
Conclusion
The role of diet in the management of IBD is complex. While diet undoubtedly influences the microbiome and metabolome, it may not be the primary driver for therapeutic success. Particularly with EEN, where SCFA mainly decreased despite their anti-inflammatory effects. Other diets showed an increase in SCFA but were mostly studied for maintenance of remission. Similar changes in Trp and BAs were inconsistent across studies and diets, highlighting the need for further research.
Moreover, the effectiveness of dietary interventions can be limited by the established microbiome’s ability to produce desired metabolites, as these vary between individuals and their global residence. Most diets share common features, such as increased fiber and reduced animal fat. These guidelines are recommended for the prevention of disease.
Importantly, dietary strategies should account for the specific IBD subtype and individual microbiome variations. A high-fiber, low-fat diet with lean protein sources appears broadly beneficial. It is important to distinguish between diets for inducing remission and those for maintenance.
To conclude, there is no single diet that fits all IBD patients. Personalized dietary approaches, tailored to the individual microbiome and disease subtype are essential. Further research is needed to uncover other potential metabolites and mechanisms involved in dietary treatment and to establish more practical and effective long-term dietary strategies.
Abbreviations
3-IAld: | indole-3-carboxaldehyde |
AhR: | aryl hydrocarbon receptor |
anti-TNF-α: | anti-tumor necrosis factor alpha |
BAs: | bile acids |
CD: | Crohn’s disease |
CDCA: | chenodeoxycholic acid |
CDED: | Crohn’s disease exclusion diet |
CRP: | C-reactive protein |
EEN: | exclusive enteral nutrition |
FC: | fecal calprotectin |
FGS: | functional gastrointestinal symptoms |
FODMAP: | fermentable oligo-, di-, mono-saccharide and polyol |
FXR: | farnesoid X receptor |
GI: | gastrointestinal tract |
HDACs: | histone deacetylases |
I3A: | indole-3-aldehyde |
IBD: | inflammatory bowel disease |
IBDQ: | inflammatory bowel disease questionnaire |
IBS: | inflammatory bowel syndrome |
IL-1β: | interleukin-1β |
IOIBD: | International Organisation for the Study of Inflammatory Bowel Disease |
IPA: | indole-3-propionic acid |
isoDCA: | 3β-hydroxydeoxycholic acid |
Kyn: | kynurenine |
LCA: | lithocholic acid |
LFD: | low fermentable oligo-, di-, mono-saccharide and polyol diet |
MD: | Mediterranean diet |
PBAs: | primary bile acids |
PBD: | plant-based diet |
PEN: | partial enteral nutrition |
PUFAs: | poly-unsaturated fatty acids |
SAD: | standard American diet |
SBAs: | secondary bile acids |
SCD: | specific carbohydrate diet |
SCFAs: | short chain fatty acids |
Treg: | T-regulatory |
Trp: | tryptophan |
UC: | ulcerative colitis |
Declarations
Acknowledgments
We thank Prof. B. Witteman (emeritus from Wageningen University and Research, Wageningen, The Netherlands) for stimulating discussions during the preparation of the manuscript, and Dr. T. Dinse (University College Roosevelt, Middelburg, The Netherlands) for correcting the language and style of the manuscript.
Author contributions
HM: Conceptualization, Investigation, Writing—original draft, Writing—review & editing. GR: Validation, Writing—review & editing, Supervision. Both authors read and approved the submitted version.
Conflicts of interest
Not applicable.
Ethical approval
Not applicable.
Consent to participate
Not applicable.
Consent to publication
Not applicable.
Availability of data and materials
Not applicable.
Funding
Not applicable.
Copyright
© The Author(s) 2025.
Publisher’s note
Open Exploration maintains a neutral stance on jurisdictional claims in published institutional affiliations and maps. All opinions expressed in this article are the personal views of the author(s) and do not represent the stance of the editorial team or the publisher.