Abstract
Brain metastasis is the most prevalent neurologic problem of systemic cancer and it can increase the mortality rate in patients with cancer. It occurs more in patients with lung cancer, breast cancer, and melanoma. There are several molecular mechanisms in cancer cell progression, invasion, and location in new places during brain metastasis. Significant interactions between cancer cells, the brain microenvironment, and the blood-brain barrier (BBB) play a major role in brain metastasis. This study will focus on molecular mechanisms that contribute to cancer metastasis into the brain and finding new treatments with molecular research. Treatment strategies in patients with brain metastasis include surgical resection, radiotherapy, and chemotherapy; however, the penetration of chemotherapy drugs beyond the BBB is limited. Studying molecular, cellular, and physical mechanisms in brain metastasis helps to improve new strategies in drug delivery across the BBB. There are significant impacts of ion channels in brain metastasis and cancer treatment failure. Targeting molecular mechanisms and ion channels in brain metastasis led to increasing the better response in these patients. In this way, nano-drugs have caused a revolution in effective targeting and drug delivery in cancer treatment. This review describes the advances to facilitate the penetration of drugs in the BBB by using nano-drugs especially those that are targeting ion channels.
Keywords
Brain metastases, genetic alterations, blood-brain barrier, epithelial-mesenchymal transition, ion channel, nano-drugIntroduction
The most frequent cancers in the central nervous system (CNS) are caused by metastases but cancer databases often underestimate the prevalence of symptomatic brain metastases [1]. Researches indicated that the brain metastases rate in patients who are evolving with cancer is 20%. Brain metastases mostly occur when tumor cells circulate into the brain microvasculature and tumor growth is promoted in this unique microenvironment but the treatment and the entrance of chemotropic drugs are limited in this condition [2]. Lung cancer, breast cancer, and melanoma are the most prevalent cancers for developing brain metastases, and their contributions to this problem are estimated for 67% to 80% of all cancers. A remarkable number of patients with brain metastases show extracerebral metastases at the same time. Research declared that more than 40% of patients with breast and lung cancer suffer from brain metastasis; however, some patients present brain metastasis without any primary cancer diagnosis. Brain metastases in children are rare but germ cell tumors, neuroblastoma, and sarcomas are more common [3–5].
Because of the blood-brain barrier (BBB), the brain has a unique perivascular environment which acts as an elective tissue for penetrating molecules based on their size and charge and they represent around the most blood vessels for restricting flow of molecules from the blood. The BBB prevents the penetration of various hydrophilic chemotherapeutics and this can help to rescue metastatic tumors in treatment [6]. Novel systematic treatment is based on molecules with large sizes such as trastuzumab which is barely can across from the BBB [7]. Thus, new advances are urgently needed in treatment methods for curing or control of disease in brain metastasis patients.
This study will focus on molecular mechanisms in brain metastasis which are involved in escaping cancer cells from the origin, invading adjacent tissues, finding a way in the microvasculature, evading cell death, and locating to the apart site (extravasation), proliferating and colonizing in a new location finally. Understanding the molecular mechanisms in brain metastases are effective in developing new treatments. This paper will discuss treatments that are based on molecular mechanisms and nanotechnology and suggest effective treatment with nano-particles and further studies in this area.
Molecular mechanisms in brain metastasis
Heterogeneity of cells and proliferation
The cancer cells’ potential to metastasize is variable because of their natural or therapeutic genetic heterogeneity. Despite local environmental stresses like nutrient deficiency, hypoxia, and immune mediation, tumor cells could metastasize to normal tissue and invade tissues, disseminate and adhere to them, and then initiate angiogenesis. The capacity of tumor cells for evading has derived from changes in inhibitors and growth suppressor factors of cell proliferation in different mechanisms. For example, apoptosis resistance by overexpression of anti-apoptotic molecules like B cell lymphoma-2 (Bcl-2), and Bcl-extra-large (Bcl-xL) and down-regulation of pro-apoptotic molecules such as Bcl-2-associated X protein (Bax) and Bcl-2-interacting mediator of cell death (Bim) can be found in metastasis [8]. In addition, changes in epithelial cells are frequent in metastasis. The structural form of epithelial cells in normal tissue is supported by some proteins while decreasing in these proteins’ expression especially E-cadherins can be seen in metastatic behavior. Metastatic cells produce proteolytic enzymes that can degrade the epithelial basement membrane (BM) which has an important function in maintaining the BBB. Invader cells attach to the microvessel endothelial cells in the brain and lyse the BM cells then invader cells penetrate to the body circulation and invade the BBB. Invader cells which are captured in capillary beds, pass to the parenchyma and can start the angiogenesis process in the next step [9, 10]. There are heterogeneous cell lines in primary tumors like cancer stem cells (CSC), incompletely differentiated progenitor cells, and completely differentiated end-stage cells. These types of cells act without control despite normal tissue and they can contribute to the increase of malignancy features in primary tumors. CSCs with undifferentiated attributes can destroy the extracellular matrix (ECM) from entering to the blood vessels and lymph nodes. These activities facilitate migration, extravasation, and colonization of malignant cells at new locations [3, 11–14].
Epithelial-mesenchymal transition
Tumor cells with the origin epithelial gain the ability to invasion and migrate via the essential process of spreading metastatic cells which is called epithelial-mesenchymal transition (EMT) [15]. The vital role of EMT is the formation of a body plan and tissue repair. The EMT process is the downregulation of epithelial cell features and activation of mesenchymal cell characters. In the early stage of life, this is a normal reversible process with mesenchymal-epithelial transition (MET) and cells can show epithelial phenotype again [16]. In development, EMT is an integral part of growth and plays a role by starting with the generation of mesoderm. Both EMT and MET, as a natural process, happen in the diverse cell types and tissue formation in the body but by developing the body it will be decreased [17]. In cancer, EMT is reactivated and provides a suitable condition for invading tissues [18]. The EMT process is initiated by the reconstruction of epithelial cell-cell junctions and apical-basal polarity. These changes enable the cancer cells to form a new front-rear polarity which is essential for directing the migration. Subsequently, other alterations in membrane extrusions and cell adhesion an effective feature for increasing the motility of cells. The EMT and MTE processes are shown and their function in both natural development and cancer is depicted (Figure 1).
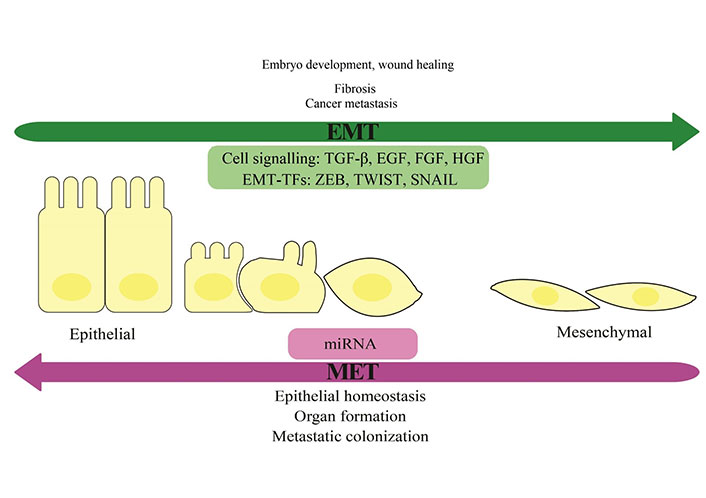
EMT and MET process. The EMT process is the loss of epithelial cells’ polarity and cell-cell adhesion, and the gain of migratory and invasive features which change these cells to mesenchymal stem cells. The reversible process is MET. There are some signaling pathways and transcription factors (TFs) in this way. Some microRNAs (miRNAs) can suppress the expression of EMT transcription factors and they can regulate EMT and MET. The role of EMT and MET in normal and abnormal processes of the body was shown. TGF-β: transforming growth factor beta; EGF: epidermal growth factor; FGF: fibroblast growth factor; HGF: hepatocyte growth factor; ZEB: zinc-finger E-box-binding; SNAIL: zinc finger protein SNAI1
Alteration in gene expression is a key factor in the EMT and gene reprogramming which are associated with this mechanism act as key transcription factors in the trans differentiation process. Changing in gene expression can provide differences in the messenger RNA (mRNA) splicing patterns and the formation of new transcripts are facilitated by alterations in the expression of splicing factors. Some signaling molecules and transcription factors such as TGF-β, EGF, FGF, HGF, ZEB, TWIST, and SNAIL can increase the EMT (Figure 1). Furthermore, some miRNAs can suppress the expression of EMT transcription factors and they can regulate EMT and MET through various mechanisms that are controlled by functional feedback (Figure 1). In addition, TGF-β family proteins can induce EMT by the small mothers against decapentaplegic (SMAD)-mediated activation of EMT transcription factor expression, and subsequently their transcription activities can be controlled by SMAD-mediated mechanisms. Signaling pathways that play a role in the induction and progression of EMT can be activated by TGF-β family proteins with mediating of complementary non-SMAD [19].
EMT, like most metastases, has a special importance and function in brain metastasis. The impacts of EMT on the metastatic process and establishing metastatic masses need more investigation but several studies showed the metastases of different cancers to the brain via the active role of EMT in this incidence. For example, results of a study in breast cancer brain metastasis (BCBM) which used intravital microscopy for screening of mRNA sequencing, showed that cells that are involved in EMT play a role in creating BCBM and invading the brain parenchyma. Furthermore, they have the acceptable capacity to go back to the epithelial status after forming secondary lesions in the brain [20].
Interaction of metastatic cells via tumor stroma
During cancer progression, paracrine signaling active cells in the adjacent stroma. [17]. In this condition, endothelial cells, fibroblasts, pericytes, and leukocytes with pro-tumorigenic elements maintain the growth of tumors. In this case, two types of cells such as cancer-associated fibroblasts (CAF) and the pericytes are the most prominent cells in brain metastasis. CAFs produce different factors to facilitate cancer cell migration and angiogenesis, especially in breast cancer metastasis into the brain. CAFs express high amounts of molecules like TGF-β, HGF, EGF, cytokines like stromal-derived factor- (SDF-), FGF, interleukin 6 (IL-6) and canonical wingless-related integration site (Wnt) families in brain metastasis progression [21]. Furthermore, some inflammatory mediators can stimulate CAFs and mediate the production of vascular endothelial growth factor (VEGF), FGF-2 which recruits endothelial progenitor cells and raises the angiogenesis process [22]. Researchers discovered that in cancer cells releasing macrophage-colony stimulating factor (CSF-1) can be seen. This event stimulates macrophages which are involved in the tumor microenvironment to release EGF to promote tumor proliferation in this stroma [3, 23].
Local invasion of metastatic cells
By developing a metastatic clone of cancer cells, there are two steps for spreading the tumor. At first, invasion of the ECM will occur in penetration into the vasculature and hematogenous dissemination of metastatic cells to the CNS. In the tumor expansion phase, leading ECM compression and modifying the flow of blood and lymphatic vessel flow will occur and subsequently it can cause the BM to thin. This is associated with other molecular and cellular events and leads to metastasis. In brain metastasis, there are molecular changes in the basal lamina of the microvessels and evidence indicates that the permeability of blood vessels increases. For instance, agrin is a component of the basal lamina of BBB microvessels and important for the maintenance of the BBB, is absent from basal lamina of tumor vessels. It facilitates the metastasis of cancer cells into the brain [24]. After penetrating tumor cells into BM, they can enter the circulation and overpass through the ECM and the vascular BM (VBM) [22, 25].
Role of E-cadherin-catenin complex and integrin
Cell-cell adhesion and the maintenance of proper tissue architecture in the body are carried out by transmembrane glycoproteins called cadherins [24]. Cell-cell adhesion, often through adherent junctions, is necessary for the maintenance of cellular and tissue morphogenesis in multicellular organisms. Cell adhesion between cells and between cells and the ECM mediates cell signaling and gene regulation in normal tissue homeostasis [25–28]. The cytoplasmic domain of catenin proteins is where the traditional cadherins, a type of adhesion molecules, engage. These traditional cadherins are categorized into three subclasses within the tree: neural (N), placental (P), and epithelial (E) [29]. In addition to being present in bodily fluids, cadherins have also been discovered in between tumor cells [30]. Numerous cancers, including melanoma, glioblastoma, pancreatic cancer, hepatocellular carcinoma, and breast, and gastric cancers, have been shown to include cadherins in the process and development of cancer [31, 32]. Numerous investigations have revealed how E-cadherin promotes cell motility, invasion, and tumor growth during malignancy in the late stages of cancer [33, 34].
Research findings suggest that aberrant expression of E-cadherin may be observed during the invasion of ductal carcinoma, which may be linked to distant locations metastasis [35]. Re-expression of the E-cadherin protein in malignant cells aids in the spread of the protein to distant places, and it is more common in distant tumor metastases than in the original malignancy. Consequently, the expression or repression of E-cadherin in cancer invasion of distant metastases suggests that it could play a major role in the development of metastatic foci [25].
Numerous factors, including TGF-β, HGF, and epithelial growth factor, downregulate E-cadherin-mediated cell adhesion; conversely, EMT-related transcriptional factors, including SNAIL, slug, and TWIST, are upregulated [26]. Furthermore, by decreasing cell-cell adhesion and inhibiting oncogenic stimulation through β-catenin, this downregulation activates the Wnt signaling pathway, which in turn activates T-cell factor (TCF)-regulated genes. TCF-regulated genes are essential for regulating other important factors in cell invasion and proliferation, such as c-MYC, cyclin D1 (CCND1), matrix metalloproteinase-7 (MMP-7), MMP-14, fibronectin 1 (FN1), leucine-rich repeat-containing G protein-coupled receptor 5 (LGR5), plasminogen activator urokinase receptor (PLAUR), etc. [27, 28]. N-cadherin expression levels change in brain cancer. Study results indicated that in various patient cohorts, N-cadherin expression levels were changed. In glioma, this level show increase. There is a relation between E-cadherin expression and the potential of proliferation in brain metastatic tumors and this role depends on the tumor’s location before the metastasizing into the brain [29].
Genetic alterations
In cancer cell invasion and metastasis, genetic alterations in tumor suppressor genes (TSGs) and promoter genes have significant roles, and several mutations are known in this process. In brain cancer, activation or inactivation of both promoter and suppressor genes originate from mutations, multiplication translocation, deletions, and loss of heterozygosity [22]. There are famous mutations in TSGs, which are important in migration and intravasation. The kisspeptin-1 (KiSS1) gene, which produces the protein metastin, is considered as one example. It is a ligand for the receptor hOT7T175—an orphan G protein pair [30]. Studies showed that suppressing the expression of KiSS1 can prevent the spread of melanoma and breast cancer. However, there is a contrary relationship between the expression of KiSS1 and the advancement of melanoma [31]. KAI1 (CD82) is another TSG that controls the migration, proliferation, and differentiation of cancer cells and has an inverse relationship with different cancer stages [32]. Association of KAI1 with EGF receptor (EGFR) can influence the Rho family of small GTPases (Rho GTPase) pathway and simulate migration in brain metastasis [3, 22, 33]. Furthermore, in the malignancy process mutations in EGFR and TP53 remain. These mutations propose that activation of oncogene EGFR and disrupting function of tumor suppressor TP53 may have a role in brain metastases. In addition, observing the mutations in these two genes in the primary tumors are sufficient for decision-making in clinical treatment [34]. Other mutations such as nuclear factor erythroid 2-related factor 2 (Nrf2), rat sarcoma (RAS), phosphatidylinositol-4, 5-bisphosphate 3-kinase catalytic subunit alpha (PIK3CA), and phosphatase and tensin homolog (PTEN) are reported in brain metastasis [35]. In addition, there are several promoter genes responsible for invasion and metastasis in the brain. For instance, TWIST1-positive LBC cells with estrogen receptors α (ERα) might inhibit forkhead box protein A1 (Foxa1) expression. Foxa1 expression is necessary to release Foxa1-arrested migration and invasion during cancerous breast cell metastasis [36].
Dissemination
During metastatic progression, cancer cells with different origins after breaching their microenvironment must be able to survive in stressful environment. Cancer cells adhere to endothelium of target tissue penetrating to them via cell-cell junctions. In this stage cancer cells are in front of the immune system for protecting the cells against cancer cells. Recruited cancer cells from the immune system can colonize, extravasation and proliferation in this environment. The disseminated cancer cells show significant genomic heterogeneity that is gradually reduced by expansion in different metastatic niches and it allows divers metastatic clones to adapt to the new microenvironments [3, 37].
Brain microenvironment and BBB
The BBB are capillary endothelial cells with a tight junction which are associated with BM, pericytes, and astrocytes and the other cells in formation of BBB. This structure provides a barrier with no penetrance for macromolecules and peptides and low amounts for small molecules and ions. The BBB regulates the transfer of chemicals and solutes between the circulatory system and the CNS and its unique structure protect the CNS against pathogens. In brain metastases, tumor cells invade BBB and increased permeability of tumor-associated endothelial cells. In brain microenvironment, glial cells impact brain and BBB integrity by creating structural support for neurons. Microglia cell type in cerebral vessels empower BBB damage throughout neuroinflammation. However, they have positive impacts in front of ischemic brain injury and studies indicated that microglia and macrophages secrete some factors which are helpful in angiogenesis including growth factors, cytokines, enzymes, tumor proliferation, and VEGF. In metastatic brain tumors, the BBB integrity and structure is altered and it being more permeable by reduction in the expression of tight junctions. The origin and stage of tumor in metastatic cells can influence the BBB disruption. Increasing in VEGF expression from the tumor cells and angiogenesis in brain disrupt the structure of BBB [3, 38].
Ion channels
Cancer cells are be potential with changes in ion channels which are different from their parental cells. Some of these channels with oncogenic potential may be selectively over-expressed in cancer cells which are named as oncochannels. In plasma and intracellular membranes of cancer cells, oncochannels facilitate malignant progression, metastasis and drug resistance in treatment [39–41]. The most important types of ion channels which play a role in brain cancer can be seen in Figure 2.
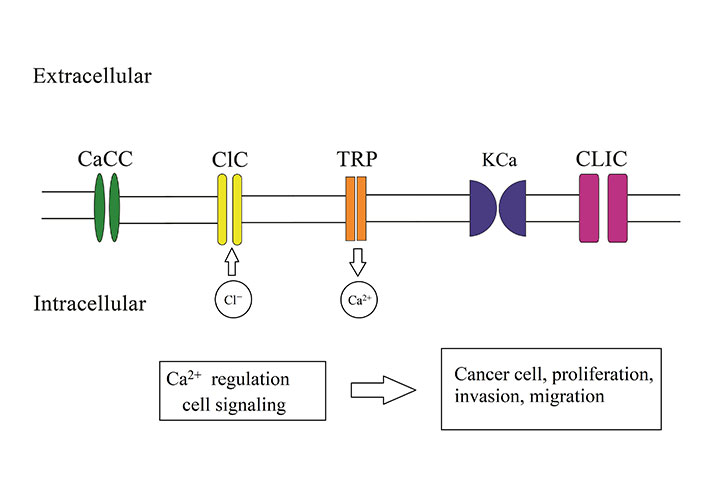
The most important ion channel in brain tumor proliferation, migration, and invasion. Calcium ion (Ca2+)-activated chloride ion (Cl−) channels (CaCC), voltage-gated Cl− channel family (ClC) proteins, the transient receptor protein (TRP), Ca2+-activated potassium ion (K+) channels, Cl− intracellular channel (CLIC) proteins
The first stage of metastasis, known as EMT, is regulated by the plasma membrane and intracellular ion channels in cancer cells that have a high propensity for brain metastasis, such as lung and breast cancer. For example, EGF-induced EMT requires the activation of the Ca2+-permeable two-pore segment channel-2 (TPC2) in the endolysomal membrane Ca2+ storage [42]. Research findings indicate that EMT and metastatic activity in lung and breast cancer cell lines can be induced by the alpha-7 nicotinic receptor (α7 nAChR), a Ca2+-permeable cation channel [41, 43].
In glioma, for migration and invadining brain tissue, changing in cell volume charge is a key factor which is provided by ion channels activity. Ca2+ and Cl– fluxes change the osmotic condition and TRP, and Ca2+-activated K+ (KCa) channels play a role for this change. Through the TRP channels, Ca2+ influx increase that is essential for invasion of cells. Moreover, this Ca2+ influx can lead to efflux of Cl– and cell migration. Results of studied indicated that Cl– channels have important role in brain cancer malignancy and they play role in proliferation, migration and invasion of cancer cells and metastasis. The overexpression of ClC proteins and CLIC are reported in brain cancer cells. CaCC have a fundamental role in glioma formation and mediate brain tumor functions. Depict the role of these channel in brain cancer (Figure 2).
Research indicates that K+ and Cl– channels play a role in gliomas and primary brain cancers. These channels produce an increase in cell invasion and the production of brain metastases by enhancing their coordinated activity. Furthermore, colocalization of cells’ Ca2+-activated K+ channels with ClC-3 Cl– channels may aid in the tumor’s invading operations. The leading process may decrease quickly as a result of the simultaneous release of Cl– and K+ ions together with required water when intracellular Ca2+ levels rise and these channels are activated. Cell invasion into the extracellular brain spaces is accelerated by this circumstance. The NKCC1 cotransporter, Na-K-2Cl, builds up intracellular Cl– to unusually high concentrations, which causes a directed gradient of Cl– ions to exit the cells. Through this process, Cl can be used by glioma cells as an osmotically active anion during invasion. It is evident that blockage of Cl– channels limits tumor cell invasion by delaying changes in cell volume. These findings validated the clinical assessment of the Cl– channel blocking peptide chlorotoxin in patients with malignant glioma. This survey’s clinical trial data demonstrated chlorotoxin tumor selectivity. A phase II clinical trial is now being conducted to study this treatment. It has been suggested that K+ and Cl– channels play a similar role in other metastatic malignancies, which might open up new avenues for the development of cutting-edge ion channel-targeting treatments [44, 45].
Treatment
Patients’ survival with brain metastases was insignificant in the past and they were treated for palliative purposes especially whole-brain radiotherapy (WBRT) and most effective treatments were surgery and stereotactic radiosurgery (SRS) [28]. By improving an understanding of tumor biology and molecular mechanisms in this process, new treatments appeared. Moreover, the improvement of cytotoxic agents and targeted therapies improved BBB penetration which is helpful in treatment. Treatments use in brain cancer and their features are summarized in Table 1.
Treatments and features
Treatment | Benefits | Negative consequences |
---|---|---|
Surgery | Removal of a brain metastasis, immediate elimination of life-threatening or symptom-generating mass effects and elimination of the source of perifocal edema, a survival benefit | Surgery risk and problems, surgical technique for detecting distinct tumor size, location |
WBRT | Improved survival | Hair loss, fatigue, headache, skin erythema, and serous otitis media |
SRS | Improved survival | Nausea, vomiting, fatigue |
Chemotherapy | Live longer, reducing cancer symptoms | Fatigue, hair loss, loss of appetite, nausea, skin and nail problems bowel issues such as constipation or diarrhea, mouth sores |
Targeting responsible molecular mechanisms | Minimizing the impact of side effects, live longer, reducing cancer symptoms, improves a patient’s response to treatment | Interventions with normal functions |
Nano drug | Minimizing the impact of side effects, improves a patient’s response to treatment, passing through the BBB more effectively | Designing |
Treatment by targeting molecular mechanisms
Treatment outcomes are improving when the molecular pathways causing metastasis are targeted. Some of these molecular mechanisms and medicines are summarized in Table 2. Treatment and management of brain metastasis greatly benefit from targeting various factors, including human EGFR2-positive (HER2+) breast cancer, EGFR-mutated, anaplastic lymphoma kinase (ALK)-translocated non-small cell lung cancer (NSCLC), and certain melanomas [46]. Researchers have looked at brain metastases and the consequences of lapatinib-based therapy in patients with HER2+ breast cancer. Lapatinib and capecitabine combined therapy improves a patient’s response to treatment for brain metastases [47]. Patients with lung cancer who do not have both an ALK rearrangement and an EGFR mutation and who have metastases in the CNS respond well to immunotherapy. Using this technique, an antibody is used to inhibit the programmed death receptor 1 (PD-1) on the surface of T or B cells. A novel class of medications called small-molecule tyrosine kinase inhibitors (TKI) has been developed for patients with non-small cell lung carcinoma and CNS metastases who have an activating mutation on EGFR that causes cancer cell proliferation [48].
Treatment by targeting molecular mechanisms
Medicine | Cancer | Molecular mechanism target |
---|---|---|
Pembrolizumab | CNS metastases from NSCLC | PD-1 |
Lapatinib | HER2+ breast cancer | TKI activity associated with EGFR and HER2 |
Combination of lapatinib and capecitabine | Brain metastases | TKI activity associated with EGFR and HER2, inhibiting the synthesis of thymidine monophosphate |
Afatinib, crizotinib, gefitinib, and erlotinib | Lung cancer and brain metastasis | ALK inhibitors |
Osimertinib and ceritinib | Lung cancer and brain metastasis | Tyrosine kinase |
Dabrafenib and vemurafenib | Metastatic melanoma | BRAF inhibitors |
Pembrolizumab (PD-1 inhibitor) is used in the treatment of individuals with CNS metastases from NSCLC. These individuals have mutations that activate the expression of the EGFR-exon 19 (deletion), exon 21 (point mutation L858R), and exon 20 (mutation), which are visible and promote the growth of cancer cells by blocking apoptosis, signal transduction, invasion, and angiogenesis. Treatment outcomes can be greatly enhanced by focusing on this gene and associated pathways. Additionally, the echinoderm microtubule-associated protein-like 4 (EML4)/ALK fusion gene is seen in individuals with lung cancer. This fusion gene prevents cancer cells from undergoing apoptosis and activates the intracellular signaling system. The class of newly discovered medications known as small-molecule TKI includes the ALK inhibitors crizotinib, gefitinib, and erlotinib. Further research led to the development of second-generation TKI, such as afatinib or the ALK inhibitor alectinib, which permanently blocks the receptor. Clinical research showed that administering TKI to individuals with CNS metastases from NSCLC enhanced their prognosis and lengthened their survival. Osimertinib and ceritinib were used with superior outcomes for patients who had become resistant to treatment during TK1 therapy [49].
Research has shown that using BRAF inhibitors, such as dabrafenib and vemurafenib, as a single drug improves both the course of therapy and the prognosis for patients with metastatic melanoma [50].
Nano-drug in cancer treatment
Nanotechnology is the study of materials in the nano (10−9) size range and applies this feature to create or modify novel objects. Manipulating structures at the atomic scale provides nanomaterials with electrical, optical, and magnetic behavior which are useful in different fields [51]. Application of nanotechnology in medicine, nanomedicine, focuses on the prevention, diagnosis, and treatment of different diseases. Using this technology in targeted drug delivery system (TDDS) can deliver therapeutic drugs safely and directly to the target and this is important in oncology because of the various benefits of TDDS including preventing the side effects and drug degradation, reducing toxicity to normal cells, increasing drug distribution in tumor and accumulating of the drug in cancer cells. In recent years, nanomedicines have significant development for cancer treatment especially with drug delivery systems (DDSs) for delivering anticancer drugs to the tumor cells [52].
For example, using hyaluronidase-activated prodrug hyaluronic-doxorubicin (hDOX) which was assembled with dual-targeting nanoparticles (NPs) in metastatic breast cancer showed the ability to dually targeting the BBB and metastatic breast cancer. hDOX improved the median survival time of mice with metastatic breast cancer. This new strategy for drug delivery is important and helpful in brain metastases treatment [53]. Several studies showed that in HER2+ breast cancer brain metastases with EGFR and HER2 over-expression using monoclonal antibodies with a cytostatic activity can interfere with the transduction pathways of EGFR and active cytotoxic activity via the immune system activation with the antibody-dependent cell-mediated cytotoxicity (ADCC). By designing a nanovector with a recombinant form of human apoferritin (HFn) which promotes the delivery of monoclonal antibodies (mAbs) to the brain for the activation of the ADCC response. In this system, without affecting the antitumoral activity of mAbs, results indicated that using HFn as an efficient carrier can enhance the BBB crossing [54]. In patients with metastatic HER2+ breast cancer, trastuzumab is a highly effective drug; however, due to its high molecular weight, it has low BBB penetration [55]. The use of nanotrastuzumab against HER2+ mice showed an overall higher survival than the control group. Results of studies clear that the covering lapatinib within liposomal nano-particles could easily cross the BBB and accumulate in BCBM patients. Thus, NPs are the potential therapeutic drug to change the landscape of the treatment in BCBM for HER2+ breast cancer patients [55, 56].
Targeting ion channels in cancer treatment and brain tumor
Ion channels are crucial modulators of the pathophysiology of cancer cells. They support a variety of processes that lead to tumor growth and metastasis, including signal transduction, transcriptional activity, maintaining cellular osmolarity and membrane potential, invasion, motility, and cell cycle progression. Ion channels are therefore possible targets for cancer treatment. Ion channels, however, are found in both cancerous and normal cells, and their functionality in normal cells may be impacted by the use of certain blockers [57]. It is advantageous to have new methods that can target ion channels and aid in medication delivery. Furthermore, anticancer medicines delivered to specific tumors may boost their effectiveness and reduce the negative effects of conventional chemotherapy. Liposomes, pro-apoptotic peptides, oligonucleotides, radionuclides, and NPs are a few strategies for directly targeting cancer cells [58].
Calcium channel inhibitors such as diltiazem and verapamil were found to decrease calcium influx and EGFR-dependent invasion pathways in an in vitro investigation of squamous cell carcinoma [59]. Researchers are drawn to the novel and intriguing topic of studying ion channel targeting in brain metastases. According to a study, the EAG2 potassium channel is involved in the development and metastasis of brain tumors as well as the enhanced migration of MB cells. Thioridazine is a possible target for ion channels in cancer therapy since it decreases xenografted MB growth and metastasis in these patients when used as an EAG2 channel blocker [60]. In recent investigations, the use of pharmacological modulators of ion channels and types of aquaporins (AQPs) has been shown to reduce the migration and invasion of glioblastoma cancer cell models. Furthermore, in vitro studies using glioblastoma models showed that pharmacological inhibitors of voltage-gated sodium (NaV), voltage-gated potassium (KV), and voltage-gated calcium (CaV) channels were linked with decreased migration, cell survival, and invasion. In glioblastoma cell lines, blocking KV channels with the scorpion toxin KAaH1 reduced cell migration [61].
Targeting ion channels by nano-drug affecting treatment in the future
Ion channels have critical roles in cancer progress such as invasion, migration, angiogenesis, and cell proliferation. However, they have significant functions in normal cells and physiological cell behaviors. Targeting ion channels in cancer treatment is attractive and effective but their functions in normal cells should be considered and blocking their abilities have enormous side effects too. Targeting them directly not only increases the efficiency of treatment but also minimizes unfavorable outcomes. The use of nanomedicines and new methods in drug delivery can be a breakthrough in this field. By designing specific nanomedicines for ion channels involved in brain cancer, the activity of these products can be controlled to reduce or increase performance. In addition, the effectiveness of nanomedicine can be limited to cancer cells in the brain. As we know, due to the presence of the BBB, the passage of most chemotherapy drugs to the brain is very limited, and this creates a great challenge in the treatment of brain cancer. By using nano-drugs, due to their small size and easier passage through the BBB, this problem will be solved to a great extent. Moreover, we propose focusing on nano-drug designing which are targeted ion channels in brain metastasis may help to overcome the difficulties created by BBB and overlap with normal cell functions of treatment in patients with this cancer (Figure 3).
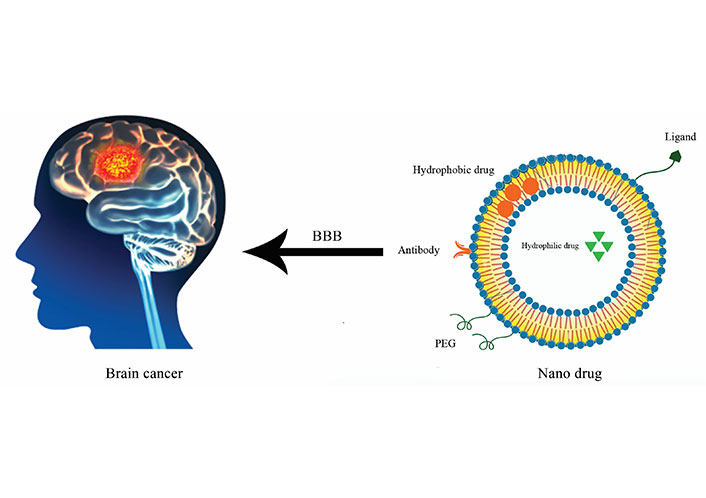
Brain cancer treatment by nano drug. Targeting brain cancer and ion channels by designing nano drug which can carry antibodies, hydrophobic and hydrophilic drugs, ligands and polyethylene glycol (PEG) for acting specificly and passaging through the BBB more easily
Conclusions
The metastatic mechanism is a complex and multi-step process which is involving in brain metastasis including proliferation, EMT, invasion and cancer cell progression, cell-cell adhesion, genetic alterations, and ion channels. The investigation of the process of metastatic disease may aid in the diagnosis and treatment of brain metastases. Learning about the main proliferative stage might help you avoid the onset of the metastatic phase. Ion channels are involved in the genesis and neoplastic evolution of brain tumors because they regulate electrical signaling, membrane potential, and chemical movement in normal cells.
Studying nanomedicine and nano delivery systems for cancer therapy is appealing due to their high efficacy and ability to target cancer cells selectively. Chemotherapy treatment for brain metastases is limited because of the BBB and the surrounding milieu. Small-sized nanodrugs can penetrate the BBB and improve treatment outcomes. Developing a nanodrug to regulate ion channels implicated in brain metastasis has the potential to transform cancer therapy and is an appealing avenue for further investigation.
Abbreviations
ALK: | anaplastic lymphoma kinase |
BBB: | blood-brain barrier |
BCBM: | breast cancer brain metastasis |
Bcl-2: | B cell lymphoma-2 |
BM: | basement membrane |
Ca2+: | calcium ion |
CaCC: | calcium ion-activated chloride ion channels |
CAF: | cancer-associated fibroblasts |
Cl–: | chloride ion |
ClC: | voltage-gated chloride ion channel family |
CLIC: | chloride ion intracellular channel |
CNS: | central nervous system |
ECM: | extracellular matrix |
EGF: | epidermal growth factor |
EGFR: | epidermal growth factor receptor |
EMT: | epithelial-mesenchymal transition |
FGF: | fibroblast growth factor |
Foxa1: | forkhead box protein A1 |
HER2+: | human epidermal growth factor receptor 2-positive |
HGF: | hepatocyte growth factor |
K+: | potassium ion |
KiSS1: | kisspeptin-1 |
MET: | mesenchymal-epithelial transition |
miRNAs: | microRNAs |
NPs: | nanoparticles |
NSCLC: | non-small cell lung cancer |
SMAD: | small mothers against decapentaplegic |
SNAIL: | zinc finger protein SNAI1 |
TGF-β: | transforming growth factor-β |
TKI: | tyrosine kinase inhibitors |
TRP: | transient receptor protein |
TSGs: | tumor suppressor genes |
VEGF: | vascular endothelial growth factor |
ZEB: | zinc-finger E-box-binding |
Declarations
Author contributions
ZKD: Conceptualization, Investigation, Writing—original draft, Writing—review & editing. The author read and approved the submitted version.
Conflicts of interest
The author declares that there are no conflicts of interest.
Ethical approval
Not applicable.
Consent to participate
Not applicable.
Consent to publication
Not applicable.
Availability of data and materials
Not applicable.
Funding
Not applicable.
Copyright
© The Author(s) 2024.