Abstract
Neoantigen vaccines are a promising strategy in cancer immunotherapy that leverage tumor-specific mutations to elicit targeted immune responses. Although they have considerable potential, development challenges related to antigen prediction accuracy, manufacturing complexity, and scalability remain key obstacles to their widespread clinical use. This literature review was conducted using PubMed, Scopus, Web of Science, and Google Scholar databases to identify relevant studies. Keywords included “neoantigen vaccines,” “personalized cancer immunotherapy,” “tumor heterogeneity,” “bioinformatics pipelines,” and “prediction algorithms”. Clinical trial data were sourced from ClinicalTrials.gov, Trialtrove, and other publicly available registries. Eligible studies included peer-reviewed research articles, systematic reviews, and clinical trials focusing on neoantigen vaccine development, bioinformatic strategies, and immunotherapy. Tumor heterogeneity and clonal evolution significantly impact vaccine efficacy, necessitating multi-epitope targeting and adaptive vaccine design. Current neoantigen prediction algorithms suffer from high false-positive and false-negative rates, requiring further integration with multi-omics data and machine learning to enhance accuracy. Manufacturing remains complex, time-intensive, and costly, necessitating advancements in standardization and automation. Combination therapies, such as immune checkpoint inhibitors and adoptive cell therapies, counteract the immunosuppressive tumor microenvironment, improving treatment outcomes. Neoantigen vaccines hold great potential for personalized cancer therapy but require advancements in bioinformatics, manufacturing scalability, and immunomodulatory strategies to enhance clinical efficacy. Continued research and interdisciplinary collaboration are essential for refining clinical applications.
Keywords
Neoantigen vaccines, personalized immunotherapy, tumor heterogeneity, bioinformatics pipelines, prediction algorithms, cancer vaccines, immunological memoryIntroduction
Neoantigen vaccines represent a key development in personalized immunotherapy, explicitly targeting tumor-specific antigens unique to each cancer type. In simple terms, they work by leveraging the immune system to selectively attack cancer cells [1, 2]. Sophisticated techniques have been developed to identify, validate, and manufacture personalized neoantigen vaccines, thereby enhancing efficacy with the least possible off-target effects [2, 3]. The therapeutic potential of neoantigen vaccines has been demonstrated through early-phase clinical trials across several cancer types, showing their ability to induce potent and durable immune responses, with possible clinical benefits [4]. As a result, neoantigen vaccines are emerging as a promising component of personalized cancer treatment, complementing the advancement of precision medicine in oncology.
Neoantigens arise from unique genetic alterations that differ across individuals, making neoantigen-based immunotherapy a fully personalized treatment [5–7]. Personalized neoantigen vaccines have emerged as a promising approach to melanoma immunotherapy. Vaccines targeting neoantigens have exhibited remarkable promise in the realm of melanoma immunotherapy. Recent studies [6, 8, 9], support these findings, collectively, demonstrating that personalized immune responses can be effectively induced in melanoma patients. These results highlight neoantigen vaccines as a feasible and safe treatment modality [1, 10, 11]. Furthermore, dendritic cell (DC)-based vaccines targeting neoantigens have been demonstrated to enhance neoantigen-specific T cell responses in melanoma, thereby increasing both immune breadth and diversity. According to published clinical trials, evaluating neoantigen-based cancer vaccines have exhibited antitumor efficacy in melanoma and glioblastoma patients [10, 12, 13]. The integration of immunotherapeutic agents with sophisticated formulations and delivery routes is illustrated in Figure 1, showcasing strategies designed to optimize cancer immunotherapy and improve patient outcomes.
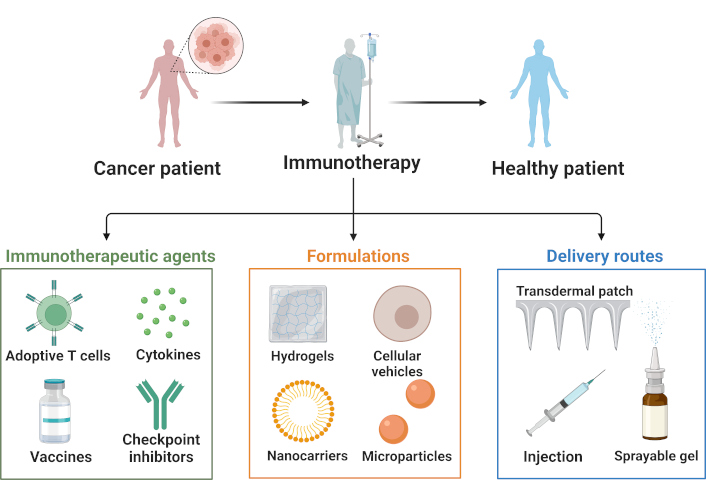
Overview of cancer immunotherapy strategies. The figure outlines the fundamental elements of immunotherapy, encompassing therapeutic agents, formulation approaches, and administration methodologies. Immunotherapeutic interventions, such as adoptive T cell therapy, cytokines, vaccines, and checkpoint inhibitors, are pivotal in orchestrating host immune responses. These therapeutic entities are integrated into sophisticated delivery platforms, including hydrogels, nanocarriers, cellular vehicles, and microparticles. The efficacy of cancer treatment is augmented through diverse administration routes, such as transdermal patches, injections, and sprayable gels, which optimize the delivery and potency of immunotherapeutic agents in clinical settings. Created in BioRender. Bashatwah, R. (2025) https://BioRender.com/m88i139
Preliminary reports from various ongoing studies using personalized long-peptide neoantigen cancer vaccines have also shown significant antitumor immune responses and early clinical activity in patients with advanced melanoma treated with such vaccines [9]. Research has demonstrated that neoantigen vaccines derived from DCs have successfully elicited T cell-mediated immune responses in a subset of patients with melanoma [14, 15]. Research has shown that neoantigen vaccination can lead to the development of long-lasting memory T cell responses and promote epitope spreading in individuals diagnosed with melanoma [16]. Notably, neoantigen vaccines induce antitumor immunity while minimizing autoimmune toxicity, due to their unique ability to specifically stimulate immune responses against mutations exclusive to tumor cells [16]. Notwithstanding recent technological advancements in the identification and prioritization of cancer neoantigens, significant challenges persist in optimizing neoantigen selection and addressing the immunosuppressive tumor microenvironment [17].
The incidence of melanoma, the most lethal form of skin cancer, continues to increase globally. In the United States alone, an estimated 99,780 new cases were projected in 2023, with approximately 7,650 melanoma-related fatalities anticipated [6, 18, 19]. Despite advancements in surgical techniques, radiation therapy, and targeted therapeutic approaches, the recurrence of disease and the development of therapeutic resistance remain significant challenges in clinical practice [20]. Despite the advent of immune checkpoint inhibitors, which have significantly altered the therapeutic landscape, attaining sustained responses and long-term remission remains a substantial challenge [21]. This persistent clinical challenge underscores the critical necessity to investigate novel therapeutic approaches, with neoantigen vaccines presenting a highly promising avenue for advancing melanoma treatment [19].
Neoantigen vaccines differ from conventional cancer therapies by targeting tumor cells through their distinct mutational signatures, rather than common oncogenic pathways [22]. These tumor-specific mutations generate novel neoantigen-peptide sequences that are absent from normal cells and can potentially activate a patient’s immune system as a potent mechanism in the individual’s defense against cancer [23]. Through the presentation of these unique antigens, neoantigen vaccines effectively stimulate the immune system to recognize and eliminate malignant cells with high specificity, resulting in tumor regression and the induction of long-lasting immunological memory [24]. The elevated mutational burden and extensively characterized tumor antigens associated with melanoma render it an ideal candidate for the development of neoantigen vaccines [25]. The clinical efficacy of immune checkpoint inhibitors in melanoma provides a compelling rationale for investigating their synergistic potential with neoantigen vaccines, potentially augmenting the magnitude and longevity of antitumor immune responses. This manuscript presents a comprehensive review of neoantigen vaccine design principles, recent clinical findings pertaining to melanoma, and critical challenges and future directions in this field of research [26, 27].
This study examines neoantigen discovery and selection methodologies, vaccination platforms, and the function of adjuvants in boosting immunogenicity. Furthermore, outcomes from early-phase and current clinical studies are examined to get insight into the therapeutic potential of neoantigen vaccines. In this study, we underline that neoantigen vaccines are a highly individualized and promising method for melanoma therapy, bringing fresh hope for tackling this life-threatening illness [2, 4, 15].
The global health landscape continues to be significantly impacted by melanoma, as its incidence rates have shown a persistent upward trend over recent decades without a corresponding reduction in mortality rates [28]. In 2014, melanoma was responsible for 75% of skin cancer-related mortalities in the United States, with an estimated 76,100 new cases and 9,710 fatalities [29, 30]. The annual increase of 4–6% in global incidence rates highlights the critical need for innovative therapeutic approaches [31, 32]. Although recent advancements have been made, the persistent challenge of long-term melanoma-related mortality remains, evidenced by survival rates as low as 24.6% at 15 years following treatment. This underscores the critical need for therapeutic approaches that yield enduring benefits [33, 34]. Although melanoma mortality rates have stabilized in certain populations, they continue to exhibit significant increases in others, underscoring the imperative for enhanced prevention strategies and expanded access to immunotherapies [35].
The increasing rates of incidence and mortality observed in countries such as Italy and Spain highlight the “melanoma epidemic”, underscoring the importance of improved strategies for treating and managing this condition [36]. A significant factor contributing to melanoma’s high mortality rate is its ability to resist standard chemotherapy treatments, which intensifies the need for innovative targeted therapies [18].
Cancer treatment is being revolutionized by personalized immunotherapy, which combines patient-specific molecular information, tumor-specific antigens, and cutting-edge pharmaceutical methods. The effectiveness of cancer immunotherapy has been demonstrated through numerous clinical validations, leading to widespread acceptance of this approach [37]. Immunotherapy approaches, including adoptive cell therapy (ACT) utilizing tumor-infiltrating lymphocytes and chimeric antigen receptor (CAR) T cells, have demonstrated encouraging clinical results. Simultaneously, immune checkpoint inhibitors continue to transform the landscape of immunotherapy treatments [38, 39]. A new investigation has shown the effectiveness of a dual-targeted personalized immunotherapy strategy. This approach involves linking cytotoxic T cell epitopes to a patient’s own T cells using IgG Fc-CD3/CD28 signaling domains. In certain patient groups, this method has yielded impressive response rates ranging from 80% to 90% [40, 41]. The remarkable feature of this, noted by the demonstrators above in the so-called personalized immunotherapy, is that clinical trials have been summarized with response rates close to 80–90%, based on the selection of patients with tumor-specific antigens [42]. Most antigens and antigen-presenting cells (APCs) have been developed and documented using a single developer. Personalized immunotherapy goals are to provide the patient with a customized treatment cancer vaccine that can be applied in any clinical center using equipment that is already in clinical use and can obtain a sufficient number of lymphocytes, which is known as leukapheresis [43]. Neoantigen vaccines represent a cutting-edge approach to tailored cancer therapy. By harnessing tumor-specific mutations, these vaccines facilitate the creation of highly customized immunotherapies, sidestepping the traditional obstacles of chemotherapy resistance and reducing collateral damage. Recent advancements in bioinformatics, coupled with the integration of multi-omics data and artificial intelligence, have significantly enhanced the accuracy of neoantigen prediction. Nevertheless, challenges in large-scale manufacturing and clinical application persist. As ongoing clinical trials continue to demonstrate their efficacy, neoantigen vaccines are set to reshape the landscape of cancer immunotherapy, ushering in a new era of personalized treatment strategies.
This study evaluated the efficacy of T cell receptor (TCR) gene therapy targeting two immunogenic mutations in cyclin-dependent kinase 4 (CDK4), R24C (p.Arg24Cys), and R24L (p.Arg24Leu), in human melanoma [44–46]. Both mutations triggered TCRs in vitro. However, R24L exhibited a stronger antitumor effect than R24C in a syngeneic mouse model with advanced tumors. Mutation-specific TCRs were engineered to target both CDK4 isoforms, with R24L demonstrating superior therapeutic efficacy [46, 47]. These findings underscore the critical role of neoantigen quality in cancer immunotherapy, suggesting that variations in neoantigen characteristics may contribute to differences in treatment responses, even among patients with comparable mutational burdens. The efficacy of the in vivo model was substantiated through the observation of tumor rejection following the introduction of epitope-specific TCRs. Verification of functional T cell activation in vivo was accomplished by examining serum concentrations of interferon gamma, a crucial cytokine in the tumor rejection process. To elucidate disparities between datasets, comprehensive statistical analyses were employed [47–49].
Despite the yearly 4–6% rise in global melanoma cases, with 99,780 new diagnoses expected in the U.S. for 2023, current treatment methods offer limited long-lasting benefits. The 24.6% mortality rate 15 years after diagnosis highlights the urgent need for more effective, long-term treatment solutions. Neoantigen vaccines have surfaced as a cutting-edge therapeutic approach, utilizing tumor-specific mutations to elicit highly individualized immune responses. This method addresses many shortcomings of traditional chemotherapy, providing greater precision while minimizing autoimmune side effects [50].
The field of neoantigen identification has seen substantial progress due to innovations in bioinformatics, multi-omics analysis, and machine learning. Yet, obstacles persist in enhancing predictive accuracy and scaling up production processes. Recent clinical studies focusing on melanoma and glioblastoma have further substantiated this approach’s effectiveness, particularly when combined with immune checkpoint inhibitors, indicating a pivotal shift in cancer immunotherapy strategies. Despite ongoing concerns regarding standardization and cost-efficiency, neoantigen vaccines demonstrate the potential to induce sustained immune responses and long-lasting immunological memory. Consequently, this vaccine platform is poised to revolutionize personalized cancer treatment. Neoantigen vaccines, which integrate genomics, immunology, and precision medicine, offer a promising direction for advancing individualized oncology therapies. The key advantages of this approach are summarized in Table 1.
SWOT (Strengths, Weaknesses, Opportunities, and Threats) analysis of neoantigen vaccines as a personalized immunotherapeutic strategy for cancer treatment
Category | Elements |
---|---|
Strengths |
|
Weaknesses |
|
Opportunities |
|
Threats |
|
This analysis systematically evaluates the intrinsic advantages and limitations of neoantigen-based vaccines, as well as external factors that could impact their clinical development and implementation. Strengths and weaknesses reflect internal capabilities, including immunogenicity, specificity, and scalability challenges, while opportunities and threats account for regulatory, technological, and market-driven influences in clinical oncology
Although several reviews have previously covered the application of neoantigens in cancer immunotherapy, including their clinical relevance and future prospects [2, 11, 51, 52]. This manuscript provides a distinct emphasis on bioinformatics-driven neoantigen prediction. Specifically, it critically evaluates the strengths and limitations of existing prediction algorithms and sequencing technologies.
This review will evaluate some major hurdles in clinical translation, such as tumor heterogeneity, vaccine manufacture at scale, and combinations of immunomodulatory therapies. It provides a thorough examination of both the computational and translation aspects in regard to next-generation neoantigen-based therapies in the field setting.
Principles of neoantigen vaccine design
Neoantigen vaccines remain promising approach, with their potential primarily relying on their ability to direct the immune response toward tumor-specific mutations. The identification of optimal neoantigens involves a multi-step process: the selection of an appropriate vaccine platform and the integration of immunogenicity-enhancing strategies [2, 26, 32, 35].
Identification of neoantigens
Next-generation sequencing (NGS) technologies have significantly advanced the identification of somatic mutations from tumor biopsies, providing a comprehensive understanding of the genomic landscape of cancer. NGS has shown notable improvements in reliability, sequencing chemistry, data interpretation, and cost efficiency [32, 53]. These advancements have enabled precise profiling of both gene expression and the mutational landscape in liquid biopsies, including circulating tumor DNA (ctDNA) and circulating tumor cells (CTCs) [53].
NGS has facilitated high-resolution and large-scale detection of somatic mutations, as demonstrated in multiple studies [54, 55]. This technology enables the analysis of millions of DNA fragments, enhancing the efficiency of identifying targetable genetic mutations in metastatic colorectal cancer and directing the implementation of individualized treatment strategies. The integration of NGS into clinical practice has steadily increased, driven by advancements that have reduced costs, improved efficiency, and minimized tissue requirements [56].
NGS panels are widely utilized for characterizing tumor mutation profiles, published research has consistently demonstrated that accurately predicting patient outcomes and informing treatment strategies is a crucial element in the clinical decision-making process [53, 57]. These screening tools provide an exceptionally sensitive method for detecting mutations and have played a crucial role in uncovering new somatic mutations linked to various types of cancer [58]. Moreover, ultra-deep plasma NGS assays have facilitated the detection of targetable oncogenic drivers and resistance mutations in patients with non-small-cell lung cancer (NSCLC), especially in situations where tissue sampling fails to reveal targetable mutations [59]. The detection of somatic mutations in tumor samples has been greatly improved by next-generation sequencing (NGS) technologies, offering a more comprehensive understanding of the genetic changes that contribute to cancer development and progression [59]. Advancements in NGS not only improve the accuracy and sensitivity of mutation detection but also support the integration of personalized medicine by enabling genomic profiles of individual tumors.
NGS platforms for mutation detection
NGS technologies are rapidly becoming a cornerstone of personalized cancer therapy, particularly for melanoma [60]. NGS plays a crucial role in unraveling the intricate landscape of somatic mutations, offering valuable insights for creating personalized neoantigen vaccines for individual patients [61]. NGS with a targeted approach hones in on specific regions of the genome known for harboring mutations, facilitating exceptionally sensitive and accurate detection. On the other hand, whole-exome sequencing examines all protein-coding regions of the genome for mutations, offering a more comprehensive perspective, though at a significantly higher expense. Whole-genome sequencing (WGS) takes this approach even further by analyzing the entire genome, including non-coding areas, to provide a complete picture of genetic variations [62]. From platform such as Illumina and Ion Torrent to Pacific Biosciences, coupled with sophisticated bioinformatics analysis tools including VarScan [63], MuTect, and GATK, advancements in microarrays and NGS have significantly enhanced the accuracy and speed of cancer genome analysis [64, 65]. These evolving technologies continue to drive innovation in melanoma treatment, facilitating personalized immunotherapeutic strategies based on the unique genetic profile on a patient’s tumor [66].
Comparison between neoantigens and tumor-associated antigens
Neoantigens and tumor-associated antigens (TAAs) represent two distinct classes of tumor antigens that play critical roles in cancer immunotherapy [67]. Although both can be targeted by immune-based therapies, their distinct origins, immune-stimulating properties, therapeutic uses, and constraints shape their specific roles in treatment approaches [22]. Neoantigens are tumor-specific antigens that originate from somatic mutations, gene rearrangements, or alternative splicing in tumor cells. The absence of neoantigens in normal tissues contributes to their high tumor specificity, rendering them prime candidates for tailored cancer immunotherapy approaches [68]. In contrast, TAAs are self-antigens that are either overexpressed or re-expressed in tumor cells but are also present at lower levels in normal tissues. These distinctions influence their immunogenicity and therapeutic potential [68].
Neoantigens elicit robust immune responses as they are recognized as foreign (non-self) by the immune system. Due to their absence in normal tissues, they circumvent central and peripheral immune tolerance mechanisms, rendering them highly efficacious in eliciting anti-tumor immunity. Conversely, TAAs exhibit diminished immunogenicity as they originate from normal proteins subject to immune tolerance. Consequently, additional strategies, such as immune checkpoint inhibitors, are frequently requisite to augment immune recognition and response [69].
Neoantigens are highly suitable for individualized cancer immunotherapy, serving key targets for neoantigen-based vaccines and adoptive T cell therapies. Their high specificity enables precise tumor targeting while minimizing the risk of damage to normal tissues. In contrast, TAAs, are more applicable in broader immunotherapeutic approaches, including monoclonal antibodies and checkpoint inhibitors, which enhance the immune response against tumor cells expressing these antigens [52, 68].
Despite their advantages, both antigen classes present distinct challenges. Neoantigen-based therapies require complex computational challenges and approaches to identify patient-specific neoantigens using sequencing technologies. Additionally, the production of personalized therapies is labor-intensive, time-consuming, and costly. TAA-based therapies, while more broadly applicable, pose a risk of off-target effects and autoimmune toxicity due to TAAs expression in certain normal tissues, which can lead to unintended immune responses [51, 70]. The contrasting characteristics of neoantigens and TAAs underscore their unique functions in cancer immunotherapy. Neoantigens provide a highly individualized and cancer-specific approach to treatment, while TAAs offer broader therapeutic targets that necessitate additional measures to combat immune tolerance and reduce off-target effects. A thorough understanding of these distinctions is crucial for enhancing immunotherapeutic approaches and boosting treatment efficacy in individuals with cancer. The optimization of cancer immunotherapy strategies relies heavily on recognizing and leveraging these differences between neoantigens and TAAs [22].
Pipelines for neoantigen prediction: algorithms, filtering criteria, and human leukocyte antigen (HLA) allele-specific prediction
Bioinformatics pipelines play a critical role in predicting neoantigens from tumor genomic and transcriptomic data, facilitating the identification of potential targets for personalized cancer immunotherapy. These pipelines integrate sophisticated algorithms and filtering criteria that account for key factors such as immunogenicity and HLA allele specificity [71, 72]. The field of neoantigen prediction has witnessed the development of multiple bioinformatics tools. Among these, ScanNeo2 and nextNEOpi stand out as advanced, fully automated systems. These pipelines excel in their ability to identify tumor neoantigens by directly processing raw DNA and RNA sequencing information [73–75]. Advanced algorithms are utilized in these instruments to scrutinize genomic changes and assess the immunogenic potential of anticipated neoantigens [76]. Another high-throughput neoantigen prediction pipeline, NeoPredPipe, employs NetMHCpan for HLA allele-specific neoantigen prediction. In addition to predicting neoantigens, NeoPredPipe evaluates their immunogenic potential, thereby enhancing the accuracy of neoantigen identification [75].
The establishment of guidelines for neoantigen bioinformatic characterization has delineated crucial parameters, encompassing variant identification, anticipated modifications in amino acid sequences, peptide sequence composition, predictions of binding affinity, and agretopicity values [72, 77]. Neoantigen prediction pipelines, such as TruNeo, incorporate multiple biological parameters, including peptide—MHC class I binding affinity, proteasomal cleavage efficiency, and expression abundance, to enhance prediction accuracy. Furthermore, these pipelines consider tumor heterogeneity, clonality, and HLA loss of heterozygosity (LOH), thereby refining neoantigen identification and improving the selection of clinically relevant targets [78, 79].
Despite advancements in neoantigen prediction, the accurate identification of highly immunogenic neoantigens remains a significant challenge. The limited precision of current bioinformatics pipelines constrains their capacity to reliably identify neoantigens capable of eliciting protective T cell responses, thus necessitating further refinement [51, 79]. Bioinformatic neoantigen prediction pipelines play a crucial role in neoantigen-based cancer immunotherapy. Through the utilization of advanced algorithms and HLA allele-specific predictions, these tools facilitate the identification of candidate neoantigens for personalized therapeutic strategies. Continued advancements in these pipelines are essential for the development of more efficacious and targeted cancer treatments.
Tumor heterogeneity and clonal pose significant challenges in cancer research and treatment, particularly in neoantigen prediction and immunotherapy [80]. Tumors consist of genetically diverse, resulting in the development of evolving subclonal neoantigens, which impact immune system monitoring and responses to treatment over time [81]. The presence of subclonal neoantigens complicates the identification of immunogenic targets for cancer immunotherapy [22]. Current research emphasizes the significance of neoantigen heterogeneity in immune surveillance, revealing that an elevated subclonal neoantigen load is linked to tumor development and immune avoidance tactics. By integrating subclonal neoantigens into prediction models, the accuracy of neoantigen identification could be substantially enhanced, potentially leading to improved immunotherapeutic strategies [82, 83].
Tumor evolution introduces an additional layer of complexity to neoantigen prediction and immunotherapy [84]. Clonal evolution drives intratumoral heterogeneity, wherein distinct tumor subclones possess diverse genetic profiles, resulting in heterogeneous antigenic landscapes. Continuous genetic alterations influence the efficacy of neoantigen-targeted immunotherapies, as tumor antigens evolve concomitantly with immune system responses throughout the course of treatment [81, 85].
Such a bioinformatics pipeline is urgently needed, capable of predicting neoantigens while considering of tumor evolution dynamics to overcome tumor heterogeneity and clonal evolution. Various works underlined that multi-region sequencing, in association with the analysis of clonal architecture, may lead to the disclosure of spatial and temporal patterns of tumor evolution and enable insights into intratumor heterogeneity and clonal dynamics [71, 75].
Tumor heterogeneity and clonal evolution represent a significant challenge, and advanced bioinformatics tools and deep analyses that consider subclonal neoantigens and tumor evolution over time are essential. These factors need to be integrated into neoantigen prediction pipelines and immunotherapeutic strategies to increase prediction accuracy and improve the efficacy of personalized cancer treatments [81, 86].
Current clinical landscape of neoantigen vaccine
Neoantigen vaccines are being actively investigated for several types of cancer, including melanoma, glioblastoma, non-small cell lung cancer (NSCLC), and pancreatic cancer. These vaccines target tumor-specific mutations, eliciting strong immune responses through cytotoxic T cell activation. Their ability to generate highly tumor-specific antitumor activity has made them promising candidates for personalized cancer immunotherapy [87, 88].
Up to late 2022, 199 clinical trials were reported, of which Phase I studies represented the majority (59.8%) according to the Trialtrove database. Peptide-based vaccines are still the most extensively explored, while mRNA and DC-based platforms have gained popularity owing to their potential for tailored and adaptive immunotherapy. The appeal of mRNA-based neoantigen vaccines has been enhanced by the development of RNA stability, delivery technologies, and large-scale manufacturing [1, 88].
Early phase clinical trials have evaluated the safety and immunogenicity of neoantigen vaccines. For instance, in glioblastoma, enhanced intratumoral T cell infiltration has been observed following vaccination, implying an intensified local immune response. Furthermore, combination therapies with immune checkpoint inhibitors, such as nivolumab and ipilimumab, or adjuvants like poly-ICLC, have enhanced vaccine activity by promoting T cell activation and contracting tumor immune evasion mechanisms [89].
Neoantigen vaccines are being explored for a variety of cancer types, with NSCLC, melanoma, glioma, and pancreatic cancer being the most common indications. Triple-negative breast cancer (TNBC) has also emerged as an area of interest because it carries a high mutational burden, making it an attractive candidate for neoantigen-based immunotherapy [1]. In terms of vaccine delivery systems, peptide vaccines were the most common, accounting for 41% of neoantigen vaccine trials. Nevertheless, mRNA-based vaccines and liposomal delivery systems are rapidly emerging as promising options considering their flexibility in encoding patient-specific neoantigens. DC-based platforms, accounting for 16.1% of trials, have the advantage of direct antigen presentation to T cells, but pose challenges related to ex vivo manipulation and scalability [90, 91].
Neoantigen vaccines are frequently applied in combination with standard cancer treatments, including chemotherapy, radiation therapy, and immune checkpoint inhibitors. These combination regimens aim to enhance therapeutic efficacy by modulating the tumor microenvironment and promoting long-term antitumor immunity [4]. Despite these promising advances, several challenges remain in the widespread clinical implementation of neoantigen vaccines. One of the most significant obstacles is the computational complexity involved in identifying patient-specific neoantigens. This process requires high-throughput sequencing, advanced bioinformatics pipelines, and machine learning algorithms to accurately predict immunogenic epitopes. Additionally, the manufacturing of personalized vaccines is still labor-intensive and costly, necessitating advancements in automation and scalability. Patient recruitment also presents a challenge, as the stringent inclusion criteria for neoantigen vaccine trials limit enrollment, sometimes leading to the early termination of studies [15, 51, 92].
It is anticipated that ongoing technological advancements in sequencing and bioinformatics pipelines will facilitate neoantigen discovery, enhancing both accuracy and efficiency in vaccine development. Moreover, innovative adjuvants and delivery systems may improve vaccine efficacy by optimizing antigen presentation and immune stimulation. Expanding clinical trial designs to encompass more diverse patient populations will be essential for increasing the generalizability of neoantigen vaccine strategies and demonstrating broader clinical utility.
Neoantigen vaccines represent one of the most promising approaches in personalized cancer immunotherapy, and current clinical trials continue to elucidate their potential to transform treatment strategies for various cancer indications. However, further refinement in vaccine design, patient stratification, and manufacturing processes is necessary to enhance their clinical utility and facilitate their integration into mainstream oncology practice [22, 87].
Vaccine platforms
Once candidate neoantigens have been defined, an appropriate vaccine platform is required to present these neoantigens to the immune system. Several vaccine platforms are available, each with its advantages and disadvantages [2, 17].
Peptide vaccines consist of synthetic peptides that mimic neoantigens. Well-defined epitopes and their ease of production make them attractive candidates. The well-defined epitopes and efficient production methods of these entities render them promising candidates for immunotherapeutic applications. However, their efficacy is significantly limited by HLA restriction, as peptides are presented exclusively by specific HLA alleles. Moreover, it is not yet clear whether off-target effects may occur, where T cells that recognize neoantigens could also target related sequences in healthy cells [93, 94].
The principle of DC-based vaccines involves the isolation of DCs from patients and neoantigen loading in vitro neoantigen loading before introducing them to the same patient [95, 96]. DCs are highly potent professional antigen-presenting cells capable of eliciting robust T cell-mediated immune responses [97]. Although DC vaccines provide strong antigen presentation and, broader immune activation, their widespread clinical applications are hindered by complex manufacturing, high costs, and logistical challenges [98].
DNA/RNA vaccines are based on DNA- or RNA-encoding neoantigens. After administration, nucleic acids are translated into proteins, thus allowing the in vivo expression and processing of neoantigens [99]. Furthermore, the high interest in vaccines is based on their fast and scalable production processes and their ability to encode many neoantigens on one vaccine construct. A major challenge is the efficient delivery of nucleic acids to target cells; specialized delivery systems such as nanoparticles or viral vectors are typically required [100]. For DNA vaccines, the safety considerations related to the risk of insertional mutagenesis are controversial. Generally, the following neoantigen delivery platforms can be compared: neoantigen/nucleic acid-based, peptide-based, and DC-based [101].
Neoantigen-specific, peptide-based vaccines offer well-defined epitopes and are easy to synthesize, making them a straightforward approach for targeting neoantigens. However, their fundamental limitations are HLA restriction, which may limit their applicability in different patient cohorts, and off-target toxicity [102]. DCs-based vaccines have strong antigen presenting abilities, leading to a robust immune response and wider immune system activation. But their clinical use is still limited by complex manufacturing processes and costs [13, 95, 97]. As schematically depicted in Figure 2, DC-based immunotherapy utilizes an ex vivo or in vivo approach to activate T cell, aiming to enhance the specificity of the immune response against tumor cells. This strategy is an integral component of modern cellular therapies cancer treatment.
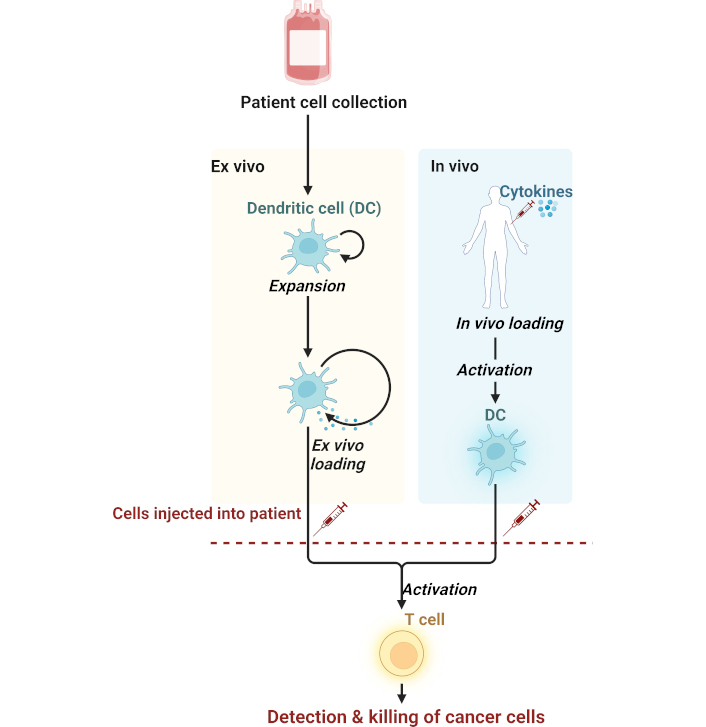
Mechanism of dendritic cell-based immunotherapy against cancer. Cells are derived from a patient and then processed in two ways: (1) Ex vivo loading, where outside the body, the dendritic cells (DCs) are expanded and loaded with antigens before being reinjected into the patient, and (2) In vivo loading, where cytokines act directly on DCs inside the patient to activate them, thus initiating the immune response. T cell activation in both methods will lead to the detection and killing of cancer cells and implicate the use of dendritic cells in adaptive immunity. Created in BioRender. Bashatwah, R. (2025) https://BioRender.com/z60g279
Recent improvements in neoantigen vaccine delivery platforms have focused on leveraging innovative methodologies to enhance both immunogenicity and efficacy [2, 27, 71]. For example, structure-based immunogenicity and antitumor activity in preclinical models. Nanoparticles-based vaccine delivery systems also allow for improving pharmacokinetics of the administered drugs and facilitate co-delivery of antigens and adjuvants also improving immune activation. All these strategies synergistically improve delivery of neoantigens into target cells, enhancing immune responses for tumor-specific neoantigens [103, 104].
Taking this into consideration, although each of these platforms offers distinct advantages and disadvantages, current research efforts are focused on optimizing of delivery systems for maximal immunogenicity and therapeutic benefits in neoantigen vaccines for personalized cancer immunotherapy [27, 49, 105]. By overcoming the limits of each individual platform and combining the advantages of both, a better and more broadly applicable neoantigen vaccine can be developed for use in cancer patients [2, 4, 13, 71]. Table 2 summarizes neoantigen vaccine modalities with their mechanism of action, their advantages, and disadvantages clinically. The table also summarizes current efforts focused on optimizing peptide-based, DC-based, and RNA-based vaccine platforms against melanoma and glioblastoma.
The key characteristics of neoantigen vaccine platforms
Vaccine platform | Mechanism | Advantages | Limitations | Clinical applications |
---|---|---|---|---|
Peptide-based vaccines | Uses synthetic peptides representing tumor-specific neoantigens to activate T cells | Well-defined epitopes; ease of production; high specificity | Limited by HLA restriction; potential off-target effects; lower immunogenicity without adjuvants | Effective in melanoma and glioblastoma; often combined with adjuvants for enhanced efficacy |
Dendritic cell vaccines | Involves loading patient-derived dendritic cells with neoantigens for antigen presentation to T cells | Strong antigen presentation; potent and broad immune responses | High cost; complex manufacturing; logistical challenges | Successfully used in early-phase trials for melanoma and prostate cancer |
DNA-based vaccines | Encodes neoantigens in DNA plasmids for in vivo antigen expression and immune activation | Fast and scalable production; can encode multiple neoantigens | Risk of insertional mutagenesis; requires specialized delivery systems | Preclinical studies in melanoma and colorectal cancer; promising safety profiles |
RNA-based vaccines | Encodes neoantigens in mRNA for transient antigen expression in target cells | High immunogenicity; non-integrative; can encode multiple neoantigens | Limited stability; cold chain storage requirements; complex delivery mechanisms | Successfully used in melanoma clinical trials; synergy with immune checkpoint inhibitors |
Nanoparticle-based vaccines | Uses nanoparticles to deliver neoantigens and adjuvants directly to antigen-presenting cells | Enhanced antigen delivery; co-delivery of adjuvants; improved stability | High development costs; variability in targeting efficiency | Promising results in preclinical studies; under investigation in combination therapies for melanoma and glioblastoma |
Virus-like particle (VLP) vaccines | Presents neoantigens on virus-like particles for effective T cell activation | High immunogenicity; strong antigen presentation; scalable production | Immune response may target carrier particles; limited availability of VLP production platforms | Preclinical success in melanoma and pancreatic cancers; potential for rapid production during clinical trials |
This table summarizes the mechanisms, advantages, limitations, and clinical applications of the main neoantigen vaccine platforms, including peptide-based, dendritic cell (DC)-based, and nanoparticle-based vaccines. These platforms offer distinct approaches to personalized cancer immunotherapy, each with specific benefits and challenges depending on the clinical context. The comparison highlights how neoantigen vaccine platforms can advance cancer therapy, particularly in melanoma and glioblastoma, by improving vaccine design and overcoming existing limitations to enhance therapeutic efficacy
Immunological adjuvants
Adjuvants actively increase neoantigen vaccine immunogenicity by enhancing innate and adaptive immunity [14]. By stimulating the innate immune response, adjuvants activate mature APCs, including DCs, which present neoantigenic peptides to T cells and ultimately elicit a strong adaptive immune response [106]. The application of adjuvants improves the efficiency of neoantigen vaccines through multiple mechanisms [106].
Improved antigen presentation: Adjuvants stimulate APCs in the uptake and processing of antigens more effectively to increase the presentation of neoantigens to T cells [106].
Enhancement of immune cell activation: In addition, adjuvants are used to activate immune cells, such as DCs and macrophages, which, in turn, activate T cells for a more effective response [106–108].
Modulation of the immune response: Adjuvants may modulate the balance between pro- and anti-inflammatory cytokines, thus further contributing to minimizing side effects [106, 109].
Promising adjuvant candidates have demonstrated promising results in enhancing neoantigen vaccine immunogenicity. Adjuvant use is widespread, especially for those that engage Toll-like receptors including several molecules, including poly-ICLC. These factors have been associated with enhanced neoantigen vaccine immunogenicity in numerous clinical trials [109].
STING agonists: STING agonists trigger the STING pathway, elicit innate immune responses, and enhance immunogenicity. The combination of STING agonists with other therapies is possible [110, 111].
Cytokine-based adjuvants: These include many cytokines, including IL-12 and IL-15, which have been shown to activate T cells and natural killer cells and enhance neoantigen vaccine activity in preclinical models [112, 113].
Combination with other immunotherapies: Several adjuvant systems can also collaborate with other immunotherapies to enhance the overall therapeutic activity of neoantigen vaccines [114, 115].
Combination with immune checkpoint blockade: Neoantigen vaccine immunogenicity enhanced through adjuvant therapy may be further augmented by the addition of immune checkpoint inhibitors. In this vein, the combination of adjuvants with checkpoint inhibitors, such as anti-PD-1 therapy, has thus far shown tremendous promise in enhancing immune responses across a wide variety of malignancies, including melanoma and renal cell carcinoma [116].
Combination of chemotherapy and radiation therapy: Adjuvants can also improve neoantigen vaccine immunogenicity upon administration in combination with chemotherapy and radiation therapy, thereby enhancing cancer disease outcomes [117].
The improvement of vaccine immunogenicity using immunological adjuvants is one of the most critical vaccine development strategies [17, 24, 44, 100]. Adjuvants are substances that enhance host immune responses, including cytokines, agonists of TLRs, and host defense peptides. Several studies have underlined the potential of using agonists of costimulatory molecules and modulating pre-existing inflammation to improve vaccine responsiveness. Knowledge of the molecular mechanisms of adjuvant action, such as their interaction with TLR4 receptors, is an important issue in formulating potent and safe vaccine compositions [9, 15, 17, 100].
The molecular and cellular mechanisms triggered by TLR4-targeted combination adjuvants have been studied [24, 44]. For example, the importance of understanding DC cross-presentation in the quest for effective vaccines has been stressed because adjuvants modulate DC cross-presentation toward balanced antibody and T cell-based immunity induction [8, 96, 98]. The combination of adjuvants that target cross-presenting pathways with innate immune signaling is expected to give rise to powerful cytotoxic T lymphocyte (CTL) immunity and long-lasting CTL memory [44, 65, 76, 102].
In summary, adjuvants play a fundamental role in enhancing neoantigen vaccine immunogenicity by activating the innate immune pathways and inducing an adaptive immune response. The most promising adjuvant candidates are TLR agonist-based, STING agonists, and cytokine-based adjuvants [110, 111]. The combination of adjuvants with immune checkpoint inhibitors and other immunotherapies has great potential for synergistic effects, ensuring better therapeutic outcomes in patients with cancer. Ongoing efforts focus on optimizing the conditions of adjuvant formulations to maximize neoantigen vaccine efficacy and promote their clinical use in personalized cancer immunotherapy.
Neoantigen vaccines, in concert with immune checkpoint inhibitors, represent one of the most effective strategies in cancer immunotherapies to maximize synergistic effects for improved therapeutic outcomes [116]. Several studies have emphasized the synergy between neoantigen vaccines and immune checkpoint inhibitors, including PD-1/PD-L1 inhibitors, in the generation and maintenance of neoantigen-specific immune responses [116]. The blockade of immune checkpoints is expected to act synergistically with both cell-mediated and vaccine-induced neoantigen-specific immune responses [118].
A combination of neoantigen vaccination with immune checkpoint inhibitors has shown promising clinical efficacy in patients with cancer. This combination treatment is expected to enhance the general efficacy of cancer immunotherapy by targeting patient-specific neoantigens, thereby improving the antitumor immune response. Preclinical and clinical investigations have reported encouraging results for the combination of a neoantigen vaccine with immune checkpoint inhibitors in melanoma, lung cancer, and gastrointestinal cancers. Neoantigen vaccines when used alongside immune checkpoint inhibitors, are being actively investigated in personalized cancer immunotherapy to enhance both treatment specificity and efficacy.
Mechanisms of synergy
Perhaps combining neoantigen vaccines with strategies targeting different levels of the immune response will be the future of treatment [33, 45, 66]. Neoantigen vaccines can elicit strong, specific immune responses against tumor-specific antigens, whereas checkpoint inhibitors remove the breaks in the immune system and further potentiate the attack against cancerous cells [16, 41, 49]. This approach could overcome some of the mechanisms of resistance in cancer therapy and thus improve patient outcomes. Several studies have reported adjuvant use in combination with immune checkpoint inhibitors, such as anti-PD-1 therapy, to improve immune response and clinical outcomes in various malignancies [119].
Several such combinations have shown promise for non-small cell lung carcinoma, urothelial carcinoma, head and neck, gastric, esophageal, triple-negative breast, and pancreatic malignancies [120]. The proposed mechanisms of synergy include immunogenic tumor cell death, anti-angiogenesis, selective depletion of myeloid-derived immunosuppressive cells, and lymphopenia, which leads to the proliferation of effector T cells [40, 44, 48]. Although clinical benefits have been observed with some of these combinations, further studies are needed to optimize treatment strategies and to identify better predictors of response to immune checkpoint inhibitors [27, 116, 119].
Neoantigen vaccines combined with immune checkpoint inhibitors are emerging as promising new modalities for cancer immunotherapy [32, 114]. Preclinical and translational studies are underway using combinations of neoantigen vaccines with immune checkpoint inhibitors to further enhance antitumor immune responses against tumor-specific antigens, potentially providing better therapeutic benefits and more personalized treatment options for patients with cancer [10, 40]. Optimizing neoantigen vaccine-based combination optimization and expanding clinical translation across tumor types should further improve outcomes in patients with cancer.
Clinical trials and emerging data
The translation of neoantigen vaccines from preclinical studies to clinical testing has progressed rapidly, and encouraging results have been reported in personalized cancer therapy. This section discusses key findings from early phase and ongoing clinical trials evaluating the safety, immunogenicity, and clinical outcomes of neoantigen vaccines in melanoma [17, 121].
The first wave of phase I/II clinical trials, designed mainly to assess safety and proof-of-concept, has yielded promising results, especially in patients with advanced-stage melanoma that has already progressed despite standard treatment [122].
Safety and tolerability
Neoantigen vaccines are generally well-tolerated. The most common adverse events are usually mild-to-moderate grade and include injection-site reactions, fatigue, and flu-like symptoms, which contrast with the often serious toxicities of conventional chemotherapy or targeted therapies [17].
Immunogenicity
Neoantigen vaccines demonstrate robust immune responses against tumor-specific neoantigens [2]. This is largely supported by neoantigen-specific T cell responses often measured in phase I/II trials using techniques such as enzyme-linked immunospot (ELISpot), intracellular cytokine staining, and tetramer staining [123]. Moreover, the magnitude of T cell responses has been observed to parallel clinical outcomes in a manner that strongly suggested that neoantigen-specific responses play a role in antitumor activity [44, 45].
Although early-phase trials are not powered to definitively assess clinical efficacy, they have reported encouraging results [9, 27, 121]. Findings include prolonged progression-free survival (PFS), improved overall survival (OS), and complete tumor regression. For instance, a phase I trial combining a personalized neoantigen vaccine with anti-PD-1 therapy in advanced melanoma reported a 6-month PFS rate of 62% and an OS rate of 80% [123, 124].
Successful neoantigen vaccines depend on careful patient selection, optimized treatment regimens, and identification of predictive biomarkers. Patients with a high tumor mutational burden (TMB) and a favorable HLA genotype are more likely to benefit [51]. Several biomarkers are being explored for their potential to predict vaccine response, including tumor mutation burden, neoantigen clonality, and pre-existing T cell responses against neoantigens. A trial was initiated to improve neoantigen-based anticipation of a response to immune checkpoint blockade (ICB) therapy in melanoma patients by integrating neoantigen burden with immune-related resistance mechanisms [22, 125].
Tumors from patients with late-stage melanoma were comprehensively analyzed using an immune-enhanced exome and transcriptome platform to develop a predictive model. The neoantigen burden score, which integrates both exome and transcriptome features, effectively stratified patients into responders and non-responders, demonstrating superior predictive performance compared to TMB alone [126]. By incorporating immune-related resistance mechanisms, such as HLA allele-specific LOH, researchers have devised a composite neoantigen presentation score (NEOPS), which shows a strong correlation with therapy response [126]. NEOPS has emerged as a more reliable biomarker compared to single-gene biomarkers and expression signatures, positioning it as a novel predictor of ICB response in melanoma. Its validation in an independent patient cohort further reinforced its robustness and reliability as a predictive biomarker for ICB therapy response [126].
Building on the promising results of early phase trials, several phase III clinical trials are underway to rigorously assess the efficacy of neoantigen vaccines in melanoma by directly comparing them with standard-of-care therapies. Examples of ongoing phase III trials include [127]. NCT04990479, which evaluates the combination of pembrolizumab and a personalized neoantigen vaccine (NOUS-PEV) in patients with metastatic melanoma [128, 129].
The Nous-PEV vaccine is specifically designed to target patient-specific neoantigens, aiming to enhance the immune response against melanoma cells [130]. Pembrolizumab, an immune checkpoint inhibitor targeting PD-1, is administered in combination with neoantigen vaccines to improve the antitumor immune response and optimize clinical outcomes in patients with metastatic melanoma [131]. Studies have demonstrated that the development of a personalized vaccine, NOUS-PEV, incorporates 60 patient-specific neoantigens [130]. Currently, this is being investigated in a clinical trial involving patients with metastatic melanoma and non-small cell lung cancer in combination with pembrolizumab. This study aims to evaluate the safety, immunogenicity, and efficacy of this neoantigen vaccine in enhancing the antitumor immune response in patients with advanced tumors [132].
The combination of pembrolizumab with a neoantigen vaccine represents a promising approach in cancer immunotherapy, where, the synergy between immune checkpoint inhibition and neoantigen specificity enhances tumor elimination. These personalized strategies have the potential to improve patient outcomes and advance precision medical strategies for metastatic melanoma [132, 133].
This trial highlights the rising interest in neoantigen-targeting personalized cancer vaccines and the potential synergistic benefits of their combination with immune checkpoint inhibitors in the enhancement of immune responses against cancer cells. The results of the trial will be crucial for understanding the efficacy of neoantigen vaccines when used in combination with checkpoint inhibitors and their interaction with clinical outcomes in patients with metastatic melanoma [134].
The clinical trial investigating the efficacy of the EVX-01 vaccine in patients with advanced melanoma compared pembrolizumab alone [134, 135]. This study (NCT05309421) evaluated the efficacy of the neoepitope vaccine EVX-01 in patients with advanced melanoma relative to pembrolizumab alone. EVX-01 is a personalized neoepitope vaccine that targets neoantigens in patients, whereas pembrolizumab is an immune checkpoint inhibitor that targets the PD-1 receptor. This trial assessed the safety, immunogenicity, and efficacy of the EVX-01 vaccine in combination with pembrolizumab in patients with metastatic melanoma [136].
The KEYNOTE-D36 trial: personalized immunotherapy with the EVX-01 neoepitope vaccine and pembrolizumab in patients with advanced melanoma. In this study, the efficacy and safety of EVX-01 neoepitope vaccine combined with pembrolizumab were tested in patients with metastatic or unresectable melanoma [137]. The trial aims to evaluate clinical outcomes and immune responses generated by the EVX-01 vaccine in combination with pembrolizumab will follow.
Personalized immunotherapy against metastatic melanoma was conducted using the neoantigen peptide-based vaccine EVX-01 coupled with the adjuvant CAF®09b in five patients. Neoantigens were precisely identified by analyzing blood and tumor samples using the PIONEERTM AI-powered platform. The vaccine consisted of six infusions containing 5–10 predicted neoantigens as synthetic peptides. Interim analysis confirmed the safety profile of EVX-01 in eliciting long-lasting, neoantigen-specific, T cell responses targeting tumor-associated neoantigens [138].
In addition, early signs of clinical efficacy were observed, indicating the potential of EVX-01 as one of the most promising strategies for further investigation and advancement of personalized immunotherapy for metastatic melanoma [138]. Further attention has been directed toward the development of the next-generation neoantigen vaccine-based personalized therapy, EVX-02, includes a novel adjuvant, CAF®09b, developed for patients with melanoma [1]. Neoantigen selection for EVX-02 was performed using the AI-driven platform called PIONEER platform, enabling the development of targeted neoantigen vaccine in melanoma.
Thus, EVX-02 has demonstrated safety in patients with resected melanoma, eliciting only low-grade side effects while inducing neoantigen-specific immune responses driven by activated CD4+ and CD8+ T cells [139]. More importantly, this underscores the accuracy and prognostic potential of the PIONEER platform, as DNA-encoded neoantigen delivered via gene-based technology induce strong neoantigen-specific immune responses [140]. Collectively, these findings highlight the promise of neoantigen vaccines as therapeutic modalities in cancer immunotherapy. The results demonstrated the accuracy and efficiency of the PIONEER platform in predicting neoantigens capable of inducing potent immune responses, reinforcing the feasibility of personalized neoantigen vaccines in cancer immunotherapy [10].
Another published research [10] described a novel platform for neoantigen identification. The combination of the EVX-01 vaccine and pembrolizumab represents an unusual approach in cancer immunotherapy because it leverages the specificity of neoantigens and the immune-modulating effects of checkpoint inhibitors to augment antitumor immune responses [10]. The results of this trial may give insight into the neoepitope vaccine efficacy, personalized in combination with immune checkpoint inhibitors, and associated clinical outcomes of patients with advanced melanoma [137]. These are generally randomized controlled trials (RCTs) with primary endpoints of OS, progression-free survival, and objective response rates. These trials are intended to assess whether neoantigen vaccines confer long-term clinical benefits that will eventually define their position in (clinical) practice [87, 88, 140].
Case studies in personalized medicine
Compelling case studies involving individual patients have further accelerated the development of neoantigen vaccines [16, 102, 132]. Two notable examples demonstrate the transformative potential of personalized immunotherapy in treating melanoma. The first case involved a patient with stage IIIB/C melanoma who was treated with NeoVax vaccine [16, 141]. The patient achieved a complete response within 25 months and showed no signs of tumor progression at four years. The second case describes a patient with advanced-stage melanoma who had been treated with EVX-01 vaccine [16, 138]. The patient also achieved a complete response within 12 months and was free of tumor progression at two years. These two cases highlight the potential of neoantigen vaccines to induce durable responses, further emphasizing that the personalization is a key factor in treatment success [8, 135].
These cases further illustrate the impact of personalized immunotherapy, in which treatment is tailored to the unique tumor profile of each patient. Neoantigen vaccines exploit neoantigen specificity and develop individualized treatment strategies, highlighting a promising path forward in personalized cancer immunotherapy [135, 142].
Early-phase clinical trials, ongoing phase III trials, and compelling case studies collectively underscore the transformative potential of neoantigen vaccines in melanoma treatment. These studies provide important insights into safety, immunogenicity, and clinical outcome outcomes associated with from neoantigen vaccines, thereby paving the way for personalized, effective immunotherapeutic strategies in cancer care [143].
Mechanisms of antitumor responses induced by neoantigens
The efficacy of neoantigen-based cancer immunotherapy relies on a series of immunological events that trigger potent antitumor activity [22]. Following vaccination, APCs, particularly DCs, engulf and process neoantigens, and subsequently present them via MHC molecules to naïve CD8+ and CD4+ T cells. This activation leads to the expansion of tumor-specific CTLs, which recognize and eliminate neoantigen-presenting tumor cells [144].
The effectiveness of neoantigen vaccines relies on several variables, including antigen presentation quality, T cell priming efficiency, and longevity of T cell memory responses. Strong CD8+ T cell activation is required for the direct killing of tumor cells, while CD4+ helper T cells play an important role in providing cytokine support to sustain the cytotoxic response. Additionally, neoantigens with high MHC-binding affinity and low sequence homology to self-proteins elicit stronger intense immune responses, with a lower probability of immune tolerance [2, 4].
Optimal responses also necessitate overcoming the immunosuppressive tumor microenvironment. The immunosuppressive activities of regulatory T cells (Tregs), myeloid-derived suppressor cells (MDSCs), and inhibitory cytokines can diminish vaccine-induced immune responses [88]. Strategies that combine neoantigen vaccines with immune checkpoint inhibitors (e.g., anti-PD-1 and anti-CTLA-4 antibodies) help restore T cell function by blocking suppressive signaling pathways. Furthermore, adjuvants, including poly-ICLC, CpG oligodeoxynucleotides, and STING agonists, enhance vaccine activity by inducing innate immune activation and boosting DC function [116, 145].
The combination of personalized vaccine strategies that consider tumor heterogeneity and clonal evolution is the cornerstone for ensuring long-term immunity [146]. Multi-epitope neoantigen vaccines targeting multiple mutations within a tumor increase the likelihood preventing immune-evasion mechanisms. In addition, optimizing vaccine delivery systems, such as mRNA-based systems, nanoparticle carriers, and DC-based vaccines, can increase antigen stability and presentation, ultimately leading to enhanced therapeutic outcomes. A deeper understanding of these mechanisms is critical for optimizing neoantigen vaccine approaches and develping more potent and persistent cancer immunotherapies [87, 147, 148].
Challenges and future directions
Neoantigen vaccines demonstrate great promise in personalized cancer therapy; however, these vaccines must overcome several technical, biological, and regulatory challenges before their full potential can be realized [1].
Technical challenges
Further refinement of neoantigen prediction algorithms is needed. Currently, neoantigen algorithms have low power for predicting immunogenicity, resulting in high false-positive and false-negative rates are high. To improve predictive accuracy, advancements in algorithms are required to integrate machine learning with multi-omics data. In addition, manufacturing needs to be harmonized across processes to produce vaccines that are consistent, scalable, and affordable [76]. Automation in production and collaboration with industrial partners can facilitate streamlining and economic growth. Optimized neoantigen selection can be achieved by prioritizing highly immunogenic neoantigens, enhancing antigen presentation, and improving T cell trafficking through state-of-the-art bioinformatics and novel delivery platforms [22].
Biological challenges
The heterogeneity of the tumor and its clonal evolution require multi-epitope approach and adaptive vaccine designs that consider immune escape mechanisms for further improvement in efficacy [81]. Several combination strategies have been developed to overcome immunosuppressive conditions within the tumor microenvironment, including neoantigen vaccination in combination with checkpoint blockade and cell therapy, which remains one of the most promising approaches [149]. To establish long-lasting immunological memory, the factors affecting the generation and maintenance of memory T cells need to be understood; hence, studies on specific adjuvants and cytokines are essential to induce durable protection [150].
Ethical considerations
From a fundamental viewpoint, access to personalized therapies such as neoantigen vaccines is crucial to reducing disparities in cancer care. The industry must take proactive measures to ensure that it is accessible to all patients [9]. Informed consent must be sought in advance and privacy must be safeguarded through appropriate data governance structures. Long-term safety monitoring is essential to address potential off-target effects such as autoimmune reactions and late-onset toxicities. Therefore, continuous surveillance and evaluation should be performed.
Off-target effects and long-term safety concerns
However, neoantigen vaccine development presents major challenges related to the management of off-target effects and long-term safety [2, 87, 130, 132]. In this context, monitoring autoimmune reactions with stringent protocols can help detect immune responses against unintentionally targeted non-neoplastic tissues long-term toxicity monitoring will help assess toxicities that have adverse effects that may develop over time. Overall, risk-benefit analyses derived from these studies will continue to provide critical insights into overall safety and therapeutic benefits, including evaluating the risk versus benefit to the patient [151].
Conclusions
Neoantigen vaccines represent a new frontier in personalized cancer therapy and offer promising treatment options with unprecedented specificity and potency tailored to the unique neoantigenic mutations of each tumor. These vaccines harness tumor-specific neoantigens and allow the induction of a potent, specific, and targeted immune response, thereby increasing the possibility of durable clinical outcomes.
Neoantigen vaccines depend critically on the neoantigen prediction algorithm. Although current models, although improved, still have difficulties in correctly predicting immunogenic neoantigens due to persistent false positives and false negatives. To enhance feasibility, the manufacturing processes must focus on homogeneity, scalability, and cost-effective production. Additionally, antigen presentation and T cell homing in the tumor microenvironment should be optimized to improve vaccine efficacy. However, tumor heterogeneity and clonal evolution remain significant barriers. To address these challenges, multi-epitope targeting and adaptive vaccine design are essential. The immunosuppressive nature of the tumor microenvironment complicates its efficacy; therefore, combination therapies with checkpoint inhibitors or adoptive cell therapies are necessary. Furthermore, long-term immunological memory for long-lasting antitumor responses. Achieving this requires a deeper understanding of the mechanisms governing memory T cell generation and persistence.
Future studies should focus on enhancing neoantigen prediction algorithms by incorporating multi-omics integration and machine learning techniques. The broader application of neoantigen vaccination relies on the development of standardized manufacturing processes. Further research should explore nanoparticle-based platforms and viral vectors, which may improve delivery systems and enhance antigen presentation alongside T cell activation. Innovative approaches are required to overcome tumor heterogeneity and the immunosuppressive microenvironment, including adaptive vaccine design and combination therapy. Moreover, the molecular mechanisms governing long-term immunological memory must be elucidated to develop vaccines that can induce long-lasting protection against tumor recurrence.
Neoantigen vaccines have the potential to transform cancer treatment; therefore, more research on their potential is highly warranted. This progress should be supported by enhanced collaboration among academia, clinicians, and industrialists, together with more investment in the field of personalized immunotherapy. By addressing technical, biological, and ethical challenges faced, neoantigen vaccines can transition from experimental therapies to mainstream clinical practice, ultimately improving outcomes for cancer patients can be improved worldwide.
Many challenges persist, even as advancements continue; neoantigen vaccines have great potential for reshape cancer therapy. Further innovation, research collaboration, and adherence to ethical standards will be essential to fully harness these personalized therapies and realize their potential for efficient and durable treatment. This review underscores the progress in neoantigen vaccine development and the need for further research, collaboration, and technological advancements to overcome current challenges and optimize their clinical application in personalized cancer immunotherapy.
Abbreviations
APC: | antigen-presenting cell |
CDK4: | cyclin-dependent kinase 4 |
CTL: | cytotoxic T lymphocyte |
DC: | dendritic cell |
ICB: | immune checkpoint blockade |
LOH: | loss of heterozygosity |
NEOPS: | neoantigen presentation score |
NGS: | next-generation sequencing |
NSCLC: | non-small-cell lung cancer |
OS: | overall survival |
PFS: | progression-free survival |
TAAs: | tumor-associated antigens |
TCR: | T cell receptor |
TMB: | tumor mutational burden |
Declarations
Author contributions
AAAA: Conceptualization, Writing—original draft, Investigation, Writing—review & editing. YH: Investigation, Writing—original draft. AA and LA: Investigation, Writing—review & editing. All authors have read and agreed to the published version of the manuscript.
Conflicts of interest
The authors declare that they have no conflicts of interest.
Ethical approval
Not applicable.
Consent to participate
Not applicable.
Consent to publication
Not applicable.
Availability of data and materials
Not applicable.
Funding
Not applicable.
Copyright
© The Author(s) 2025.
Publisher’s note
Open Exploration maintains a neutral stance on jurisdictional claims in published institutional affiliations and maps. All opinions expressed in this article are the personal views of the author(s) and do not represent the stance of the editorial team or the publisher.