Abstract
Aim:
Previously, we reported increased number of T helper 17 (Th17) cells in vitiligo. However, in our recent study, tryptase and interleukin (IL)17 double positive cells which identified by polyclonal anti-IL17 antibody with specificity for IL17A, B, D, F was observed, but these mast cells cannot be stained by monoclonal anti-IL17 antibody with specificity for IL17A. Therefore, this study was aimed to clarify the role of mast cells in induction and progression of vitiligo.
Methods:
Mast cells were stained with two antibodies against IL17 and one antibody against tryptase by immunofluorescent staining. Furthermore, immunoelectron microscopy (IEM) analyses were conducted using anti-tryptase. In vitro, cultured epidermal keratinocytes were treated with agents which released by mast cells. Expression levels of mRNA were analyzed by real-time polymerase chain reaction (PCR), expression of protein levels was analyzed by western blotting.
Results:
An increased number of tryptase positive mast cells was observed at the lesional skin of upper dermis in vitiligo and rhododendrol-induced leukoderma (RDIL). These mast cells showed prominent degranulation in vitiligo. Interestingly, the melanosome forming glycoprotein non-metastatic melanoma protein B (GPNMB) is downregulated in the lesional basal keratinocytes in vitiligo and mast cell tryptase contributes to this phenomenon. In addition, small interfering GPNMB RNA (siGPNMB RNA)-introduced keratinocytes increased melanocyte survival through stem cell factor (SCF) production in the melanocyte/keratinocyte co-culture system.
Conclusions:
Mast cells might be two-faced in vitiligo induction, progression, and recovery through the differential function of histamine and tryptase.
Keywords
Vitiligo, rhododendrol-induced leukoderma, mast cell, tryptase, interleukin 17Introduction
Vitiligo vulgaris is common depigmented skin disorder characterized with loss of functional melanocytes. It is a multifactorial disorder with a complex pathogenesis. The pathogenesis theories include oxidative stress, neurotoxic effect, CD8+ cytotoxic T cells and autoantibodies against melanocyte. Epidermal cytokine imbalance was recently known to play an important role in the induction of melanocytes’ dysfunction and hypomelanotic lesions [1, 2]. Inflammatory cytokine expression which are reported increased in vitiligo, such as that of interleukin (IL)6, tumor necrosis factor α (TNF-α), interferon-γ (IFN-γ), or IL2, and reduced endothelin-1 (ET-1) or stem cell factor (SCF) expression exhibit melanocyte-stimulating activity [3, 4].
It is well known that hypermelanosis is observed in the surrounding skin of vitiligo and drug-induced photo-leukoderma, similar clinical features are also observed in cosmetic-induced rhododendrol-induced leukoderma (RDIL) from Japan [5–7].
In a previous study, Tomita et al. [8] reported that histamine stimulates melanogenesis in vitro and contributes to ultraviolet-B (UVB) induced hypermelanosis through the H2 histamine receptor. We have previously reported that IL17A-producing T helper 17 (Th17) cells infiltrated to the lesional skin in vitiligo and IL17A stimulated fibroblast- and keratinocyte-generated proinflammatory cytokines, such as TNF-α, IL1, or IL6. Furthermore, all of these cytokines downregulated micropthalmia-associated transcription factor (MITF) activation resulting in promoting the depigmentation process in vitiligo [9]. More recently, IL17 positive mast cells were found at higher densities than IL17-positive T cells in psoriasis [10], which prompted us to analyze IL17-positive infiltrating cells in the lesional skin of vitiligo.
Cosmetically, hypermelanosis of the perilesional skin in leukoderma is an important problem to be resolved as the patients are instructed not to use skin whitening for these hypermelanotic lesions [6]. Therefore, in this study, we focused on IL17 positive cells and mast cells in vitiligo.
Materials and methods
Patients
The following participants have been recruited in this study: patients with vitiligo (n = 7, of which 2 and 5 were males and females, respectively, aged 60.1 ± 4.0 years; skin biopsies including 1 from neck, 2 from arm and 4 from abdomen); patients with RDIL (n = 5, of which all patients were females, aged 56.8 ± 8.1 years; skin biopsies including 1 from forehead, 1 from chin, 1 from arm and 1 from abdomen); healthy participants as a control (n = 5, of which 2 and 3 were males and females, respectively, aged 69.2 ± 4.3 years; skin biopsies including 2 from eyelid, 1 from abdomen and 2 from arm).
Human skin specimens
Skin samples were fixed in 10% formaldehyde for paraffin embedding. Paraffin-embedded tissue sections (5 μm) of the lesional skin samples from healthy controls and patients with vitiligo or RDIL were used in this study. Written informed consent was obtained from all the participants prior to their inclusion in the study. The study was approved by the ethics committee of the Osaka City University Faculty of Medicine (No.10339).
Reagents
Anti-IL17 polyclonal antibody (AF-317-NA, R&D Systems, Minnesota, USA; specificity: IL17A, B, D, F); Anti-IL17A monoclonal antibody (AHP455G; Bio-Rad Laboratories, Hercules, CA, USA; specificity: IL17A); anti-mast cell tryptase monoclonal antibody (ab134932; Abcam, Cambridge, UK); FITC-AffiniPure Donkey Anti-Rabbit IgG (H+L) antibody (711-095-152, Invitrogen, Carlsbad, CA, USA); Anti-Pmel17 antibody (sc-377325, Santa Cruz Biotechnology, Dallas, TX, USA); anti-SCF antibody (ab52603, Abcam, Cambridge, UK); anti-GPNMB antibody (AF2550; R&D Systems, MN, USA); Alexa Fluor® 555 Phalloidin (#8953, Cell Signaling Technology, Beverly) have been used in this study.
Quantitative real-time polymerase chain reaction analysis
Total RNA was extracted from cultured cells using the Maxwell® 16 LEV simplyRNA Tissue Kit (Promega, Netherlands) following the manufacturer’s instructions. Total RNA (100 ng) was reverse-transcribed using the ReverTra Ace qPCR RT Master Mix (TOYOBO, Japan). The primers used for real-time polymerase chain reaction (PCR) were as follows: human SCF (hSCF), sense 5-TCAAGGACTTTGTAGTGGCATCTG-3′, antisense 5-CTGCTACTGCTGTCATTCCTAAGG-3′; human glycoprotein non-metastatic melanoma protein B (hGPNMB), sense 5-GCGAGATCACCCAGAACACA-3′, antisense 5-AGAGCCAGGCTTGTGTCATC-3′; human glyceraldehyde-3-phosphate dehydrogenase (hGAPDH), sense 5-GACAGTCAGCCGCATCTTCT-3′, antisense 5-GCGCCCAATACGACCAAATC-3′. The real-time PCR was performed using an ABI PRISM 7900H Sequence Detection System (Applied Biosystems, CA, USA). The reactions were performed in triplicates in three independent experimental sets. The geometric mean of the housekeeping gene GAPDH was used as an internal control to normalize the expression level variabilities.
Fluorescent immunohistochemical staining
Skin tissue samples were fixed in 10 % formaldehyde and embedded in paraffin. Next, 5 μm sections were used for the fluorescent immunohistochemical staining. The sections were incubated with primary antibodies specific for human mast cell tryptase (Anti-Mast Cell Tryptase rabbit monoclonal antibody; dilution, 1:10), incubated at 4°C overnight, washed with phosphate buffered saline with Tween 20 (PBS-T) trice (5 min each time), then incubated with anti-Rabbit IgG (H+L) Goat polyclonal Alexa Fluor® 488 (dilution, 1:500) or anti-Mouse IgG (H+L) Donkey polyclonal Rhodamine Red-X (dilution, 1:500) antibodies at room temperature for 30 min, washed with PBS trice (5 min each time), and mounted. The anti IL17A antibodies were used similarly, except for the secondary antibodies.
Human pre-embedding immuno-electron microscopy
Pre-embedding immuno-electron microscopy was performed as described previously [11], with slight modifications. Briefly, finely minced pieces of skin tissue were fixed in 4% paraformaldehyde for 2 h at room temperature and embedded in optimal cutting temperature compound. Frozen samples were cut into 10 μm sections and permeabilized in 0.25% saponin-containing phosphate buffer (PB) for 30 min. the sections were blocked for 30 min in 0.1% saponin-, 10% bovine serum albumin-, 10% normal goat serum-, and 0.1% cold water fish skin gelatin-containing PB and incubated overnight with rabbit anti-mast cell tryptase primary antibodies (ab134932; Abcam) in the blocking solution. The specimens were then incubated with colloidal gold-conjugated goat anti-rabbit IgG antibody (1.4 nm diameter, #2003, Nanoprobes, Yaphank, NY, USA) in the blocking solution for 2 h and the signal was intensified using the GoldEnhanceTM EM kit (#2113, Nanoprobes, Yaphank, NY, USA) for 4 min at room temperature. The specimens were post-fixed in 1% OsO4 containing 1.5% of potassium ferrocyanide, dehydrated in a series of graded ethanol solutions, and embedded in epoxy resin. Ultrathin sections were collected and stained with uranyl acetate and lead citrate, then images were acquired with a Hitachi H7650 electron microscope equipped with an advanced microscopy technique charge-coupled device-based camera system.
Western blotting
For protein sample preparation, cell pellets were extracted as described previously [12] and 5–10 µg of the extracted protein was used for western blotting. The applied primary antibodies were as follows: anti-SCF antibody (ab52603, Abcam) at 1:1000, anti-GPNMB antibody (HPA04496, Sigma-Aldrich, St. Louis, MO, USA), and anti-GAPDH antibody (#2118, CST) at 1:1000. The anti-GAPDH antibody was used as a loading control.
Statistical analysis
The experiments were repeated at least trice. Data are presented as the mean ± SD. The statistical analysis was conducted using two-way analysis of variance for the interactions between variances and Tukey’s or Bonferroni’s post hoc tests. Unpaired student’s t-test (Microsoft Excel; Microsoft Corp., Redmond, WA, USA) was used for the comparison between groups. Statistical significance was set at P < 0.05.
Results
The number of IL17+ cells increased in vitiligo based on an anti-IL17A polyclonal antibody labeling (AF-317-NA; specificity: IL17A, B, D, F) from a study in 2012 [9], in which the authors might have stained extracellular traps, mast cell extracellular trap (MET+) cells, as reported [10]. The percentage of IL17A+ cells increased in the lesional skin in vitiligo and RDIL (Figure 1A and B). The number of tryptase-positive mast cells increased in the lesional skin of the upper dermis in vitiligo and RDIL (Figure 1A and B). Tryptase and IL17 double-positive cells were identified by the polyclonal anti-IL17 antibody, which recognizes IL17A, B, D, F (Figure 1A). However, mast cells were not identified by the monoclonal anti IL17A (AHP455G; specificity IL17A) antibody (Figure 1B and C), these results suggest that these mast cells might contain the non-A subtype of IL17 and MET [10] might occur in vitiligo, similar to those observed in psoriasis or rheumatoid arthritis and provides one possible explanation for the increase in IL17+ cell infiltration into the lesional skin vitiligo [9].
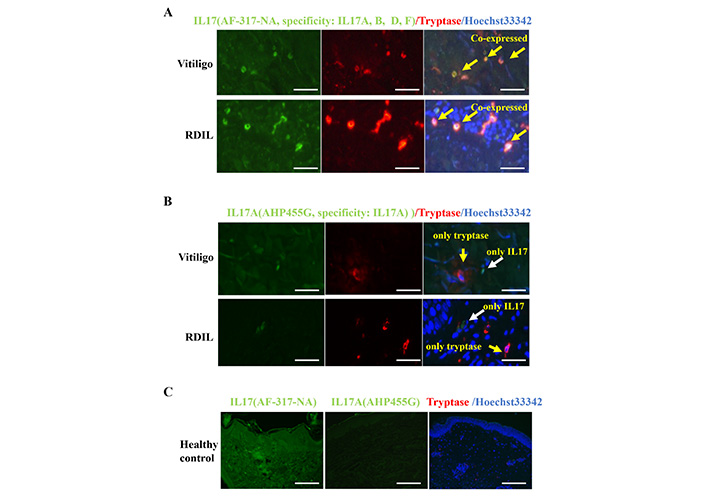
A polyclonal, but not monoclonal, anti-IL17 antibody dose stains tryptase-positive mast cells. Skin sections from vitiligo and RDIL were stained with anti-IL17 polyclonal antibody (AF-317-NA; specificity: IL17A, B, D, F) (A) and the monoclonal anti IL17A (AHP455G; specificity: IL17A (B) in green, mast cells were stained with anti-tryptase in red, cell nuclei were stained in blue with Hoechst33342. Consecutive skin sections from healthy control were stained with polyclonal IL17, monoclonal IL17A and tryptase antibodies (C). Scale bar: 100 μm
The increased mast cell numbers showed prominent degranulation (Figure 2A and B) and possibly secreted histamine, which might be responsible for the transient hypermelanosis of the peri-lesional skin in vitiligo, as previously reported by Yoshida et al [8]. Similar mast cell infiltration increase was observed in RDIL as in vitiligo (Figure 2B). Therefore, persistent hypermelanosis might be induced by mast cell-derived histamine in response to the overexpression of SCF by the keratinocytes or fibroblasts in the dermis.
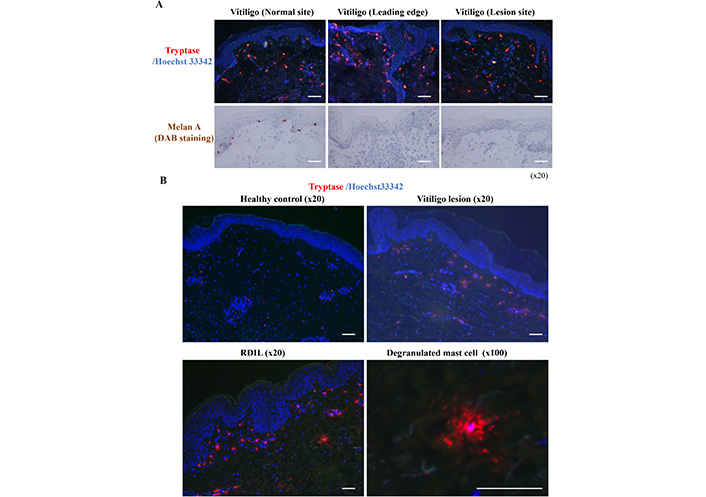
Degranulated mast cell numbers are increased in vitiligo and RDIL. Skin biopsies were taken from the edge of vitiligo lesion, and then cut into three portions including normal site, leading edge, and lesion site. These skin sections were stained with anti-tryptase in red, and consecutive sections were stained with anti-Melan A by 3,3’-Diaminobenzidine (DAB) staining (A). After staining with anti-tryptase in red, the degranulation of mast cells was examined in skin sections of healthy control, vitiligo and RDIL (B). Scale bar: 100 μm
It has already been reported that cosmetic whiten reagent-induced leukoderma and hypermelanosis around the perilesional skin significantly impaired the daily quality of life (QOL) of the RDIL patients [6]. Using skin sections from vitiligo and RDIL, after toluidine blue staining (Figure 3C), mast cells were counted (Figure 3A) and the degranulation rate was calculated (Figure 3B). It was found that degranulated mast cell numbers were increased both in vitiligo and RDIL (Figure 3A-C). It suggests increased mast cell activation in vitiligo and RDIL.
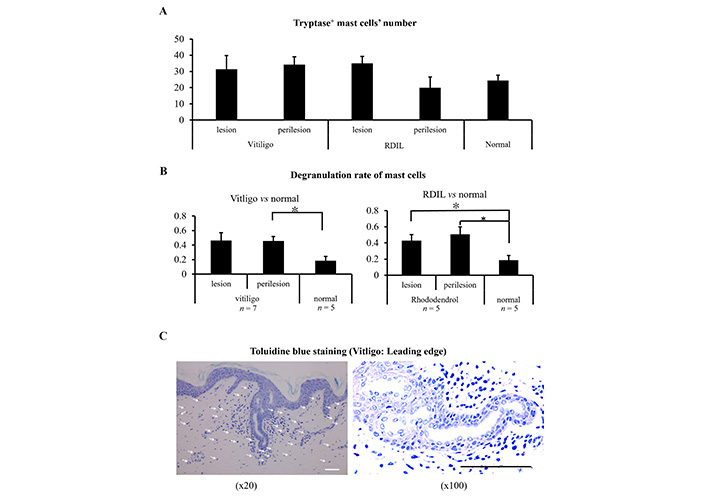
Mast cell activation in vitiligo and cosmetic-induced leukoderma. Skin sections from lesion and perilesion of vitiligo, RDIL and healthy control (normal) were stained with toluidine blue, and then the mast cell number were counted (A), the degranulation rate was calculated (B). The presentative image of toludine blue staining was shown (C). Scale bar: 100 μm
Mast cells are usually distributed around blood vessels and hair follicles or sweat glands [13]. However, in vitiligo and related leukoderma, prominent mast cell infiltration was observed in the upper dermis. Mast cells are also observed in the epidermis, where mast cells are normally absent. This phenomenon was confirmed using electron microscopy (Figure 4A). The mast cell chemotactic factors are not well recognized at present. Western blotting analysis was performed to identify possible candidate SCF for mast cell chemotaxis to the epidermis. As shown in Figure 4B, elevated SCF expression was observed in the epidermis but not in the dermis in vitiligo, which might suggest that SCF is a possible candidate for mast cell infiltration into the epidermis.
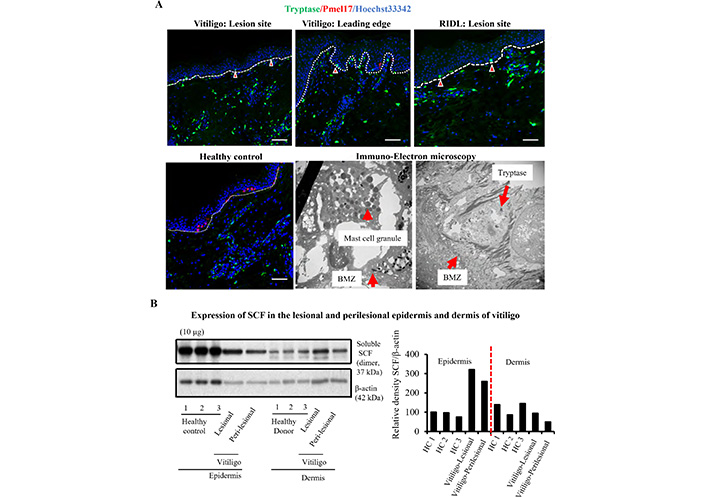
Elevated SCF expression was observed in epidermis accompanied with increased mast cell infiltration in vitiligo. Skin sections from lesion site and leading edge of vitiligo were stained with anti-tryptase in green and anti- pre-melanosomal protein (Pmel17) in red, and epidermal mast cells were examined by immune-electron microscopy using anti-tryptase staining (A). Protein extracted from lesional and perilesional skin of vitiligo were applied to western blotting analyses, the expression of SCF was detected by anti-SCF antibody (B). The red arrowheads in (A) indicate the epidermal mast cells. BMZ: basement membrane zone; HC: healthy control. Scale bar: 100 μm
It has been recently reported that GPNMB, expressed in the basal layer of the healthy skin and regulates melanosome formation in the melanocytes, was completely absent in vitiligo skin samples [14]. Interestingly, it was found that increased number of degranulated mast cells below the basement membrane (Figures 2 and 3). Similarly decreased of GPNMB expression in the BMZ was also be observed in vitiligo (Figure 5A) and RDIL (Figure 5B).
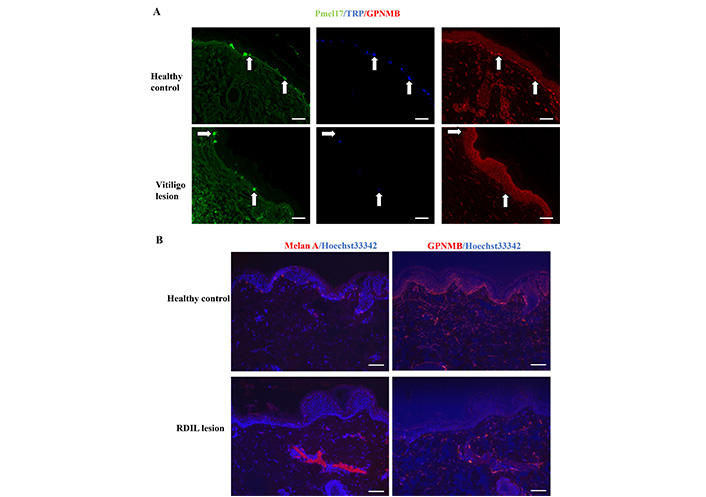
Downregulated GPNMB expression in vitiligo and RDIL. Skin sections from lesion site of vitiligo and healthy control were stained with anti-Pmel17 in green, anti-transient receptor potential (TRP) in blue and anti-GPNMB in red (A). Skin sections from lesion site of RDIL were stained with anti-GPNMB in red (B). White arrows in (A) indicate melanocytes. Scale bar: 100 μm
To confirm the underlying mechanisms of decreased GPNMB expression and its role in vitiligo, GPNMB expression in mouse tail skin was analyzed after daily application of rhododendrol, which induces leukoderma in humans [15]. Daily rhododendrol application visibly reduced both the number of Pmel17-positive melanocytes (Figure 6A) and GPNMB expression (Figure 6B) in a dose-dependent manner.
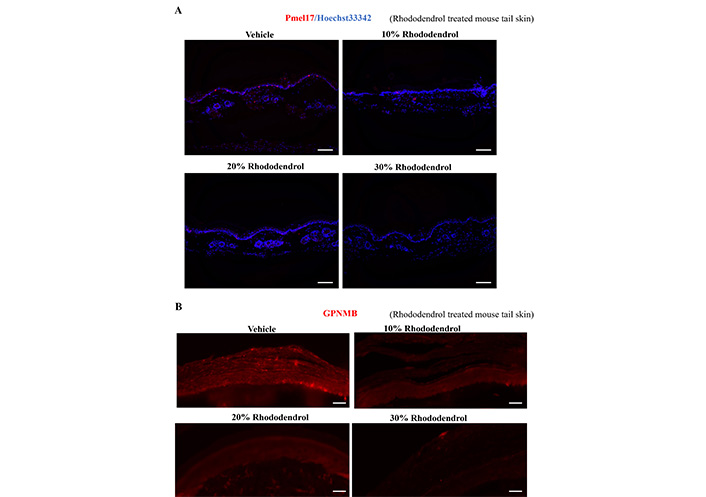
Rhododendrol application on mouse tail skin downregulates GPNMB. Topical rhododendrol from 10% to 30% v/w in ethanol were applied to the tail of 7 weeks old C57BL6/J mice. After 20 days of topical application, tail skin was collected, and skin sections were stained with anti-Pmel17 (A) and anti-GPNMB (B) antibodies. Scale bar: 100 μm
To further confirm the role of mast cells in GPNMB expression in the skin, real-time PCR (Figure 7A) and western blotting analysis (Figure 7B and C) of the GPNMB expression were performed in keratinocytes stimulated with various mast cell-derived chemical mediators. GPNMB expression was visibly upregulated by histamine and downregulated by tryptase, indicated both by our western blotting and real-time PCR analyses.
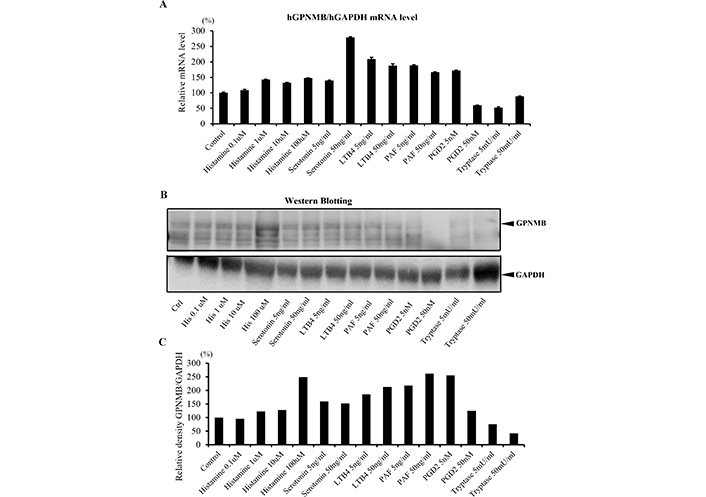
Effect of mast cell-derived mediators on GPNMB expression. Cultured human epidermal keratinocytes PSVK1 were stimulation with the indicated reagents, 24 h after stimulation, cells were collected for RNA extraction, 48 h after stimulation, cells were collected for protein extraction. The mRNA expression (A) and the protein expression (B, C) level of GPNMB were analyzed by real-time PCR (A) and western blotting (B, C). LTB4: leukotriene B4; PAF: platelet-activating factor; PGD2; prostaglandin D2
Finally, to confirm the role of keratinocyte derived GPNMB in melanocyte survival and melanin synthesis, a co-culture experiment was performed. As shown in Figure 8, unexpectedly, GPNMB siRNA-treated keratinocytes (KC) exhibited visibly upregulated melanocyte survival (Figure 8A) and melanin synthesis (Figure 8B). Furthermore, the treatment of the KC with siRNA GPNMB visibly upregulated the SCF production (Figure 8C), which might rescue melanocyte loss in the negative feedback mechanisms.
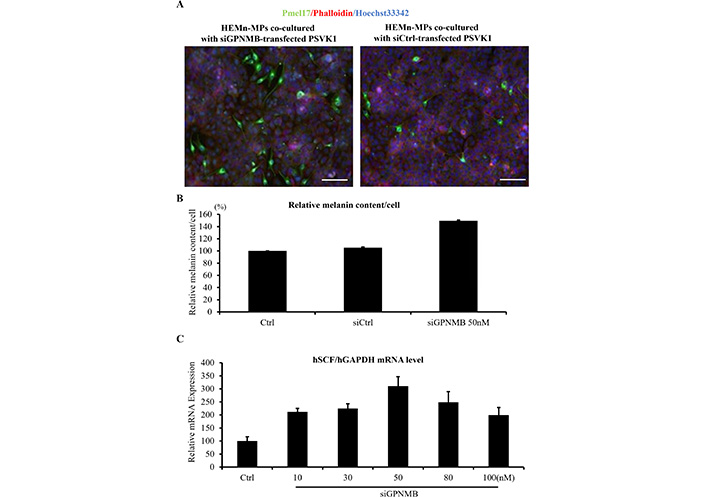
Effect of siRNA GPNMB introduced KC on melanocytes in a co-culture experiment. Human primary skin epidermal melanocytes (HEMn-MPs) were co-cultured with siGPNMB-transfected keratinocytes PSVK1 or siCtrl-transfected PSVK1. 24 h after co-culturing, cells were collected for RNA extraction, 5 days after co-culturing, cell images were collected for melanin content analyses or fixed for immunofluorescence staining. Melanocytes were stained with anti-Pmel17 antibody in green (A). Melanin content were measured in melanocytes without co-culture with keratinocytes (Ctrl), melanocytes co-cultured with siCtrl-transfected PSVK1 (siCtrl), and melanocytes co-cultured with siGPNMB-transfected PSVK1 (siGPNMB) (B). mRNA expression level of SCF in siGPNMB-transfecetd PSVK1 were examined by real-time PCR (C). Scale bar: 100 μm
Therefore, as shown in Figure 9, mast cell might possess two faces in vitiligo induction, progression and recovery through differential function of histamine on stimulation of melanin synthesis and tryptase on possible downregulation of melanosome forming protein GPNMB. Increased SCF expressions by epidermal keratinocytes might affect on not only recruitment and recovery of melanocytes but also on mast cells in the lesional skin of vitiligo.
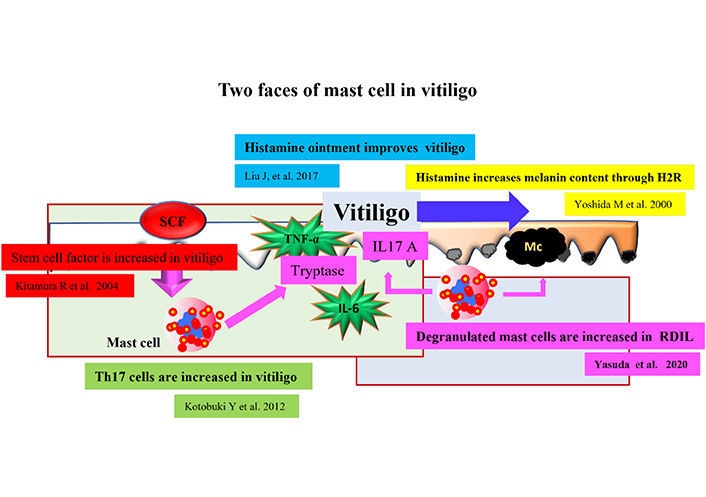
The illustration for the story of the two faces of mast cells in vitiligo. The story of our study was illustrated. H2R: histamine 2 receptor; Mc: melanocyte
Discussion
Vitiligo vulgaris is a relatively common depigmented skin disorder associated with melanocyte destruction [16]. CD8+ cytotoxic T cells under regulation of resident memory T cells (Trm) [17] or autoantibodies against melanocyte-specific proteins reportedly destroy melanocytes in autoimmune vitiligo, in addition to oxidative stress or neurotoxic effects on melanocytes. Recent reports have shown that epidermal cytokine imbalance plays an important role in the induction of hypomelanotic lesion formation. A significant increase in the expression of inflammatory cytokines, such as IL6, TNF-α, IFN-γ, or IL2, and ET-1 or SCF expression reduction show melanocyte-stimulating activity [9, 18–20]. Recently, an outbreak of RDIL occurred in Japan, resulting in social impact and interest in the precise analysis of the pathogenesis and development of effective treatments for similar refractory vitiligo [5, 6].
In a previous study, it was observed that IL17A+ T cells infiltrated to the lesional skin of vitiligo and a lesser extent in RDIL [21]. During the analysis of these unexpected observations, it was found that some of these IL17A positive cells expressed tryptase with mast cell characterization (Figures 1 and 2). IL17 double-positive cells were identified using a polyclonal anti-IL17 antibody (AF-317-NA; specificity: IL17A, B, D, F), which recognizes IL17A, B, D, F, but using a monoclonal anti IL17A antibody (AHP455G; specificity: IL17A), which recognizes IL17A. These findings suggest that the IL17 MET occurs in vitiligo, similarly to those observed in psoriasis or rheumatoid arthritis, providing a possible explanation for the increase in IL17+ cell infiltration into the lesional skin of vitiligo. It is previously reported that IL17A stimulated fibroblasts and keratinocytes to generate proinflammatory cytokines, such as TNF-α, IL1, and IL6, all of which downregulate MITF activation, thereby promoting depigmentation in vitiligo. An increased number of mast cells showed prominent degranulation and possibly secreted histamine, which might be responsible for the transient hypermelanosis of the perilesional skin in vitiligo, previously reported by Yoshida et al [8]. It is recently reported that the melanocyte-related molecule GPNMB, known for its contribution to melanosome maturation [22], is also expressed by epidermal basal keratinocytes [14]. Surprisingly, GPNMB expression in keratinocytes was significantly downregulated in the lesional but not in the perilesional skin in vitiligo. Interestingly, IFN-γ and IL17A downregulated GPNMB expression in cultured human keratinocytes, and the Janus kinase 2 (JAK2) inhibitor restored GPNMB [14]. It was observed that similar GPNMB downregulation by tryptase but not histamine or other mast cell chemical mediators might support mast cell involvement in vitiligo induction (Figure 6). Taken together, these results suggest a possible pathogenic involvement of GPNMB in vitiligo and, partly, a promising target in vitiligo treatment. Our present data, using a promising mouse model for vitiligo, demonstrated that rhododendrol clearly induces melanocyte loss with decreased GPNMB expression by the basal keratinocytes (Figure 7). In addition, siGPNMB introduction increased melanocyte survival and melanin synthesis in melanocyte/keratinocyte co-culture experiments, possibly by SCF induction with both melanocyte and mast cell growth-promoting activity (Figure 8). Recent report suggest that ultraviolet (UV) irradiation induced SCF through proteinase-activated receptor-2 (PAR2) activation in keratinocytes and possible melanin synthesis in vitiligo [23]. Kapoor et al. [24] suggest that SCF might regulate disease progression in vitiligo through c-Kit positive melanocyte and mast cell. Finally, a similar increase in mast cell infiltration was observed in rhododendrol-induced cosmetic leukoderma as in vitiligo. Therefore, persistent hypermelanosis, which impairs the QOL of the patients, might be induced by mast cell-derived histamine, which reportedly promotes melanin synthesis in UVB irradiated guinea pigs [10]. Promising results of clinical trials for vitiligo using histamine solution also support the histamine induction of hypermelanosis in the surrounding skin in vitiligo [25]. Another possible new treatments are under investigation at present targeting epidermal keratinocytes [26–30].
In conclusion, our present study suggests that mast cells are two-faced players in vitiligo pathogenesis, as described above and summarized in Figure 9. As well-known, mast cells play an important role in innate immune by production of various cytokines [31, 32], interestingly, the imbalance of cytokines are involved in vitiligo pathogenesis [1]. The results obtained in our study questioned whether these activated mast cells would be an appropriate target for treatment in vitiligo and RDIL. Besides, it might provide new information to further understand the pathogenesis and development of new, alternate therapies for refractory vitiligo.
Abbreviations
BMZ: | basement membrane zone |
GAPDH: | glyceraldehyde 3-phosphate dehydrogenase |
GPNMB: | glycoprotein non-metastatic melanoma protein B |
HC: | healthy control |
HEMn-MPs: | human primary skin epidermal melanocytes |
IFN-γ: | interferon-γ |
IgG: | immunoglobulin G |
IL: | interleukin |
KC: | keratinocytes |
LTB4: | leukotriene B4 |
MET: | mast cell extracellular trap |
PAF: | platelet-activating factor |
PCR: | polymerase chain reaction |
PGD2: | prostaglandin D2 |
Pmel17: | pre-melanosomal protein |
RDIL: | rhododendrol-induced leukoderma |
SCF: | stem cell factor |
siGPNMB: | small interfering glycoprotein non-metastatic melanoma protein B |
Th17: | T helper 17 |
TNF-α: | tumor necrosis factor α |
Declarations
Author contributions
IK, LY and WM contributed conception and design of the study; AY and FY performed the experiments and statistical analysis; IK and LY wrote the manuscript. All authors contributed to manuscript revision, read and approved the submitted version.
Conflicts of interest
The authors declare that they have no conflicts of interest.
Ethical approval
The study was approved by the ethics committee of the Osaka City University Faculty of Medicine (No.10339).
Consent to participate
Informed consent to participate in the study was obtained from all participants.
Consent to publication
Not applicable.
Availability of data and materials
Not applicable.
Funding
Not applicable.
Copyright
© The Author(s) 2021.