Abstract
In recent decades, abundant methods for targeted tumor cell immunotherapy have been developed. It was recently discovered that excellent curative effects observed in hematological tumors cannot be achieved in solid tumors, as serious side effects will occur. These are all derived from engineered adaptive immune cells, the use of which will bring limitations. γδT cells have a unique ability to respond to a variety of tumor cells while linking innate immunity and adaptive immunity, and thus, they are an ideal source of therapeutic allogeneic cells. This review introduces strategies that can optimize the clinical application of γδT cells to provide novel ideas for adoptive immunotherapy in the future.
Keywords
Adoptive immunotherapy, γδT cells, antitumor capacityIntroduction
γδT cells are primarily distributed in subcutaneous tissue, intestinal mucosa, the respiratory tract, and the urogenital canal [1]. Since they can quickly identify infected and mutated cells, they are considered the first line of defense for “dissidents” inside the human body [2]. γδT cells participate in immune regulation in the body, present antigens, induce the maturation of dendritic cells (DCs), and exert cytotoxic effects to kill tumor cells [3, 4]. Activated γδT cells can secrete perforin, granzyme A/B, interferon-γ (IFN-γ) and tumor necrosis factor-alpha (TNF-α), and can express Fas ligand (FasL) and TNF-related apoptosis-inducing ligand (TRAIL) simultaneously [5]. In addition, γδT cells infiltrate a variety of tumor tissues, such as colorectal, breast, prostate, and ovarian carcinomas [6, 7]. The importance of γδT cells in immune surveillance has also been confirmed in multiple mouse models [8]. Mice lacking γδT cells are prone to malignant skin tumors [9]. In humans, the distribution and phenotype of γδT cells in tumors can also serve as prognostic indicators in cancer patients [10]. Some studies have also reported that interleukin-17 (IL-17)—secreting γδT cells exert a tumor-promoting effect, which will not be described in this review [11].
Studies on the genetic engineering technology of tumor immunotherapy based on allogeneic transplantation predominantly focus on αβT cells, but the main limitation of this approach is that these cells can cause severe graft-versus-host disease (GvHD) [12], and inefficient infiltration of solid tumors has led to a limited curative effect in solid tumors [13]. However, γδT cells can recognize various tumor cells through a variety of innate cytotoxic receptors expressed on their surface [14]. This broad response can reduce the opportunity of tumors to escape, and the independent manner by which major histocompatibility complex (MHC) molecules recognize tumor cells [15] to effectively avoid GvHD and cytokine release syndrome (CRS); therefore, this approach allows more opportunities for γδT cells to be designed through genetic engineering.
Biologic features of γδT cells
γδT cells are relatively small in circulating T cells, with a content of about 1–5% in peripheral blood. They express a diversified T-cell receptor (TCR) repertoire composed of a γ chain and a δ chain. Different functional subsets of γδT cells may have a different function in antitumor immunity.
Vγ9Vδ2 T cells are the most abundant subset [16] in peripheral blood, as they account for 50–90% of all of the γδT cells [17]. The main target of this group of cells is the nonprotein pyrophosphate metabolite produced by the host mevalonate pathway [isopentenyl pyrophosphate (IPP)] or the microbial nonmevalonate pathway (E)-4-hydroxy-3-methyl-but-2-enyl pyrophosphate (HMBPP), namely phosphoantigens (pAg) [18]. This process can be blocked by nitrogen-containing bisphosphonates (N-BPs), which leads to the accumulation of pAg in cells, this, in turn, activates Vγ9Vδ2 T cells. Zoledronate (ZOL) as a representative stimulus plays an important role in the activation and expansion of Vγ9Vδ2 T cells [17]. In some studies, the combination of ZOL in the form of liposomes and Vγ9Vδ2 T cells can significantly improve its efficacy [19, 20].
Vδ1+ T cells constitute the main population of γδT cells in the mucosa and skin. These cells can be activated by many ligands, including stress-induced autoantigens, glycolipids presented by CD1c/d, and self-lipids, as well as potential ligands that have not yet been determined [21, 22]. It has been reported that Vδ1+ T cells have a wide range of tumor cell recognition capabilities [23], which indicates that they are a potential tool in antitumor immunotherapy.
Most non-Vγ9Vδ2 T cells and non-Vδ1+ T cells in humans express Vδ3+ TCR chain, accounting for about 0.2% of circulating T cells, but they are enriched in the liver and some patients with chronic virus infection and leukemia. Vδ3 T cells are glycolipid reactive T cells, which specifically recognize CD1d, release T-helper 1 (Th1), Th2, and Th17 after activation, and induce DC cells maturation [24]. However, due to their small number, there is no effective large-scale amplification method for immunotherapy, so it will not be introduced in this review.
Different from αβT cells, γδT cells do not rely on MHC molecules for TCR mediated antigen recognition, and can directly mediate cytotoxicity through cytotoxic cytokines, granzyme, and perforin [1]. At least three cytotoxicity pathways of how γδT cells kill tumor cells have been described. One is γδTCR-dependent cytotoxicity, another is cytotoxicity mediated by receptor-ligand binding, such as NK cell receptor (NKG2D)-NKG2D ligands (NKG2DL), Fas-FasL, TRAIL-TRAIL receptor (TRAILR) and so on, and the third is antibody-dependent cytotoxicity (ADCC) via the Fc domain of antibodies that bind to tumor cell antigens recognized by γδT cells [25]. Based on these, γδT cells represent a promising immune population for the next generation of immunotherapy.
Methods of expanding γδT cells ex vivo for adoptive therapy
Adoptive immunotherapy using γδT cells has produced ideal effects in both solid and hematological tumors in mice and humans. Regardless of whether the cells are used alone or in combination with IL-2 or ZOL, the recipients experience no serious adverse reactions [26–31]. Since γδT cells account for a relatively small proportion of peripheral blood and tumor-infiltrating T lymphocytes, many studies are devoted to obtaining clinical-scale γδT cells. It has been reported that vitamin C helps to increase the expansion of γδT cells [32]; moreover, Vγ9Vδ2 T cells incubated with IL-2 and IL-15 exhibit stronger cytotoxicity [33], and in the absence of IL-2, the delivery of Vγ9Vδ2T cells in vivo requires high doses of IL-15 [34]. Some researchers developed a new formula to improve the expansion of γδT cells in vitro. This formula consists of ZOL, IL-2, IL-15, and vitamin C. Compared with the culture system that only adds ZOL and IL-2, γδT cells expanded according to the new formula can produce higher levels of effector molecules (IFN-γ, TNF-α, and NKG2D), which increases cytotoxicity against a variety of tumor cell lines in vitro and in vivo [35]. IL-21 is a cytokine that has been proved to promote the cytotoxicity of natural killer (NK) cells and CD8+ T cells and can enhance cytotoxicity and proliferation [36]. Studies have shown that HMBPP can induce the up-regulation of IL-21 receptors (IL-21Rs) on human γδT cells in the amplification system. γδT cells show stronger antitumor activity [37], such as granzyme and perforin. The signal of the IL-21R can inhibit γδT cells secreting IL-17 [38]. The presence of IL-21 can also affect the differentiation and polarization of γδT cells and have more memory phenotypes [39]. The erythroleukemia cell line K562 was modified, irradiated, and used as a feeder cell line to express costimulatory molecules CD19, CD64, CD86, CD137L, and the membrane-bound protein IL-15 (mIL-15), which was supplemented by the addition of exogenous IL-2 and IL-21. This engineered cell line can substantially expand γδT cells derived from the peripheral blood of healthy donors or tumor patients. γδT cells expanded in vitro are not a specific subtype and these polyclonal γδT cells exhibit a wide range of resistance to tumor cells [7, 40–42]. However, IL-21 also increased the expression of T-cell immunoglobulin and mucin domain 3 (TIM-3), making its efficacy limited.
Agents with the potentials to stimulate γδT cells in vivo
Bispecific antibodies enhance cytotoxic activity by selectively targeting tumor-associated antigens [such as human epidermal growth factor receptor 2 (HER2), CD19, epidermal growth factor receptor (EGFR), epithelial cell adhesion molecule (EpCAM)] recognized by γδT cells, by carrying CD3, CD16 or Vγ9 domains in the recombinant protein which activates γδT cells, CD107a on the surface of γδT cells, intracellular IFN-γ and TNF-α are significantly increased, which can lead to stronger cytotoxic activity in tumor cell lines isolated in vivo and drug-resistant tumor cells [40, 43–46]. In addition to conventional antibodies, nanobodies that lack the CH1 domain of light and heavy chains are produced by camelids and can be used as alternatives to traditional antibodies [47]. Some researches based on the specific heavy chain of HCAbs (VHH) of Vγ9Vδ2 T cells can not only induce activation, degranulation and cytokine production in Vγ9Vδ2 T cells, but it can also be used in flow cytometry analysis, magnetic bead sorting, and immunohistochemistry [48].
Some drugs also affect the cytotoxicity of γδT cells by affecting the signaling pathways involving γδT cells. In mice, rapamycin can inhibit the mechanistic target of rapamycin (mTOR) signaling in Vγ4δ T cells expanded in vitro, which leads to the upregulation of NKG2D and increased TNF-α expression, thereby enhancing their cytotoxic effects against a variety of tumor cell lines [49]. In the treatment of patients with nonmuscular invasive bladder cancer (NMIBC), Bacillus Calmette-Guerin (BCG) is the standard treatment method. When rapamycin was used in combination with BCG, the levels of IL-8 and TNF-α were significantly increased in the patients’ urine, and in a mouse xenograft model, rapamycin promoted the killing of humans squamous cell carcinoma mediated by γδT cells [50]. Some therapeutic monoclonal antibodies, such as rituximab, can activate the phospho-zeta-chain-associated protein kinase-70 (ZAP70) and phospho-extracellular signal-regulated kinases 1 and 2 (ERK1/2) signaling pathways in γδT cells, and enhance their ADCC activity [51–53]. The Wnt signaling pathway plays a key role in the differentiation, survival, and immune response of T lymphocytes. As an activator of this pathway, TWS119 can enhance the activity of γδT cells to lyse on tumor cells through the perforin-granzyme pathway [54]. Studies have found that the T cell immunoglobulin domain and TIM-3 are significantly up-regulated during the expansion of γδT cells in vitro, which reduces the production of perforin and granzyme B in γδT cells through the ERK1/2 pathway. The use of TIM-3 inhibitors can prevent apoptosis of γδT cells and promote their accumulation at the tumor site [55, 56]. In one interesting study, acute dynamic exercise was used as an experimental model. Controlled exercise of a certain intensity within 30 minutes could increase the number of γδT cells in the peripheral blood by nearly three-fold; these effects occur through β2 adrenergic receptor signaling, which enhances the expansion and antitumor activity of γδT cells in vitro [57].
Selenium is an essential trace element that helps maintain the health and survival of eukaryotic cells [58, 59]. Selenium nanoparticles (SeNPs) are considered as excellent antitumor active substance [60]. The particles can reorganize the cytoskeleton by upregulating tubulin-α, which increases the rigidity of γδT cells. SeNP-pretreated γδT cells can significantly upregulate the expression of NKG2D, CD16, IFN-γ, and other cytotoxicity-related molecules while downregulating the expression of programmed cell death protein 1 (PD-1), which will prolong the survival time of γδT cells in vitro [61].
Some drugs can affect the susceptibility of tumor cells to toxicity by affecting the NKG2DL-NKG2D axis, Fas-FasL signaling, or TRAIL-TRAILRs signaling between γδT cells and tumor cells; examples of such drugs include, disintegrins and metalloproteinase inhibitors [62], bortezomib [63, 64], doxorubicin [65], decitabine [66], temozolomide [67], 4,5-diazafluorene derivatives (14c) [68], carboplatin [69], valeric acid [70]. Pretreatment of tumor cells with these drugs can increase the expression of NKG2DL, Fas, or TRAILRs on their surface, thereby promoting tumor cell apoptosis. Even bisphosphonates that are used to amplify γδT cells can increase the sensitivity of tumor cells to γδT cells when they are pretreated [71–74]. It had been reported that the replacement of the hydroxyl group in ZOL with fluoride ions, and as a result of this structure, γδT cells became more capable of killing tumor cells [75] (Figure 1).
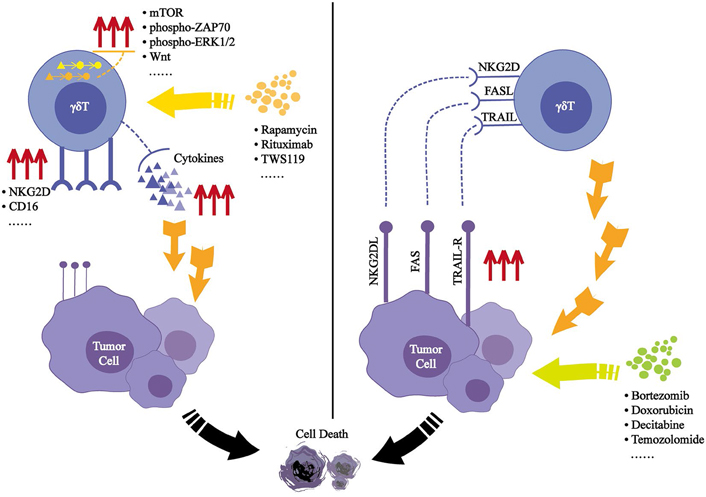
Effected induced by small molecules after acting on γδT cells or tumor cells. Small molecules can activate the signaling pathways in γδT cells, leading to secrete added effector molecules or induce further expression of surface receptors, which will mediate its cytotoxic effects on tumor cells. (Left) Small molecules can cause enhanced tumor cell surface ligands expression, thus (Right) enhancing its recognition by γδT cells. PAS: polyglandular autoimmune syndromes
The complementarity determining region 3 (CDR3) of the γδTCR determines the binding affinity of pAg-expressing tumor cells [76]. Some research identified a specific CDR3 region in tumor infiltrating lymphocytes (TILs) in human ovarian epithelial carcinoma and named it OT3 [77]. Thus, a fusion protein containing OT3 and the human IgG (hIgG) Fc domain was designed. This fusion protein exhibited the ability to bind properties to a variety of human tumor cell lines in vitro and could mediate cell killing through ADCC. It also significantly inhibited tumor progression in human ovarian cancer xenograft models and improved the survival rate of tumor-bearing mice [78].
All the methods described above provide better combination treatment strategies that can improve γδT cell antitumor immunotherapy at a later stage.
Transfusion of natural γδT cells
Autologous transplantation
Previous clinical trials have evaluated the safety and feasibility of autologous infusion of γδT cells. Clinical trials have included patients with solid tumors and those with hematological tumors. Most of the γδT cells used were amplified from the products resulting from ZOL and IL-2 treatment in vitro and contained mostly Vγ9Vδ2 T cells in vitro by ZOL and IL-2, which were then returned to the patients by intravenous injection.
During the treatment of patients with advanced renal cell carcinoma (RCC), it was found that the percentage of γδT cells in RCC patients was significantly lower than that in healthy individuals [79]. Among 11 subjects who had undergone nephrectomy and had lung metastases, after adoptive treatment of γδT cells, the tumor doubling time was prolonged in all patients, complete response (CR) for one patient, stable disease (SD) for five patients, and progressive disease (PD) for five patients. One patient with 5 metastatic tumors, 3 metastases disappeared within 3 months and 2 disappeared within 7 months, and there was no evidence of tumor recurrence for three years [80]. In another clinical study, 3 of 7 RCC patients showed increased numbers of γδT cells in the peripheral blood and an increased response to antigens in vitro, two patients showed favorable reactivity to antigen and survived, while five patients died with the progression of the disease [79]. Some studies have used the proportion of γδT cells in the peripheral blood as a prognostic indicator in RCC patients [81]. One method to expand Vγ9Vδ2T cells in vitro into products by Bennouna et al. [82] containing highly enriched Vγ9Vδ2T cells, which were administered to patients with metastatic RCC (mRCC). A reduction in tumor tissue was observed in two of ten patients, and repeated infusions were well tolerated, SD for six patients and PD for four patients [82]. In cases of autologous reinfusion in patients with multiple myeloma, the proportion and the absolute number of Vγ9Vδ2 T cells in peripheral blood were increased in 6 patients after the fourth treatment, and most increases were maintained for more than 4 weeks. Twenty-four hours after administration, the IFN-γ level in the serum and NKG2D expression on γδT cells were increased significantly; 3 of 6 patients experienced mild side effects after treatment, including fever, itching, and fatigue unrelated to the tumor, and no serious adverse reactions were observed [83]. In a clinical study of 18 patients with advanced solid tumors, one group of patients was treated with indium111-labeled Vγ9Vδ2 T cells. In these patients, Vγ9Vδ2 T cells had rapidly migrated to the lungs where they remained for 4–7 h; at later stages, they also migrated to the liver and spleen, CR for only one patient. This clinical trial provides preliminary evidence for the migration of Vγ9Vδ2T cells in vivo [84]. Intraperitoneal injection of Vγ9Vδ2 T cells expanded in vitro and combined with ZOL was used to treat malignant ascites of gastric cancer. ZOL was administered 24 h before intraperitoneal injection to sensitize the tumor cells, and the volume of ascites was significantly reduced in 2 of 7 patients without treatment-related deaths [71]. Its safety has been verified primarily in patients with non-small cell lung cancer [85, 86].
The most common adverse reaction in the abovementioned clinical trials was fever, and no serious GvHD reaction was observed, which demonstrates the safety of γδT cells in clinical practice. Although autologous transplantation of γδT cells can inhibit tumor cell growth in vivo, it is difficult to amplify γδT cells from patients due to the reduced number of lymphocytes after radiotherapy and chemotherapy.
Allogeneic transplantation
Patients with solid or hematological tumors have been included in several existing clinical trials. In phase I clinical trial, in which 414 cell infusions were performed in 132 patients with advanced cancer, Vγ9Vδ2 T cells were expanded with additional IL-15 and vitamin C; this clinical trial did not reveal significant side effects, which clearly verified the clinical safety of Vγ9Vδ2 T cells expanded by this new method. Among the included patients, 8 with liver cancer and 10 with lung cancer who received more than 5 adoptive immunotherapies exhibited a substantially prolonged survival, among them, 5 patients survived 30–35 months, which preliminarily demonstrates the curative effect of these cells [35]. One patient who was diagnosed with recurrent mediastinal lymph node metastasis due to cholangiocarcinoma after liver transplantation received 8 infusions of γδT cells from healthy donors, which also demonstrates the safety of this treatment [87]. After 62 patients with locally advanced pancreatic cancer (LAPC) were treated with irreversible electroporation (IRE), 30 received γδT cell infusion. The survival rate of these patients was significantly increased, the median overall survival (OS) increased from 11 months to 14.5 months, and patients who received multiple courses of infusion therapy also exhibited longer survival times than those who only received a single course of treatment. The maximum transverse diameter of the tumor was also significantly smaller in those who received infusions, which also reflects the effectiveness of allogeneic γδT cells [88]. During the process of haploidentical bone marrow transplantation in 4 patients with advanced refractory hematological malignancies who were not suitable for allograft transplantation, the returned cell product contained approximately 20% γδT cells, while the remainder were NK cells and CD4−CD8− αβT cells. After treatment, 3 patients achieved complete remission, which continued for 2 to 8 months, one patient died from an infection 6 weeks after treatment. No patients exhibited any signs of acute or chronic GvHD or organ damage [89].
Since they are not MHC restricted, γδT cells from healthy donors have always been considered for use in an allogeneic immunotherapy strategy, but their safety and feasibility need to be determined by clinical research in a large number of cancer patients.
Genetic engineering γδT cells
γδTCR transfer into αβT cells
Marcu-Malina et al. [90] first proposed the transfer of easily available Vγ9Vδ2TCR into CD4+ and CD8+ αβT cells, which can effectively kill a variety of tumor cell lines in vitro and can also inhibit colony formation of primary leukemia cells. These cells could also inhibit disease progression in a mouse leukemia model [90]. The specific CDR3δ region OT3 from human epithelial ovarian cancer has been described above. This region was transplanted into the CDR3 region of the δ chain in the γδTCR to engineer cells by Zhao et al. [91], which significantly improved the survival rate of nude mice with ovarian epithelial cancer. However, the limitations of γδT cell treatment include the diversity of γδTCRs and regulation by innate receptor ligands. To overcome these obstacles, TEG cells developed by Trudy Straetemans can express a specific γδTCR. This Vγ9Vδ2TCR clone was selected from the natural repository of healthy individuals that are highly responsive to tumors [76]. The resulting TEG cells are superior to natural Vγ9Vδ2 T cells in recognizing a variety of tumor cells, and these TEG cells can migrate to the tumor cells in a 3D primary myeloma cell niche model in vitro and exert cytotoxic effects [92]. Since the current production process of TEG cells usually does not include a purification step, Straetemans and others have also developed a good manufacturing practice (GMP)-level manufacturing process to remove αβT cells in this system [93, 94]. Some studies have also used single-cell sequencing technology to analyze γδTILs in breast cancer, and 15 types of TEG cells have been generated by reconstructing matched TCRγ and TCRδ. These cells can combat not only breast cancer cells but also other tumor cell types [95].
A new antibody-TCR platform was designed by Xu et al. [96] that combined the Fab structure of the CD19 antibody with the transmembrane and intracellular domains of γδTCR. In a patient-derived xenograft mouse model, engineered cells with this structure could activate tumor-suppressing effects similar to those of CD19-chimeric antigen receptor (CAR) T (CAR-T) cells, which release fewer cytokines [96].
Studies have shown that TCRγ and TCRδ will not form heterodimers with TCRα and TCRβ chains [78, 85]. The use of γδTCR can effectively avoid the mismatch of the endogenous αβTCR of T cells, enable αβT cells to recognize a wider range of tumor cells, and can reduce serious adverse reactions caused by MHC recognition.
αβTCR transfer into γδT cells
Since endogenous and introduced TCRs may form mixed dimers, the use of γδT cells as recipient cells may prevent the formation of such specific unknown T cells. van der Veken et al. [97] expressed OT-I αβTCR specific to OVA on γδT cells, which could proliferate in vivo in an antigen-specific manner and persist in the body. Data from mouse models indicated that this protocol might be a safe method for adoptive immunotherapy against specific antigens [97]. Transduction of specific TCRs against other antigens such as mHag HA-2, cytomegalovirus B7 (CMV-B7) [98], melanoma-associated antigen A4 (MAGE-A4) [99], and gp100/HLA-A2 [100] enable γδT cells to recognize target cells in an αβTCR-dependent manner and to exert antigen-specific cytolytic activity.
NKT TCR transfer into γδT cells
NKT cells are innate immune cells derived from bone marrow that can produce direct cytotoxic effects in tumor cells. Similar to γδT cells, NKT cells do not cause GvHD, which means that they are an ideal source of therapeutic [101–103]. One study transfected NKT cell-derived TCRα and β chain mRNA to form bifunctional γδT cells, which can be stimulated by the NKT ligand α-galactose ceramide to secrete IFN-γ. NKT cells can also respond to both NKT cell and γδT cell ligands and exert an antitumor effect [104].
CAR T engineered strategies
CD19-CAR-αβT cells generate an impressive clinical response in the treatment of leukemia but have failed to achieve equivalent success in solid tumors. In addition, CAR-αβT cells also result in severe CRS, neurotoxicity, and GvHD [12].
Studies on CAR-γδT cells are scarce, the first study on CAR-γδT cells was published in 2004. First- generation CARs were used to target the specific GD2 antigen in neuroblastoma and Ewing’s sarcoma (EWS). CAR-γδT cells exhibit specific toxicity to GD2-positive target cell lines. In one study, compared with untransduced γδT cells, CD69 was upregulated on the surface of CAR-γδT cells when they were incubated with tumor cells [105]. Second-generation CARs targeting the GD2 region, whether transduced into Vδ1+ T or Vδ2+ T purified γδT cell subsets, exert specific cytotoxic effects on GD2-positive tumor cell lines, such as LAN1 or TC-71 [106]. To avoid possible off-target effects and T cell exhaustion in CAR-αβT cells, one study used the NKG2D adaptor protein DAP10 as an intracellular costimulatory signal. Only when CD3 and its costimulatory signal receptors were involved did CAR-γδT cells become activated and release cytokines [107]. In addition, CAR-γδT cells targeting CD19 [17, 108], NKG2D [109], gp100/HLA-A2 [100], carcinoembryonic antigen (CEA), and HER2 [41] have antitumor effects on corresponding tumor cells in vivo and in vitro.
Vδ1+ T cells as a new choice in adoptive cell therapy
It has been reported that Vδ1+ T cells are increased in patients with B-cell chronic lymphocytic leukemia (B-CLL) [110, 111], while human CMV infection is a complication after allogeneic stem cell transplantation that activates, Vδ1+ T cells in vivo after transplantation. This activation has a positive impact on patients’ prognosis [112], which indicates that the CMV antigen is crucial to the expansion of Vδ1+ T cells. The low abundance of Vδ1+ T cells in peripheral blood hinders their clinical use, and thus, some studies have used irradiated artificial antigen-presenting (aAPCs) that express CD86, 41BBL, CD40L, and CMV-pp65 as feeder cells to expand γδT cells. The resultant polyclonal cell population showed high expression of activated memory markers without signs of exhaustion, namely, Vδ1+ T cells, and could exhibit antitumor activity in vivo in a mouse model of leukemia [113]. The addition of concanavalin A (ConA), phytohemagglutinin (PHA), or OKT monoclonal antibody (mAb) to the culture system results in a high percentage expansion of the Vδ1+ cell. These TCR agonists can selectively induce Vδ1+ T cells to express NK cell receptors (NCRs) such as NKp30, NKp44, and NKp46, which increases their resistance to activation-induced cell death and exhaustion after continuous stimulation [114–116].
A rapid and reproducible clinical transformation protocol developed by the Almeida et al. [116] termed DOT cells. The program is divided into two phases. The first phase involves a TCR agonist and IL-4 costimulation to greatly expand the number of cells, and during the second phase, IL-15 is added to induce NCR expression. Using this scheme, Vδ1+ T cells can be expanded by more than 1,000 times in three weeks. Genetic manipulation and feeder cells are not needed for the production process. In vitro, DOT cells target unspecified leukemia cells and bind to a wide range of recognition domains. DOT cells also exert a cytotoxic effect on drug-resistant acute myeloid leukemia (AML) cells that survive after chemotherapy [116, 117].
TILs are activated by autoantigens of hematological tumors, and the detection of TILs in other solid tumors also supports the response of Vδ1+ T cells to malignant tumors. Vδ1+ T cells isolated from colorectal cancer TILs mainly express NKp46, not only because autologous or allogeneic epithelial tumor cells are cytotoxic but also because this can delay the risk of metastatic tumors [118, 119]. After Vδ1+ T cells and Vδ2+ T cells from TILs derived from samples were expanded in vitro, all Vδ1+ T cells were able to exert cytotoxic effects on the A375 cell line cultured in vitro, while only two Vδ2+ T cell samples were cytotoxic [120].
Vδ1+ T cells are not susceptible to apoptosis induction. They have unique innate recognition and regulation abilities, and thus, they exert strong lytic activity on tumor cells. They can persist in circulation for many years and provide lasting immunity against certain cancers [22]. Consequently, Vδ1+ T cells are gradually becoming a promising candidate for cancer immunotherapy.
Conclusions
At this stage, γδT cell adoptive immunotherapy has achieved certain successes. So far, there are no serious adverse reactions related to γδT cells therapy, which has satisfactory therapeutic effects on hematologic tumors and solid tumors. γδT cells can also distinguish tumor cells from healthy cells by sensing the stress signals specifically expressed by tumor cells. However, the treatment process is associated with two main problems: on the one hand, γδT cells apoptotic during continuous stimulation in vitro, which results in reduced cytotoxicity; on the other hand, it is difficult for the infused γδT cells to infiltrate or accumulate at the tumor site [55]. Therefore, understanding the mechanism of dysfunction of adoptively transferred γδT cells is essential for their future clinical application. The distribution and abundance of T cells play a key role in tumor immunotherapy. Research has led to the development of a stable and accurate method for evaluating the abundance of immune cells from transcriptome data. This Immune Cell Abundance Identifier (ImmuCellAI) can provide important insights for dynamic immune cell infiltration during immunotherapy, and provide valuable indicators into immunotherapy [121]. Studies have also reported that γδT cells in mice that secrete IFN-γ completely rely on glycolysis for their metabolism, and thus, glucose supplementation could enhance the antitumor function of these cells. However, the level of oxidative metabolism of IL-17 secreting cells is increased, and the mass and activity of mitochondria are increased with the uptake of lipids, which accelerates tumor progression in obese mice. It was found that the metabolic resources needed by different subsets of γδT cells can be controlled to amplify antitumor effects, which provides a metabolism-based plan for tumor intervention treatment [122].
Abbreviations
ADCC: | antibody-dependent cytotoxicity |
CAR: | chimeric antigen receptor |
CDR3: | complementarity determining region 3 |
CMV: | cytomegalovirus |
ERK1/2: | extracellular signal-regulated kinases 1 and 2 |
FasL: | Fas ligand |
GvHD: | graft-versus-host disease |
IFN-γ: | interferon-γ |
IL-17: | interleukin-17 |
MHC: | major histocompatibility complex |
NK: | natural killer |
NKG2D: | natural killer cell receptor |
NKG2DL: | natural killer cell receptor ligands |
pAg: | phosphoantigens |
RCC: | renal cell carcinoma |
TCR: | T-cell receptor |
Th1: | T-helper 1 |
TILs: | tumor infiltrating lymphocytes |
TIM-3: | T-cell immunoglobulin and mucin domain 3 |
TNF-α: | tumor necrosis factor-alpha |
TRAIL: | tumor necrosis factor-related apoptosis-inducing ligand |
TRAILR: | tumor necrosis factor-related apoptosis-inducing ligand receptor |
ZOL: | zoledronate |
Declarations
Author contributions
XZ wrote the manuscript. YX helped to edit the figure. HC, JZ and WH revised the manuscript. All authors contributed to the article and approved the submitted version.
Conflicts of interest
The authors declare that they have no conflicts of interest.
Ethical approval
Not applicable.
Consent to participate
Not applicable.
Consent to publication
Not applicable.
Availability of data and materials
Not applicable.
Funding
This work was supported by the National Natural Science Foundation of China (81972866, 31970843, 82071791 and U20A20374), Chinese Academy of Medical Sciences Initiative for Innovative Medicine (2021- 1-I2M-005 and 2021-1-I2M-035) and Chinese Academy of Medical Sciences Basic Research Expenses (2018PT31052). The funders had no role in study design, data collection and analysis, decision to publish, or preparation of the manuscript.
Copyright
© The Author(s) 2022.