Abstract
The environmental impact of drug manufacturing raises concerns about sustainability in healthcare. To address this, exploring alternative approaches to drug production is crucial. This review focuses on seaweed as a sustainable resource for greening drug manufacturing processes. Seaweed offers advantages such as renewability, abundance, and a positive environmental footprint. The review begins by providing an overview of sustainable drug manufacturing practices and the challenges faced in achieving sustainability. It then discusses seaweed as a sustainable resource, including cultivation techniques and environmental benefits. Seaweed has various applications in drug manufacturing, including extracting and purifying bioactive compounds with potential therapeutic properties. Seaweed’s role in developing green technologies, such as seaweed-based excipients, biodegradable packaging materials, and as a source of sustainable energy for drug manufacturing processes, is highlighted. The environmental and economic implications of incorporating seaweed-based solutions are discussed, emphasizing reduced carbon footprint and cost-effectiveness. Regulatory and industrial perspectives are addressed, examining challenges, and opportunities for implementing seaweed-based drug manufacturing. Collaboration between academia, industry, and regulatory bodies is crucial for successful integration. The review presents future directions and opportunities, including emerging trends and innovations in seaweed-based drug manufacturing, areas for further research, policy development, and industry engagement recommendations. Incorporating seaweed into drug production facilitates a reduction in environmental impact, promotes resource efficiency, and contributes to sustainable healthcare. This review showcases seaweed-based solutions as a means to foster a greener future for drug manufacturing, addressing environmental concerns, and promoting sustainability.
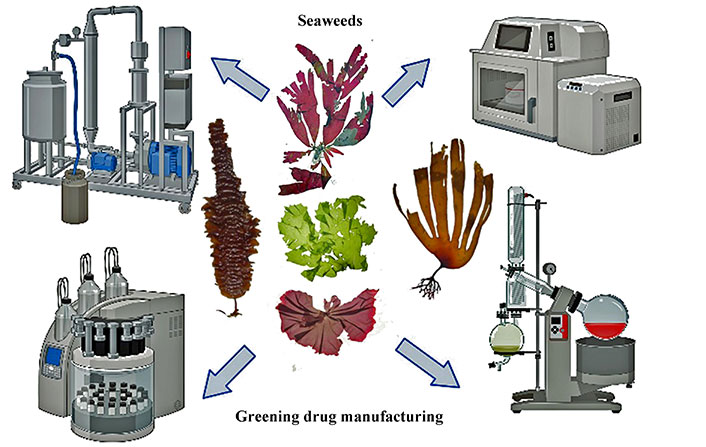
Illustrating the utilization of seaweed as a sustainable solution to enhance eco-friendly practices in drug manufacturing, aligning with the goals of sustainable healthcare
Keywords
Macroalgae, drug manufacturing, sustainability, greening, sustainable healthcare, bioactive compounds, green technologies, regulatory perspectivesIntroduction
Seaweed-based drug manufacturing is an emerging field that holds great promise for the pharmaceutical industry. Seaweeds, also known as marine macroalgae, are diverse and abundant marine organisms found in oceans and coastal regions around the world. Macroalgae (seaweed) are macroscopic, multicellular, and marine algae that are visible to the naked eye in one of their life cycle (unlike the microalgae). They have been traditionally used for various purposes, including food, fertilizers, and animal feed. However, recent research has revealed the immense potential of seaweeds as a source of bioactive compounds for drug development [1, 2].
Seaweeds are rich in bioactive compounds such as polysaccharides, polyphenols, proteins, and pigments, which possess various therapeutic properties. These compounds have shown potential as anti-inflammatory, antimicrobial, antiviral, antitumor, and antioxidant agents, among others. The unique chemical composition of seaweeds and their ability to thrive in diverse marine environments contribute to the production of a wide range of bioactive molecules [3, 4].
The utilization of seaweed in drug manufacturing is not a new concept. Traditional medicine systems in coastal regions, such as traditional Chinese medicine (TCM) and Ayurveda, have employed seaweeds for centuries to treat various ailments [5, 6]. However, with advancements in scientific research and technology, there has been a renewed interest in exploring the pharmaceutical potential of seaweeds on a larger scale [7, 8]. Seaweed-based drugs offer several advantages over conventional pharmaceutical sources. Firstly, seaweeds are a sustainable resource that can be harvested without causing significant environmental harm. Unlike terrestrial plants, seaweeds do not require freshwater or arable land for cultivation. They can be cultivated in coastal areas or in specially designed offshore farms, minimizing the impact on terrestrial ecosystems [9, 10].
Furthermore, seaweed cultivation has the potential to contribute to marine ecosystem restoration. Seaweeds are known for their ability to absorb excess nutrients, including nitrogen (N) and phosphorus (P), from the surrounding water [11]. This feature can help mitigate the negative effects of eutrophication, a process characterized by excessive nutrient enrichment in aquatic systems, leading to harmful algal blooms and oxygen depletion [12, 13]. In addition to their sustainable cultivation, seaweeds have a high growth rate, allowing for rapid biomass production. This scalability makes seaweed-based drug manufacturing a feasible and cost-effective approach, potentially reducing the dependence on traditional terrestrial sources of pharmaceutical compounds [14, 15].
This review aims to be a global and incisive review of how seaweed can be a next generation material for drug manufacturing, reducing pressure in a terrestrial environment and promoting a healthier solution using the larger ecosystem in the planet Earth: the aquatic and their lower chain base: seaweeds.
Environmental impact of seaweed-based drug manufacturing
Seaweed-based drug manufacturing has the potential to reduce the environmental impact associated with conventional pharmaceutical production [16]. The cultivation of seaweeds requires fewer resources compared to terrestrial plant cultivation. Seaweeds do not require the use of synthetic fertilizers, pesticides, or irrigation, minimizing the release of pollutants into the environment [10, 17, 18].
Moreover, seaweed cultivation can act as a carbon sink, absorbing and sequestering significant amounts of carbon dioxide (CO2) from the atmosphere. This ability to capture CO2 can help mitigate climate change by reducing greenhouse gas emissions [19]. Additionally, the extraction of bioactive compounds from seaweeds can be done using eco-friendly techniques. Supercritical fluid extraction, ultrasound-assisted extraction, and other innovative methods have been developed to minimize the use of organic solvents and energy-intensive processes [20, 21].
However, it is important to consider potential environmental challenges associated with large-scale seaweed cultivation. The introduction of non-native seaweed species for cultivation purposes may lead to ecological disruptions and the displacement of native species. Monitoring and careful management of seaweed farms are crucial to ensure the preservation of biodiversity and the prevention of invasive species outbreaks [22, 23].
Seaweed-based drug manufacturing offers an exciting avenue for the pharmaceutical industry, combining the potential for the development of novel drugs with significant environmental benefits [24]. By harnessing the bioactive compounds found in seaweeds, sustainable and eco-friendly alternatives to conventional pharmaceutical sources can be created. However, careful consideration of the environmental impact and the implementation of responsible cultivation practices are essential to maximize the benefits and minimize any potential negative effects [25, 26].
Growing need for sustainable practices in healthcare
The growing need for sustainable practices in healthcare is driven by several interconnected factors, including environmental concerns, resource limitations, and the recognition of the impact of healthcare activities on both human and planetary health [27]. As healthcare systems continue to expand and evolve, there is an increasing realization that unsustainable practices in this sector can have far-reaching consequences for the environment, public health, and future generations. Therefore, adopting sustainable practices in healthcare has become imperative for the well-being of both present and future populations [28, 29].
Healthcare activities contribute to a significant ecological footprint. Hospitals and healthcare facilities consume vast amounts of energy, water, and resources, leading to greenhouse gas emissions, water pollution, and waste generation. The use of fossil fuels for heating, cooling, and electricity, as well as the disposal of hazardous waste and single-use medical products, can have detrimental effects on ecosystems and contribute to climate change [29, 30]. The need for sustainable practices in healthcare arises from the urgent need to reduce these environmental impacts and minimize the sector’s contribution to environmental degradation [31]. Sustainable practices in healthcare are essential due to the finite nature of resources. The healthcare sector relies on various resources, including medical equipment, pharmaceuticals, and clean water, among others. However, these resources are not unlimited, and their availability may be compromised in the face of population growth, climate change, and other factors. Sustainable healthcare practices aim to optimize resource utilization, minimize waste, and ensure the long-term availability of essential resources. By embracing sustainable practices, healthcare systems can enhance resource efficiency, reduce costs, and support resilience in the face of future challenges [28, 32].
The need for sustainable practices in healthcare is closely tied to public health considerations. Climate change, pollution, and other environmental issues have a direct impact on human health, leading to increased rates of respiratory diseases, cardiovascular disorders, and other illnesses [33]. By adopting sustainable practices, healthcare systems can contribute to mitigating climate change, reducing air and water pollution, and improving overall environmental quality. This, in turn, helps to safeguard public health and create healthier environments for patients, healthcare workers, and communities [34]. Healthcare providers have a social responsibility to deliver care in a manner that minimizes harm to both individuals and the broader society. Sustainable practices align with this responsibility by promoting ethical and responsible healthcare delivery [35]. Sustainable healthcare encompasses a holistic approach that considers not only clinical outcomes but also social equity, community engagement, and patient empowerment. By integrating sustainability principles into healthcare practices, providers can ensure that healthcare delivery addresses the needs of the present generation without compromising the ability of future generations to meet their own needs [36].
Sustainable practices in healthcare can yield substantial cost savings. Energy-efficient buildings, water conservation measures, waste reduction strategies, and environmentally friendly procurement practices can lead to significant financial benefits for healthcare organizations. Reducing energy consumption, optimizing waste management, and adopting sustainable procurement policies and healthcare facilities can lower operational costs and redirect resources toward improved patient care, research, and innovation [37, 38].
The growing need for sustainable practices in healthcare stems from the recognition that environmental stewardship, resource efficiency, public health considerations, social responsibility, and cost savings are intrinsically linked. By prioritizing sustainability in healthcare delivery, organizations can reduce their ecological footprint, improve public health outcomes, ensure the availability of resources for future generations, and fulfill their ethical and social obligations. It is imperative for healthcare systems to embrace sustainable practices to safeguard both human and planetary health in the face of current and future challenges [28, 39].
Role of seaweed in greening drug manufacturing
The role of seaweed in greening drug manufacturing is gaining significant attention due to its potential to provide sustainable and eco-friendly alternatives to conventional pharmaceutical sources. Seaweeds, also known as marine macroalgae, offer unique advantages that contribute to greener drug manufacturing practices. Let’s explore how seaweed can contribute to greening drug manufacturing [9, 40].
Seaweeds are a renewable resource that can be cultivated in a sustainable manner. Unlike traditional terrestrial plants used in drug manufacturing, seaweeds do not require freshwater or arable land for growth. Therefore, they can be cultivated in coastal areas or in specially designed offshore farms, minimizing the impact on terrestrial ecosystems [9, 41]. Utilizing seaweed as a pharmaceutical source reduces pressure on land-based agriculture and preserves natural habitats [10, 42].
Seaweeds have a remarkable ability to absorb and sequester CO2 from the atmosphere. Through the process of photosynthesis, seaweeds convert CO2 into biomass, effectively acting as a carbon sink [19, 43]. Cultivating seaweed on a large-scale for drug manufacturing purposes has the potential to offset greenhouse gas emissions and contribute to climate change mitigation efforts [44].
Seaweeds have a natural resistance to pests and diseases, reducing the need for synthetic pesticides and herbicides in cultivation [45, 46]. Unlike terrestrial crops, seaweeds do not require synthetic fertilizers since they can utilize nutrients present in the marine environment. This eliminates the release of harmful chemicals into the ecosystem and reduces the ecological footprint associated with conventional agriculture [24, 47]. Seaweed cultivation requires minimal freshwater usage compared to land-based agriculture. This is particularly important in regions facing water scarcity and drought conditions [9, 24]. Utilizing seaweed for drug manufacturing conserves freshwater resources and alleviates pressure on water supplies [4, 48].
Seaweed-based drug manufacturing can employ eco-friendly extraction techniques to obtain bioactive compounds. Innovative methods such as supercritical fluid extraction, ultrasound-assisted extraction, and other sustainable extraction processes can minimize the use of organic solvents and reduce energy consumption. These techniques ensure that the manufacturing process itself is environmentally friendly and reduces the generation of harmful waste products [8, 49].
Seaweed cultivation for drug manufacturing can contribute to the conservation of marine biodiversity [19]. By providing an economic incentive for seaweed cultivation, it reduces the dependence on wild harvesting, which can deplete natural seaweed populations and harm marine ecosystems. Responsible cultivation practices, combined with proper monitoring and management, can help preserve biodiversity and protect the delicate balance of coastal ecosystems [50]. Seaweed-based drug manufacturing can make use of waste products generated from other industries. For example, certain species of seaweed can thrive on nutrients released from aquaculture operations or wastewater treatment plants, effectively transforming waste streams into valuable biomass. This contributes to the circular economy and minimizes waste disposal issues [51].
The role of seaweed in greening drug manufacturing is multifaceted. From sustainable cultivation practices to carbon sequestration, reduced chemical inputs, minimal water usage, eco-friendly extraction techniques, biodiversity conservation, and waste utilization, seaweed offers numerous opportunities for the pharmaceutical industry to embrace more sustainable and environmentally friendly practices. Incorporating seaweed into drug manufacturing processes contributes to greener and more sustainable healthcare systems while reducing the ecological impact of pharmaceutical production [52, 53].
Sustainable drug manufacturing
Overview of sustainable manufacturing practices
Sustainable drug manufacturing refers to the application of environmentally responsible and socially conscious practices throughout the entire drug manufacturing process. It encompasses various strategies and technologies aimed at reducing the environmental impact, conserving resources, minimizing waste generation, and ensuring social and economic sustainability. Here is an overview of sustainable manufacturing practices in the pharmaceutical industry [54, 55].
Green chemistry principles focus on the design of chemical processes and products that minimize the use and generation of hazardous substances. Pharmaceutical companies are increasingly adopting green chemistry approaches to develop drugs with reduced environmental impact. This includes using safer and more sustainable solvents, optimizing synthetic routes to minimize waste and energy consumption, and designing environmentally friendly manufacturing processes [56, 57].
Pharmaceutical manufacturing consumes significant amounts of energy, contributing to greenhouse gas emissions and climate change [58]. Sustainable manufacturing practices emphasize energy efficiency measures such as optimizing equipment and process design, utilizing energy-saving technologies, and implementing renewable energy sources. This includes the use of energy-efficient equipment, heat recovery systems, and the integration of renewable energy generation, such as solar panels or wind turbines [54, 59].
Water is a valuable resource, and sustainable drug manufacturing aims to minimize water usage and wastewater generation. This can be achieved through the implementation of water recycling and reuse systems, efficient water management practices, and the use of water-saving technologies [60, 61]. Additionally, technologies like membrane filtration and reverse osmosis can help in the purification and recycling of water used in manufacturing processes [62]. Sustainable manufacturing practices focus on minimizing waste generation and promoting waste reduction, reuse, and recycling. Pharmaceutical companies implement waste management strategies that adhere to environmental regulations and guidelines. This includes segregating and treating hazardous waste, implementing recycling programs for packaging materials, and exploring ways to repurpose or recover by-products and waste streams [54, 63].
Sustainable drug manufacturing also extends to the entire supply chain, ensuring ethical and sustainable sourcing of raw materials and ingredients. Pharmaceutical companies are increasingly seeking suppliers who adhere to responsible environmental and social practices. This includes sourcing from environmentally certified suppliers, promoting fair trade and labor practices, and considering the ecological and social impact of raw material extraction [64].
Life cycle assessment (LCA) is a tool used to evaluate the environmental impact of a product or process throughout its entire life cycle, from raw material extraction to disposal [65]. Sustainable drug manufacturing incorporates LCA methodologies to identify and quantify environmental hotspots and develop strategies for improvement. This allows for informed decision-making regarding the selection of materials, processes, and packaging, with a focus on reducing the overall environmental footprint of drug manufacturing [66]. Sustainable drug manufacturing involves actively engaging stakeholders, including employees, local communities, and regulatory bodies. This engagement ensures that sustainability goals are understood, supported, and implemented effectively. It also fosters transparency, accountability, and collaboration in addressing environmental, social, and economic challenges associated with drug manufacturing [29, 67].
Sustainable manufacturing practices are not static; they require ongoing assessment, monitoring, and improvement. Pharmaceutical companies employ quality management systems and implement continuous improvement methodologies to drive sustainable practices. This includes setting targets, measuring performance, and implementing corrective actions to enhance sustainability performance and reduce environmental impact [68, 69]. By adopting sustainable manufacturing practices, the pharmaceutical industry can contribute to a more environmentally friendly and socially responsible approach to drug production. Sustainable drug manufacturing not only reduces the environmental footprint of the industry but also promotes the development of safer and more effective medications that align with the principles of sustainability and public health [54, 70].
Current challenges in drug manufacturing sustainability
Sustainable drug manufacturing faces several challenges that hinder its widespread adoption. These challenges stem from technical, economic, regulatory, and cultural factors within the pharmaceutical industry. Understanding and addressing these challenges is crucial to advancing sustainable practices in drug manufacturing [71, 72]. Here are some of the current challenges. Implementing sustainable manufacturing practices often requires significant investments in new technologies, equipment, and process modifications. Pharmaceutical companies may be hesitant to adopt sustainable practices due to concerns about increased upfront costs and potential impacts on profitability. Balancing economic viability with sustainability goals remains a challenge, and there is a need for innovative financing models and incentives to encourage companies to invest in sustainable manufacturing [71, 73].
The regulatory landscape for drug manufacturing is complex and can pose challenges to the adoption of sustainable practices. Regulations often focus primarily on product safety and efficacy rather than environmental or social sustainability. There is a need for clearer and more comprehensive guidelines and regulations that encourage and incentivize sustainable manufacturing practices. Regulatory bodies play a crucial role in fostering sustainability by establishing standards and providing support for sustainable initiatives [67, 74]. The pharmaceutical manufacturing process involves complex chemical reactions and stringent quality control requirements [75]. Introducing sustainable practices may require modifications to existing processes, which can be technically challenging. Developing sustainable alternatives for certain chemicals or processes, while maintaining product quality and safety, requires extensive research and development. Overcoming technical challenges and ensuring the scalability and compatibility of sustainable manufacturing processes remains a significant hurdle [76].
The pharmaceutical supply chain is global and often involves multiple stakeholders, making it challenging to ensure sustainability throughout the entire chain. Sustainable sourcing of raw materials, managing waste streams, and monitoring social and environmental practices of suppliers can be complex and require collaboration and transparency across the supply chain. Establishing robust sustainability criteria and implementing effective supply chain management systems are necessary to address these challenges [64, 77]. Despite increasing interest in sustainability, awareness, and education about sustainable practices in drug manufacturing remain relatively limited. Many stakeholders, including manufacturers, employees, healthcare professionals, and consumers, may not fully understand the environmental and social impacts of pharmaceutical production or the potential benefits of sustainable practices. Building awareness, providing training programs, and promoting knowledge-sharing are essential to overcome this challenge [55, 67, 78]. Shifting towards sustainable practices requires a cultural shift within pharmaceutical companies. Resistance to change, risk aversion, and a lack of awareness can impede the adoption of sustainable manufacturing practices. Overcoming organizational resistance and fostering a culture that values sustainability and innovation are essential. Leadership commitment, employee engagement, and integrating sustainability into corporate values and strategies can help drive the necessary cultural transformation [79, 80].
Achieving sustainability goals in drug manufacturing requires collaboration and information sharing among industry stakeholders [81]. However, the pharmaceutical industry has traditionally been competitive and guarded about proprietary information. Encouraging collaboration, sharing best practices, and establishing platforms for knowledge exchange are crucial to overcoming this challenge and promoting sustainable drug manufacturing at an industry-wide level [82].
Addressing these challenges requires a multifaceted approach involving collaboration among industry stakeholders, regulatory bodies, and research institutions. Encouraging sustainable innovation, providing financial incentives, and developing comprehensive sustainability frameworks can incentivize pharmaceutical companies to adopt sustainable practices [67]. Additionally, raising awareness, promoting education, and fostering a culture of sustainability can create a supportive environment for sustainable drug manufacturing. By addressing these challenges, the pharmaceutical industry can advance toward more sustainable and environmentally responsible drug manufacturing practices [83].
Benefits of greening drug manufacturing for the environment and society
Greening drug manufacturing offers several benefits for the environment and society. It helps preserve the environment, mitigate climate change, conserve resources, and improve air and water quality [84]. It demonstrates social responsibility, enhances operational efficiency, and fosters innovation and competitive advantage. By embracing sustainable practices, drug manufacturers can contribute to a greener and more sustainable future while reaping social, environmental, and economic benefits [85].
Seaweed as a sustainable resource
Introduction to seaweed as a renewable and sustainable resource
Seaweed is gaining recognition as a renewable and sustainable resource with a wide range of applications. It encompasses various species, such as kelp (Saccharina species, Figure 1A), dulse (Palmaria palmata, Figure 1B), nori (Porphyra/Pyropia species, Figure 1C), and wakame (Undaria pinnatifida, Figure 1D), among others. These seaweed species offer unique properties and contribute to a more sustainable future through their rapid growth, minimal environmental impact, and numerous applications [86, 87].
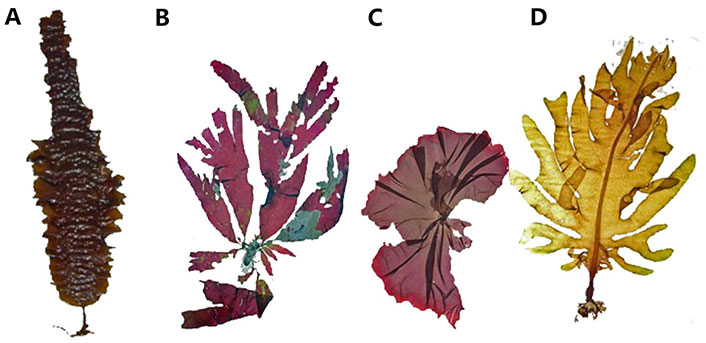
Some seaweed as a renewable and sustainable resource. (A) Kelp (Saccharina) Phaeophyceae; (B) dulse (Palmaria palmata) Rhodophyta; (C) nori (Porphyra/Pyropia) Rhodophyta; (D) wakame (Undaria pinnatifida) Phaeophyceae
One of the key reasons seaweeds are considered a sustainable resource is the rapid growth rate of species like kelp. Kelp forests can grow several centimeters per day, efficiently producing biomass. This rapid growth makes seaweed cultivation highly productive and sustainable [9, 48]. Unlike traditional terrestrial crops, seaweed cultivation does not require arable land, freshwater irrigation, or the use of synthetic fertilizers, pesticides, or herbicides. This reduces the strain on land and freshwater resources and minimizes the ecological impact associated with conventional agriculture [88].
Seaweed cultivation also has minimal negative environmental impacts. Seaweeds are cultivated in coastal areas or in specially designed offshore farms, utilizing the vast expanses of the ocean that are not suitable for other forms of agriculture. This reduces competition with food crops and avoids deforestation, soil erosion, and water contamination. Seaweeds have a low environmental footprint, making them an environmentally friendly alternative [19, 22, 23].
Seaweeds play a crucial role in marine ecosystem health, with kelp being a notable example. Kelp forests are known for their ability to absorb and remove excess nutrients, including N and P, from the surrounding water. This helps combat eutrophication, a process characterized by nutrient over-enrichment that leads to harmful algal blooms, oxygen depletion, and ecosystem degradation. Cultivating kelp on a larger scale harnesses its nutrient absorption capacity and mitigates the negative effects of eutrophication, promoting a healthier marine environment [9, 48].
Seaweeds also possess the ability to sequester significant amounts of CO2 from the atmosphere. Through the process of photosynthesis, seaweeds absorb CO2 and convert it into biomass, effectively acting as a carbon sink [89]. This carbon sequestration capacity makes seaweed cultivation an attractive strategy for climate change mitigation, as it helps to offset greenhouse gas emissions and reduce atmospheric CO2 levels [90]. Moreover, seaweed species like dulse, nori, and wakame have a broad range of applications. They can be utilized as a renewable source of bioenergy, serving as a feedstock for the production of biofuels and biogas [91]. Seaweed also holds immense potential as a feedstock for the production of bio-based materials, such as bioplastics and biofertilizers, offering sustainable alternatives to petroleum-based products [92]. Additionally, seaweed extracts and compounds from species like dulse and nori possess various bioactive properties with potential applications in pharmaceuticals, nutraceuticals, and cosmetics, presenting sustainable alternatives to conventional sources of such compounds [93].
So, seaweed species such as kelp, dulse, nori, and wakame are valuable and sustainable resources with diverse applications. Their rapid growth, minimal environmental impact, ability to absorb excess nutrients, sequester CO2, and wide range of potential uses make them attractive options for sustainable development [94]. Harnessing the potential of these seaweed species addresses environmental challenges, promotes resource efficiency, and contributes to a more sustainable and resilient future [95].
Seaweed cultivation techniques and potential for large-scale production
Seaweed cultivation techniques have shown great promise for large-scale production, offering environmental and economic benefits. Researchers, from the Marine Algae Laboratory, University of Coimbra (UC) team, have made significant contributions to optimizing these techniques [96]. Offshore cultivation involves growing seaweed in open water using floating structures like longlines and rafts. This method maximizes space and utilizes favorable water conditions for seaweed growth [97]. Integrated multitrophic aquaculture (IMTA) combines multiple species in a symbiotic system. Seaweed plays a vital role by utilizing excess nutrients from finfish or shellfish farming, reducing environmental impacts [98, 99]. Land-based systems allow year-round cultivation in controlled environments. They offer predictable growth patterns and nutrient optimization, expanding the potential for large-scale production [100]. These cultivation techniques provide several benefits, including improved water quality, resource efficiency, economic opportunities, and climate change adaptation [101]. By advancing seaweed cultivation techniques, researchers from the Marine Algae Laboratory team have paved the way for sustainable and scalable seaweed production, contributing to a more environmentally friendly and economically viable future [102].
Environmental benefits of seaweed cultivation
Seaweed cultivation presents significant environmental benefits by improving water quality through its capacity to absorb excess nutrients, such as N and P, thereby mitigating the risk of harmful algal blooms and fostering healthier aquatic ecosystems. Seaweeds also play a vital role in mitigating climate change by sequestering CO2 from the atmosphere, helping to reduce greenhouse gas emissions and combat global warming [24, 103].
Additionally, seaweed cultivation supports biodiversity by providing habitats and nursery grounds for a wide range of marine species. These underwater forests offer shelter, food, and attachment surfaces for fish, invertebrates, and other organisms, promoting species diversity and enhancing the resilience of marine ecosystems [9, 104].
Seaweed cultivation contributes to mitigating ocean acidification, a consequence of increasing CO2 levels in seawater. Seaweeds absorb carbonates, buffering the effects of acidification and creating more favorable conditions for marine organisms to thrive [105, 106].
Moreover, seaweed cultivation can aid in ecosystem restoration efforts. Cultivating and reintroducing native seaweed species in degraded areas aids in restoring biodiversity, enhancing water quality, and bolstering the overall health and resilience of coastal environments [107]. Finally, seaweed cultivation reduces the pressure on wild harvesting, which can be detrimental to natural seaweed populations and their associated ecosystems. Cultivating seaweed in a controlled and sustainable manner meets the demand for seaweed products while safeguarding the long-term health and viability of wild seaweed resources [108, 109].
Through these environmental benefits, seaweed cultivation plays a crucial role in promoting the conservation and restoration of marine ecosystems, supporting sustainable practices, and fostering a healthier planet for future generations [110].
Applications of seaweed in drug manufacturing
Extraction and purification of bioactive compounds from seaweed
Seaweed holds tremendous potential as a valuable source of bioactive compounds for drug manufacturing. The extraction and purification of these compounds from seaweed have garnered considerable interest in the field of pharmaceutical research [49]. Various techniques are employed to extract the bioactive compounds from seaweed, including solvent extraction, supercritical fluid extraction, and microwave-assisted extraction. These methods effectively isolate the desired compounds from the seaweed biomass [111]. Once extracted, purification methods are utilized to refine and enhance the purity of the bioactive compounds. Techniques such as chromatography, membrane filtration, crystallization, and solvent fractionation play a crucial role in removing impurities and isolating the target compounds. Through these purification steps, the bioactive compounds are obtained in a more concentrated and refined form, facilitating their further characterization and potential utilization [112, 113].
Structural characterization is an essential aspect of drug development. Techniques like nuclear magnetic resonance (NMR) spectroscopy, vibrational spectrometry, and infrared spectroscopy are employed to determine the chemical structures of the bioactive compounds [114, 115]. This characterization provides valuable insights into the molecular properties, functional groups, and potential biological activities of the compounds, aiding in their further evaluation and potential application in drug manufacturing [116].
The bioactive compounds derived from seaweed exhibit a wide range of therapeutic applications. For instance, seaweed polysaccharides have demonstrated immunomodulatory and antiviral properties, making them potential candidates for antiviral drug development [117, 118]. Fucoidan, a sulfated polysaccharide found in brown seaweeds, has shown promise as an anticancer agent [115]. Other bioactive compounds, such as phlorotannins and peptides, have displayed antioxidant and anti-inflammatory activities, suggesting their potential use in pharmaceutical formulations [115, 119].
So, the extraction and purification of bioactive compounds from seaweed offer exciting prospects for drug manufacturing [120]. The diverse range of extraction and purification techniques, along with structural characterization, enable the isolation and refinement of these compounds. With their therapeutic potential and sustainability advantages, seaweed-derived bioactive compounds hold promise for the development of new drugs and pharmaceutical formulations [121, 122].
Potential therapeutic properties of seaweed-derived compounds
Seaweed-derived compounds offer a wide range of potential therapeutic properties that have attracted attention in drug development. These bioactive compounds, sourced from various types of seaweed, exhibit diverse biological activities with promising applications in healthcare [123]. Here are some notable therapeutic properties of seaweed-derived compounds.
Seaweed-derived compounds possess potent antioxidant properties. They can counteract the damaging effects of free radicals in the body, reducing oxidative stress and potentially preventing chronic diseases such as cardiovascular disorders, cancer, and neurodegenerative conditions [124, 125]. Many seaweed-derived compounds exhibit anti-inflammatory properties by modulating inflammatory pathways. They can suppress the production of pro-inflammatory molecules, thereby reducing inflammation and alleviating conditions associated with chronic inflammation such as arthritis, inflammatory bowel disease, and skin disorders [126, 127]. Several compounds derived from seaweed have demonstrated promising anticancer properties [128]. They can inhibit the growth of cancer cells, induce programmed cell death (apoptosis), and impede the formation of new blood vessels that support tumor growth (angiogenesis). These compounds hold potential for use in developing novel anticancer drugs or serving as adjuvants in cancer treatment [128, 129].
Seaweed-derived compounds display broad-spectrum antimicrobial and antiviral activities. They can hinder the growth of various bacteria, fungi, and viruses, thereby potentially contributing to the development of new antibiotics, antifungal agents, and antiviral drugs [117, 130–133]. Certain seaweed-derived compounds have immunomodulatory effects, meaning they can modulate the immune system’s responses. They can stimulate immune cells, regulate the production of cytokines, and enhance overall immune function. These properties hold promise for the management of immune-related disorders and autoimmune conditions [134, 135]. Some seaweed-derived compounds, such as polysaccharides and dietary fibers, have beneficial effects on gastrointestinal health. They can promote the growth of beneficial gut bacteria, improve intestinal barrier function, and exert prebiotic effects, thus contributing to better digestive health and potentially preventing gastrointestinal disorders [136, 137].
Certain seaweed-derived compounds have shown cardiovascular benefits by helping regulate blood pressure, reduce cholesterol levels, and inhibit the formation of blood clots [121]. These properties can contribute to the prevention and management of cardiovascular diseases [137–139]. Seaweed-derived compounds have been investigated for their potential in wound healing and skin health [140, 141]. They can accelerate the closure of wounds, enhance collagen synthesis, and exhibit moisturizing and anti-aging effects. These properties make them promising ingredients for wound care products and skincare formulations [140–142]. While the therapeutic potential of seaweed-derived compounds is promising, further research and clinical studies are necessary to fully understand their mechanisms of action and ensure their efficacy and safety. Nonetheless, their diverse range of bioactive properties offers exciting prospects for the development of new drugs, functional foods, and healthcare products, aiming to promote health and well-being [49].
In this important area, there are already several commercial products based on seaweed polymers, which are normally a standard for burn wounds and deep lacerations. Due to the seaweed compounds’ benefits as anti-ultraviolet (UV) and wound moisture protection [126, 127].
Case studies showcasing the use of seaweed in drug production
While specific case studies showcasing the use of seaweed in drug production are limited, ongoing research has revealed promising findings that highlight the potential of seaweed in pharmaceutical applications. For instance, fucoidan, a complex sulfated polysaccharide found in brown seaweeds, has shown potential as an anticancer agent [115]. Preclinical studies have demonstrated its ability to inhibit cancer cell growth, induce apoptosis, and suppress tumor angiogenesis, making it a promising candidate for further exploration in cancer treatment [119, 143].
In the field of wound healing, alginic acid (Figure 2A) derived from brown seaweeds has shown promise. Alginate-based dressings create a gel-like environment that promotes wound healing by providing moisture and facilitating tissue regeneration [144]. Clinical applications of alginate dressings have been observed in the management of chronic wounds, such as diabetic foot ulcers and venous leg ulcers [145]. Furthermore, researchers have extracted and characterized various bioactive compounds from seaweed that exhibit antimicrobial, antioxidant, and anti-inflammatory properties [115]. These compounds hold the potential to address a range of therapeutic areas, including infectious diseases, chronic inflammation, and age-related disorders [146, 147]. Despite these promising findings, it is essential to emphasize that further research, clinical trials, and regulatory approvals are necessary before seaweed-based drugs can be commercially available [148]. Continued exploration of seaweed’s bioactive compounds, their mechanisms of action, and their safety profiles will provide a stronger foundation for the development of effective and safe drugs derived from seaweed sources [14, 149].
Seaweed-based green technologies in drug manufacturing
Seaweed-based excipients and delivery systems
Seaweed-based green technologies are increasingly being explored in various industries, including drug manufacturing. Seaweed offers several advantages as a sustainable and eco-friendly resource for excipients and drug delivery systems [150]. Excipients are inactive ingredients used in drug formulations to enhance the stability, solubility, and bioavailability of active pharmaceutical ingredients (APIs). Seaweed-based excipients can serve as alternatives to conventional excipients derived from non-renewable resources, such as petroleum-based polymers [151]. Seaweed polysaccharides, such as alginic acid (Figure 2A), carrageenan (Figure 2B), and agar (Figure 2C), have been studied for their potential as excipients due to their biocompatibility, biodegradability, and gel-forming properties [152]. These properties make them suitable for various drug delivery applications, including controlled-release systems and mucoadhesive formulations [152–154].
Seaweed-based delivery systems offer unique advantages in drug manufacturing. Alginate-based hydrogels, for example, can be used to encapsulate drugs and provide sustained release profiles. The gels can be tailored to control the release rate of drugs, allowing for a more precise dosing regimen, using basic systems, such as cross-linking techniques [155]. Additionally, seaweed-based nanoparticles and microspheres have been investigated as drug carriers [156]. These delivery systems can improve drug stability, protect APIs from degradation, and target specific sites in the body for enhanced therapeutic effects [156].
The use of seaweed-based excipients and delivery systems in drug manufacturing aligns with the principles of green chemistry and sustainability. Seaweed is a renewable resource that can be harvested without causing significant environmental harm. Its cultivation requires no freshwater, arable land, or synthetic fertilizers, making it a promising alternative to resource-intensive excipients. Furthermore, seaweed cultivation contributes to carbon sequestration, reducing greenhouse gas emissions and helping mitigate climate change [157, 158].
Although seaweed-based green technologies in drug manufacturing are still in the early stages of development, there is growing interest in exploring their potential. Researchers and industry stakeholders are actively investigating the formulation and manufacturing processes to optimize the performance and scalability of seaweed-based excipients and delivery systems. These technologies have the potential to reduce the environmental impact of drug manufacturing while maintaining the efficacy and safety of pharmaceutical products [97, 159].
Seaweed-based biodegradable packaging materials
Seaweed-based biodegradable packaging materials have emerged as a promising and environmentally friendly alternative to conventional plastics. The use of seaweed as a raw material for packaging offers several advantages [160].
One of the key benefits is that seaweed is a renewable resource that can be sustainably cultivated. Unlike traditional packaging materials derived from fossil fuels, seaweed can be grown rapidly and abundantly without competing for valuable land or freshwater resources. This makes it a more sustainable choice for packaging, addressing concerns related to resource depletion and land-use conflicts [24, 161].
Moreover, seaweed-based packaging materials are biodegradable, meaning they can be naturally broken down by biological processes into non-toxic components [160]. This characteristic is in stark contrast to conventional plastics that can persist in the environment for hundreds of years, causing significant pollution and harm to ecosystems. By utilizing seaweed-based materials, the accumulation of plastic waste can be mitigated, reducing the strain on landfills, oceans, and natural environments [162].
Seaweed also possesses inherent barrier properties that make it suitable for packaging applications. Its natural compounds, such as polysaccharides and proteins, provide excellent moisture resistance, oxygen barrier, and even protection against UV light [163, 164]. These properties are particularly beneficial for food packaging, as they help maintain product freshness, extend shelf life, and prevent spoilage. Seaweed-based materials can also be engineered to possess the desired mechanical strength and flexibility, making them adaptable for various packaging needs [165].
Another advantage of seaweed-based packaging is its compatibility with existing manufacturing processes. The transition from conventional packaging materials to seaweed-based alternatives can be facilitated without major disruptions or investments in new equipment. This ease of integration allows industries to adopt seaweed-based materials more readily, promoting their widespread use and making a positive impact on reducing plastic waste [166, 167]. In addition to the environmental benefits, seaweed cultivation for packaging materials has the potential to contribute to carbon sequestration. Seaweed absorbs CO2 during its growth, helping to mitigate climate change by capturing and storing carbon from the atmosphere. This carbon capture aspect further enhances the sustainability profile of seaweed-based packaging [10, 44].
While the development and adoption of seaweed-based packaging materials are still ongoing, research and innovation continue to optimize their performance, cost-effectiveness, and scalability. Standardization of processing methods and regulations regarding their use and disposal are also being addressed to ensure proper management and integration into existing waste management systems [14]. Seaweed-based biodegradable packaging materials offer a sustainable solution to the plastic waste problem. They harness the renewable and abundant properties of seaweed, providing biodegradability, barrier protection, and compatibility with existing manufacturing processes [168]. Choosing seaweed-based packaging reduces reliance on fossil fuel-derived plastics, minimizes environmental pollution, and contributes to a more sustainable future [169].
Seaweed as a source of sustainable energy for drug manufacturing processes
Seaweed holds promise as a sustainable energy source for drug manufacturing processes due to its various applications. Harnessing the energy potential of seaweed reduces reliance on fossil fuels and contributes to a more environmentally friendly approach to pharmaceutical production.
Seaweed can be transformed into biofuels such as bioethanol and biodiesel through processes like fermentation or thermochemical conversion. These biofuels can serve as renewable alternatives to traditional fossil fuels, powering the machinery and equipment involved in drug manufacturing. The conversion of seaweed into biofuels presents an opportunity to reduce greenhouse gas emissions and mitigate climate change [170]. Also, these compounds can be applied in drug manufacturing processes replacing directly synthetic ingredients [for example, seaweed-based polylactide (PLA) to substitute polyhydroxyalkanoates (PHA)].
Through a process called anaerobic digestion, seaweed can be used to produce biogas. This renewable energy source primarily consists of methane and CO2. Biogas can be utilized for heat and electricity generation, providing a sustainable power supply for pharmaceutical manufacturing facilities. Utilizing biogas reduces reliance on non-renewable energy sources and decreases carbon emissions [24, 91].
Seaweed biomass, which contains significant amounts of carbohydrates, can be harvested and processed for energy production. Methods such as combustion or gasification can be employed to convert this biomass into energy. The energy generated can be used to meet the energy-demands of drug manufacturing processes, contributing to a more sustainable and self-sufficient manufacturing ecosystem [171, 172].
Seaweed can be directly burned as a fuel source to generate heat or steam. This thermal energy can be utilized in various pharmaceutical manufacturing processes that require heat or steam. Utilizing seaweed directly avoids the need for fossil fuel-based energy sources, thereby reducing environmental impact and fostering sustainability [173, 174].
It is important to emphasize that while seaweed holds promise as a sustainable energy source, further research and development are needed to optimize the efficiency of seaweed-based energy production [175]. Additionally, careful attention must be given to sustainable seaweed cultivation practices and minimizing potential impacts on marine ecosystems. Integrating seaweed-based energy into a broader renewable energy portfolio, in conjunction with sources like solar, wind, and geothermal power, can help achieve a comprehensive and resilient energy supply for drug manufacturing processes [176].
Environmental and economic implications
Reduction of carbon footprint in drug manufacturing through seaweed-based solutions
The reduction of carbon footprint in drug manufacturing through seaweed-based solutions has significant environmental and economic implications. Exploring this approach addresses the environmental challenges linked with traditional drug production while simultaneously benefiting the economy [177, 178].
From an environmental perspective, seaweed-based solutions offer several advantages. One of the key benefits is carbon sequestration. Seaweed, through the process of photosynthesis, absorbs CO2 from the atmosphere and stores it in its biomass [179]. Cultivating seaweed specifically for drug manufacturing purposes effectively captures and stores substantial amounts of CO2, mitigating its release into the atmosphere and aiding in combating climate change [9, 19].
Furthermore, adopting seaweed-based solutions can lead to a reduction in energy consumption [180]. Traditional drug manufacturing processes often rely on energy-intensive procedures such as chemical synthesis and purification [181]. In contrast, seaweed-based alternatives offer the potential for more sustainable approaches that require less energy. Leveraging compounds derived from seaweed as natural ingredients or precursors can minimize the need for complex and energy-demanding chemical reactions, resulting in a decreased carbon footprint [53, 182]. In terms of resource utilization, seaweed-based solutions also offer advantages. Seaweed cultivation requires fewer freshwater resources compared to land-based agriculture. Reducing the demand for freshwater alleviates pressure on limited water supplies and contributes to water conservation efforts [183]. Additionally, since seaweed farming can take place in marine environments, it does not require arable land. This mitigates the conversion of land for agricultural purposes, reducing the impact on natural habitats and biodiversity [184].
Turning to the economic implications, the reduction of carbon footprint in drug manufacturing through seaweed-based solutions can lead to various benefits. One crucial aspect is cost reduction [185]. Seaweed cultivation generally requires fewer inputs, such as fertilizers and pesticides, compared to traditional agriculture. This can result in decreased production costs for drug manufacturers, potentially enhancing their competitiveness in the market [186]. Moreover, the growing demand for environmentally friendly and sustainable products presents a significant market opportunity. Seaweed-based solutions align with this trend, positioning drug manufacturers favorably by offering greener alternatives. Companies that prioritize sustainability can attract environmentally conscious consumers, potentially expanding their customer base and gaining a competitive edge [92]. Additionally, embracing seaweed-based solutions can contribute to regulatory compliance. Governments and regulatory bodies are increasingly implementing measures to reduce carbon emissions and promote sustainable practices. By adopting these solutions, drug manufacturers can align with such regulations, avoiding penalties and negative consequences associated with non-compliance. This enhances their reputation and strengthens their position in the market [187].
Lastly, the reduction of carbon footprint in drug manufacturing through seaweed-based solutions fosters opportunities for innovation and research. Developing and implementing these solutions require scientific advancements, collaboration between various stakeholders, and technological innovations. This presents an avenue for academia, industry, and government entities to collaborate, leading to potential economic benefits through patents, licensing, and commercialization of new technologies [188]. The reduction of carbon footprint in drug manufacturing through seaweed-based solutions offers compelling environmental benefits such as carbon sequestration, reduced energy consumption, and resource conservation. Simultaneously, it provides economic advantages, including cost reduction, market potential, regulatory compliance, and opportunities for innovation. Embracing these solutions contributes to a more sustainable and economically viable future [189, 190].
Cost-effectiveness and scalability of seaweed-based processes
Seaweed farming holds immense potential for producing feedstocks for various applications, including food, feeds, fertilizers, biostimulants, and biofuels. Unlike land-based biomass, seaweeds offer advantages such as no freshwater inputs and no need for arable land allocation. However, large-scale seaweed farming for meaningful biofuel production has not been extensively practiced. In this context, a techno-economic model was developed to assess the feasibility of large-scale seaweed farms, specifically focusing on sugar kelp (Saccharina latissima) (Figure 1A) in cool temperate regions and Eucheumatopsis isiformis in tropical regions [191]. According to the model, when considering seaweed farms spanning 1,000 ha or more, the estimated farm gate production costs range between $200 and $300 per dry ton, considering offshore locations up to 200 km from the onshore support base. The model also suggests the possibility of achieving production costs below $100 per dry ton in certain scenarios, which would make seaweeds economically competitive with land-based biofuel feedstocks. However, it is important to note that these results are based on the model’s assumptions and require further validation through field studies [192].
The techno-economic model highlights the potential economic viability of large-scale seaweed farms for biofuel production, with estimated production costs in certain scenarios being economically competitive with land-based feedstocks. However, additional research and validation are necessary to confirm these findings and ensure the feasibility of seaweed farming as a sustainable and economically viable source of biofuels [193].
On the other hand, in order to limit global surface warming to below 1.5°C by 2100, it is necessary to expand the range of cost-effective CO2 removal (CDR) technologies. To evaluate the potential of macroalgae-based CDR, a bio-techno-economic model for kelp aquaculture was developed. The model proposes large-scale farming of kelp at an offshore site, followed by transportation to a deep water “sink site” where the kelp would be deposited below the sequestration horizon at a depth of 1 km [194]. Regarding associated emissions of macroalgae production, costs and associated emissions was estimated for various components of the project, including nursery production, permitting, farm construction, ocean cultivation, biomass transport, and monitoring, reporting, and verification (MRV). The baseline project, covering 1,000 acres (405 ha) in the Gulf of Maine, USA, utilized current kelp cultivation systems adapted for deep water sites (0.1 km) using the best available modeling methods. The levelized cost of CO2 capture (LCOC) was calculated at $17,048 per metric tons of CO2 equivalent (tCO2eq) [195]. Despite the annual sequestration of 628 metric tCO2eq within the kelp biomass at the sink site, the project only generated 244 carbon credits (tCO2eq) each year, resulting in a true sequestration “additionality rate (AR)” of 39%. This refers to the ratio of net carbon credits produced to the gross carbon sequestered within the kelp biomass. However, optimizing 18 key parameters within a range identified in the literature led to a reduction of LCOC to $1,257 per metric tCO2eq, accompanied by an increase in the AR to 91% [195]. These results demonstrate that significant cost reductions can be achieved through process improvement and decarbonization of production supply chains [195].
Challenges in implementing kelp CDR include high production costs, energy-intensive operations, and uncertainties in MRV. To address these challenges, research and development efforts should focus on [195, 196]:
De-risking farm designs to maximize lease space.
Automating seeding and harvest processes.
Utilizing selective breeding to increase yields.
Evaluating the cost-effectiveness of gametophyte nursery culture for selective breeding and cost reduction.
Decarbonizing equipment supply chains and energy usage by adopting renewable energy sources and low-emission materials.
Developing low-cost and accurate MRV techniques for ocean-based CDR.
Overall, while kelp-based CDR shows promise, it requires advancements in technology, cost reduction, and improved monitoring techniques to overcome current limitations and realize its full potential as a viable CDR solution [197].
LCA and environmental impact analysis of seaweed-based drug manufacturing
An LCA and environmental impact analysis of seaweed-based drug manufacturing involves a detailed evaluation of the environmental impacts associated with the entire life cycle of the drug production process [198]. This includes assessing the environmental effects from the extraction of raw materials to the disposal of waste generated [198, 199].
The first step is to define the goal and scope of the study, which includes determining the purpose of the assessment, specifying the functional unit (such as the production of one unit of the drug), setting system boundaries, and identifying the relevant environmental impact categories to be considered (such as greenhouse gas emissions, water use, energy consumption) [200]. Next, an inventory analysis is conducted to identify and quantify all the resources and emissions associated with seaweed-based drug manufacturing. This involves assessing the inputs required, such as raw materials, energy, and water, as well as the outputs generated, including emissions and waste produced at various stages of the production process. This step ensures a comprehensive understanding of the resources consumed and the environmental burdens associated with the manufacturing process [201].
The impact assessment phase follows, where the potential environmental impacts associated with the identified inputs and outputs are evaluated. This is typically done using recognized impact assessment methods, such as ReCiPe or IMPACT World+, which provide a framework for quantifying and categorizing the impacts. Environmental impact categories such as climate change, acidification, eutrophication, and human toxicity are assessed to gauge the overall environmental consequences of the drug manufacturing process [202]. Once the impacts are assessed, the results are interpreted to identify significant environmental hotspots, trade-offs, and areas where improvements can be made. This involves analyzing the data to understand the key contributors to environmental burdens and identifying opportunities for reducing these impacts. Sensitivity analysis, scenario comparisons, and other tools may be utilized to explore different possibilities and assess their potential environmental benefits [203].
Based on the interpretation of results, improvement opportunities are identified. These may include optimizing cultivation practices to reduce resource consumption, improving extraction processes to minimize waste generation, adopting energy-efficient technologies, incorporating renewable energy sources, implementing waste reduction and recycling measures, or exploring eco-friendly packaging options. These strategies aim to reduce the environmental footprint of seaweed-based drug manufacturing [204]. Finally, the findings of the analysis are documented in a report, which includes a clear description of the methodology used, the results obtained, and the conclusions drawn [205]. It is important for the study to adhere to established LCA guidelines, such as the International Organization for Standardization (ISO) 14040 and ISO 14044, to ensure transparency and credibility [205]. If desired, the study can also undergo external verification by independent experts to validate its accuracy and reliability [205].
Overall, an LCA and environmental impact analysis of seaweed-based drug manufacturing provides valuable insights into the environmental performance of the process and highlights opportunities for sustainable improvements. This information can guide decision-making, support the development of more sustainable drug production practices, and contribute to the overall environmental sustainability of the pharmaceutical industry [22, 206].
Regulatory and industrial perspectives
Regulatory considerations and challenges for seaweed-based drug manufacturing
Regulatory considerations and challenges for seaweed-based drug manufacturing encompass several important aspects [15].
Seaweed-based drugs are subject to regulations set by drug regulatory authorities such as the Food and Drug Administration (FDA) or European Medicines Agency (EMA). Manufacturers must navigate these frameworks, which include guidelines and requirements for product approval, safety assessment, and efficacy evaluation. Compliance with these regulations is essential to ensure market access and patient safety [207]. Seaweed-based drugs must meet stringent quality and safety standards. This involves adhering to good manufacturing practices (GMP) and implementing robust quality control measures throughout the manufacturing process. Manufacturers must demonstrate consistent product quality, purity, stability, and control of contaminants [208]. Seaweed species contain various bioactive compounds that contribute to the therapeutic properties of seaweed-based drugs. However, due to the natural variability of seaweed, identifying and standardizing these compounds can be challenging. Manufacturers need to develop reliable methods for the identification, extraction, and standardization of active compounds to ensure consistent drug potency and efficacy [209, 210].
Seaweed-based drugs may require a specific regulatory approval pathway, especially if they have unique characteristics or mechanisms of action. Manufacturers need to work closely with regulatory authorities to determine the appropriate pathway for approval. This may involve conducting additional clinical trials or providing specific data to support the safety and efficacy of the product [127, 211]. Seaweed-based drug manufacturing often emphasizes sustainability. Regulatory authorities may have an interest in evaluating the sustainability claims associated with these products. Manufacturers may be required to provide evidence of sustainable sourcing practices, environmental impact assessments, and calculations of carbon footprint to support their sustainability claims [162, 187]. Protecting intellectual property rights is important for manufacturers of seaweed-based drugs. This involves securing patents, trademarks, or other forms of intellectual property protection for unique compounds and manufacturing processes. Intellectual property rights help safeguard innovations and provide a competitive advantage in the market [212].
Regulatory authorities conduct inspections and audits to ensure compliance with established regulations. Manufacturers of seaweed-based drugs must be prepared for these inspections, which may include assessments of manufacturing facilities, documentation, quality control processes, and safety measures. Maintaining comprehensive records and demonstrating compliance is essential for regulatory approval and continued market access [213, 214]. Regulatory harmonization across different regions and countries facilitates global market access for seaweed-based drugs. Collaboration between regulatory authorities is crucial for streamlining regulatory processes, aligning standards, and reducing duplicative efforts. Manufacturers should actively engage in initiatives that promote international regulatory harmonization [215, 216]. Effectively addressing these regulatory considerations and challenges is vital for the successful development, approval, and commercialization of seaweed-based drugs. Collaboration between regulatory authorities, industry stakeholders, and researchers is essential to establish appropriate guidelines, ensure patient safety, and enable the global availability of these innovative pharmaceutical products [15].
Industry adoption and implementation of greening drug manufacturing with seaweed
The greening of drug manufacturing through the use of seaweed has gained attention as a potential solution to address the environmental impact of pharmaceutical production. Seaweed, specifically certain species—brown: Ascophyllum (Figure 3A), Laminaria (Figure 3B), Saccharina (Figure 3C), Fucus (Figure 3D), Undaria (Figure 3E), Cystoseira (Figure 3F), and Sargassum (Figure 3G); red: Chondrus (Figure 3H), Porphyra (Figure 3I), Kappaphycus (Figure 3J), and Palmaria (Figure 3K); green: Ulva (Figure 3L), Cladophora (Figure 3M), Halimeda (Figure 3N), Chaetomorpha (Figure 3O), and Caulerpa (Figure 3P)—offers several compelling benefits that make it an attractive option for the industry [4, 9].
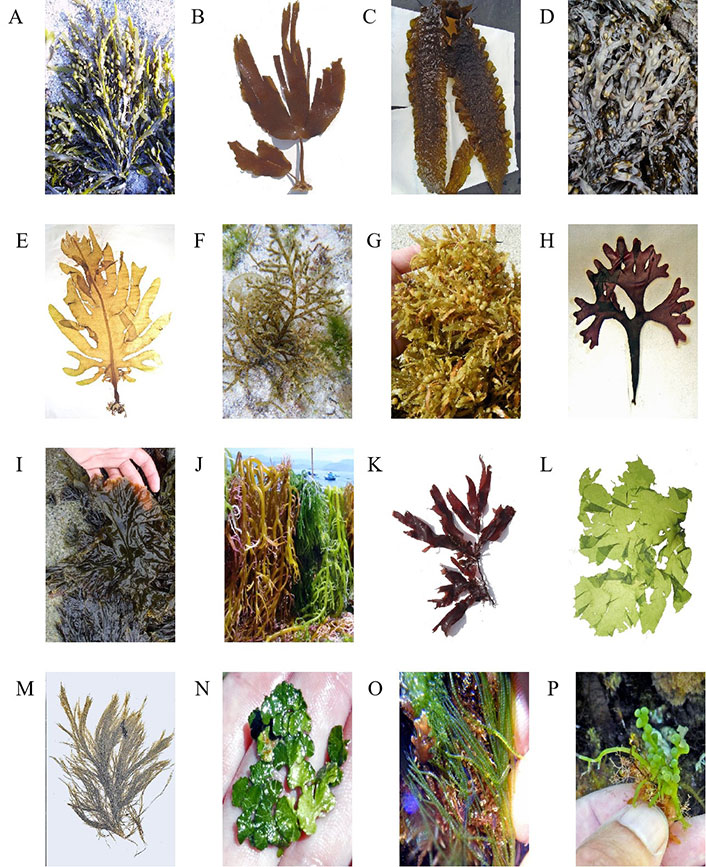
Some seaweeds potentially useful for the pharmaceutical industry. Brown: (A) Ascophyllum; (B) Laminaria; (C) Saccharina; (D) Fucus; (E) Undaria; (F) Cystoseira; (G) Sargassum. Red: (H) Chondrus; (I) Porphyra; (J) Kappaphycus; (K) Palmaria. Green: (L) Ulva; (M) Cladophora; (N) Halimeda; (O) Chaetomorpha; (P) Caulerpa
One of the key advantages of using seaweed is its ability to sequester CO2 from the atmosphere. As seaweed grows, it absorbs significant amounts of CO2, helping to mitigate greenhouse gas emissions. By cultivating and harvesting seaweed specifically for pharmaceutical manufacturing, it is possible to offset the carbon emissions generated during the production processes, making it a potentially valuable strategy for reducing the industry’s carbon footprint [24, 217, 218].
Another important aspect of seaweed is its sustainable sourcing potential. Seaweed can be cultivated in coastal areas, utilizing oceanic resources without the need for freshwater or arable land. This aspect makes it an environmentally friendly alternative to land-based crops that require extensive resources and contribute to deforestation and water scarcity [92, 219]. Additionally, since seaweed grows rapidly and does not compete with food crops for resources, it minimizes potential conflicts between food production and pharmaceutical manufacturing [211, 220]. The utilization of seaweed in drug manufacturing also presents an opportunity to reduce waste generation and promote a circular economy [221]. Seaweed contains a variety of bioactive compounds that can be extracted and utilized in pharmaceutical applications [210]. By harnessing these valuable substances, the pharmaceutical industry can tap into a renewable resource and reduce reliance on synthetic chemicals, potentially minimizing waste and environmental pollution [222]. Furthermore, seaweed cultivation can have positive impacts on marine ecosystems. The artificial habitats created by seaweed farms can serve as shelters and nurseries for marine organisms, contributing to biodiversity conservation and supporting ecosystem restoration efforts [223]. This ecological benefit further highlights the potential of seaweed-based approaches in greening drug manufacturing [216].
While industry-wide adoption and implementation of seaweed-based processes in drug manufacturing may not be extensively documented, various research initiatives and pilot projects are underway to explore the feasibility and efficacy of this approach [224]. These endeavors aim to develop sustainable extraction methods for obtaining bioactive compounds, optimize seaweed cultivation techniques, and evaluate the safety and effectiveness of pharmaceutical products derived from seaweed [8]. However, it is important to note that the widespread adoption of greening drug manufacturing with seaweed may require further advancements in research, technology, and regulatory frameworks [225]. Economic considerations, scalability of seaweed cultivation on a commercial level, and ensuring the safety and quality of seaweed-derived pharmaceutical products are also important factors to be addressed [226].
Given the dynamic nature of the field, it is advisable to stay informed about recent publications, industry news, and academic research to keep up to date with the latest developments regarding the adoption and implementation of seaweed-based approaches in drug manufacturing [227].
Collaborative efforts and partnerships for sustainable drug manufacturing
The European seaweed industry is shifting from harvesting wild seaweed to farming it. This transition requires a rethinking of the European sector’s role in a global scale. It is necessary to gain insight into the organization and innovation of the global seaweed value chain. The study on value chains using Gereffi’s conceptual framework presents results from a systematic review of scientific publications published between 2010 and 2020 for five markets: pharmaceuticals, bioplastics, biostimulants, alginate, and cosmetics [228].
Gereffi’s conceptual framework is a tool used to analyze global value chains. It was developed by Gary Gereffi, a professor of sociology at Duke University [228]. The framework is used to identify the key actors and activities involved in the production of goods and services, as well as the governance structures that shape these activities [229]. The framework has three main components: governance, upgrading, and territoriality. Governance refers to the relationships between firms and other actors in the value chain. Upgrading refers to the process of moving up the value chain by improving product quality or developing new products. Territoriality refers to the geographic distribution of activities in the value chain [230].
In the study mentioned earlier, Gereffi’s conceptual framework was used to analyze global seaweed value chains [228]. The study aimed to gain insight into the organization and innovation of the global seaweed value chain by conducting a systematic review of the pharmaceuticals, bioplastics, biostimulants, alginate, and cosmetics market [231]. The study concluded that innovation in seaweed use is happening globally and that focusing solely on high-value applications will not differentiate the nascent European seaweed sector from established producing regions such as Asia. The global value chains studied are organized around strong lead firms that require suppliers to produce according to codified product characteristics [228]. To meet the demands of regulators, lead firms, and consumers, the European seaweed sector needs to increase collaboration and develop joint efforts to create safe and sustainable products. Stronger coordination in the value chain will facilitate further business development by stimulating collaboration and innovations [232].
Future directions and opportunities
Emerging trends and innovations in seaweed-based drug manufacturing
In the field of seaweed-based drug manufacturing, there are several emerging trends and opportunities for future advancements.
Scientists are actively researching different seaweed species to discover and understand the various bioactive compounds they contain. These compounds, such as polysaccharides, proteins, peptides, pigments, and polyphenols, have shown potential for drug development [14]. By exploring unexplored habitats and utilizing advanced techniques like metagenomics, researchers hope to uncover novel compounds with therapeutic properties [3, 233].
Seaweed farming is already practiced in many regions, but there is a need for further advancements in cultivation methods. Innovations such as offshore cultivation systems, integrated multi-trophic aquaculture, and automated monitoring systems can enhance the efficiency and scalability of seaweed production. These developments aim to ensure a sustainable and reliable supply of seaweed biomass for drug manufacturing [15, 109].
Biotechnology plays a significant role in seaweed-based drug manufacturing. Scientists are using genetic engineering and synthetic biology techniques to enhance the production of specific bioactive compounds in seaweed. By modifying metabolic pathways or introducing genes from other organisms, they aim to improve the yield and quality of target compounds [3, 209].
As seaweed-based drugs move closer to commercialization, it is crucial to establish standardized protocols for extracting, purifying, and ensuring the quality of bioactive compounds [234]. Research efforts are focused on developing reliable and reproducible methods for extracting these compounds from seaweed. Standardized quality control measures will guarantee the safety, efficacy, and consistency of seaweed-based drugs [130].
Developing effective drug formulations and delivery systems is essential for optimizing the therapeutic potential of seaweed-based drugs. Techniques such as encapsulation, nanotechnology, and controlled-release systems are being explored to improve the stability, bioavailability, and targeted delivery of bioactive compounds. Scientists are tailoring formulation strategies for specific applications, such as oral delivery, topical formulations, or targeted drug delivery systems [235–237].
Seaweed-based drug manufacturing requires collaboration between various disciplines, including marine biologists, pharmacologists, chemists, and engineers. Future directions involve fostering interdisciplinary collaborations to accelerate research and development in this field [238]. Collaborative efforts between academia, industry, and government organizations can facilitate the translation of research findings into commercial products [239].
With an increasing focus on sustainability and environmental impact, future developments in seaweed-based drug manufacturing will prioritize eco-friendly practices. Efforts will be made to reduce the environmental footprint of seaweed cultivation, optimize resource utilization, and develop biodegradable packaging materials. Sustainable practices will ensure the long-term viability of seaweed-based drug manufacturing and contribute to the conservation of marine ecosystems [240, 241].
The future of seaweed-based drug manufacturing holds tremendous potential for discovering new bioactive compounds, improving cultivation techniques, leveraging biotechnology, establishing standards, optimizing drug formulations and delivery systems, promoting collaboration, and prioritizing sustainable practices. These advancements will contribute to the development of novel drugs derived from seaweed, offering exciting opportunities for the healthcare and pharmaceutical industries [206].
Why seaweed can be a trend in the pharmaceutical area and for the world welfare
Greening drug manufacturing with seaweed can play a crucial role in establishing a sustainable healthcare system [242]. The pharmaceutical industry is a major contributor to environmental degradation due to its significant energy consumption, greenhouse gas emissions, and water pollution. Shifting towards seaweed-based solutions addresses these issues while simultaneously promoting sustainability and advancing the healthcare sector [243].
Seaweed cultivation for drug manufacturing offers numerous environmental benefits for the world welfare. Seaweeds are fast-growing marine organisms that require minimal land, freshwater, and chemical inputs [41, 48]. Cultivating seaweed for pharmaceutical purposes reduces the need for land-intensive crops and minimizes the use of synthetic fertilizers and pesticides, thus decreasing the industry’s ecological footprint [10, 48].
Another advantage of greening drug manufacturing with seaweed is the potential for economic growth and social development [123]. Seaweed cultivation and processing can create employment opportunities, particularly in coastal communities where traditional livelihoods will be at risk in the next years. Supporting the development of a seaweed-based pharmaceutical industry stimulates local economies and enhances social well-being while simultaneously addressing environmental concerns [10, 183].
For the pharmaceutical area, embracing greener practices in drug manufacturing aligns with the growing demand for sustainable healthcare solutions. Consumers are increasingly conscious of the environmental impact of the products they use, including pharmaceuticals. By offering medications derived from sustainable seaweed sources, pharmaceutical companies can meet this demand and enhance their reputation as environmentally responsible organizations. This, in turn, can drive innovation and market competitiveness while contributing to a more sustainable healthcare system overall [244].
Thus, greening drug manufacturing with seaweed holds immense importance for building a sustainable healthcare system. Harnessing the environmental benefits, sustainable production processes, preservation of marine ecosystems, economic opportunities, and consumer demand creates a pharmaceutical industry that promotes both human health and planetary well-being. The integration of seaweed-based solutions represents a significant step towards achieving a greener, more sustainable future for healthcare [1, 43, 244].
Conclusions
Greening drug manufacturing with seaweed will lead to the development of more sustainable and eco-friendly production processes. Seaweed contains a wide range of bioactive compounds with potential pharmaceutical applications. With these seaweed natural compounds, pharmaceutical companies can reduce their reliance on synthetic chemicals, which are often derived from non-renewable resources and contribute to pollution. Seaweed-based drugs can provide sustainable alternatives that are both effective and environmentally friendly, this is observed already in some areas where seaweed-based pharmaceuticals have been used. However, seaweeds in the pharmaceutical area are still at the beginning of the research and development of new solutions. The main question is to pass from academic studies and initial assays to something more developed, such as preclinical assays, pharmacodynamics, and pharmacokinetics. Thus, nowadays, there is ineffective related disease (RD) work in seaweeds to be further exploited as pharmaceutically active substances. This is derived from the difficult connection between academic and industry RD teams and objectives. However, this is being slowly resolved due to the pressure to develop new raw sources for active ingredients, mainly in seaweed phenolic compounds and volatile fatty acids.
Another point to this developing area is that incorporating seaweed into drug manufacturing can contribute to the preservation of marine ecosystems. Promoting sustainable seaweed farming practices helps protect coastal habitats and biodiversity. Seaweed cultivation offers additional benefits, such as nutrient uptake and water purification, improving the overall health of marine environments. Sustainable harvesting and cultivation techniques can ensure the long-term viability of seaweed resources, safeguarding their ecological importance. Thus, promoting better welfare for the world population and alleviating the health problems related to pollution.
Abbreviations
CDR: |
carbon dioxide removal |
CO2: |
carbon dioxide |
LCA: |
life cycle assessment |
MRV: |
monitoring, reporting, and verification |
N: |
nitrogen |
P: |
phosphorus |
tCO2eq: |
tons of carbon dioxide equivalent |
Declarations
Author contributions
LP: Supervision, Conceptualization, Investigation, Writing—original draft. JC: Writing—review & editing. All authors read and approved the submitted version.
Conflicts of interest
The authors declare that they have no conflicts of interest.
Ethical approval
Not applicable.
Consent to participate
Not applicable.
Consent to publication
Not applicable.
Availability of data and materials
Not applicable.
Funding
Not applicable.
Copyright
© The Author(s) 2024.