Abstract
The emergence and re-emergence of pathogens is a public-health concern, which has become more evident after the coronavirus disease 2019 (COVID-19) pandemic and the monkeypox outbreaks in early 2022. Given that vaccines are the more effective and affordable tools to control infectious diseases, the authors reviewed two heterologous effects of vaccines: the trained immunity and the cross-reactivity. Trained immunity, provided by attenuated vaccines, was exemplified in this article by the decreased the burden of COVID-19 in populations with high Bacille Calmette-Guerin (BCG) coverage. Cross-reactive responses were exemplified here by the studies which suggested that vaccinia could help controlling the monkeypox outbreak, because of common epitopes shared by orthopoxviruses. Although modern vaccination is likely to use subunit vaccines, the authors discussed how adjuvants might be the key to induce trained immunity and improve cross-reactive responses, ensuring that heterologous effects would improve the vaccine’s response.
Keywords
Coronavirus disease 2019, monkeypox, trained-immunity, cross-immunity, adjuvantsIntroduction
The emergence and re-emergence of pathogens is an important problem for public health. The current list of the World Health Organization points the recent coronavirus disease (COVID) pandemic and the outbreaks of Ebola and monkeypox as public health emergencies [1]. Several factors explain the emergence and re-emergence of pathogens; however, some of them are unlikely to change—as the globalization and the environmental changes already promoted by men, suggesting that the health systems should adapt and respond to such problems [2–4].
Another issue that plays an important role in the re-emergence of pathogens is the low vaccine coverage. Recently, polio cases have emerged after years of elimination of the disease in the United States [5], Israel, and Ukraine [6]; thus, there is a serious risk of re-emergence of poliomyelitis in other countries, such as Brazil and Venezuela [7, 8]. Other examples of immune-preventable diseases which reemerged after the decline of vaccinations are measles, mumps [9], and the yellow fever [10].
During the COVID pandemic, different authors described how the prevalence of the disease was reduced in populations with high coverage rates of the Bacille Calmette-Guerin (BCG) vaccine [11]; and the same was observed for influenza, lower respiratory infections, and overall children mortality [12]. The most accepted explanation is the trained immunity, which would improve the immune response to different pathogens [13]. Meanwhile, after monkeypox emergency, some studies reviewed the potential efficacy of vaccinia to protect against the pathogen, based on cross-reaction between orthopoxviruses [14, 15]. Trained immunity and cross-reactivity are heterologous benefits of vaccines—that means, they confer some level of protection apart from the pathogen-specific protection [16].
Here, we summarize how these two different heterologous effects of vaccines may contribute to fight infectious diseases, using the recent examples of COVID-19 for trained-immunity and monkeypox for cross-reactivity. In addition, we discuss how new, modern vaccines could achieve similar effects: even though subunit differ from the attenuated vaccines according to immunogenicity, trained-immunity induction and cross-reactivity potential, adjuvants based on pathogen-associated molecular patterns (PAMPs), recognized by pattern-recognition receptors (PRRs), could be explored for improving the heterologous benefits. The chart below illustrates these points, which will be reviewed in this article (Figure 1).
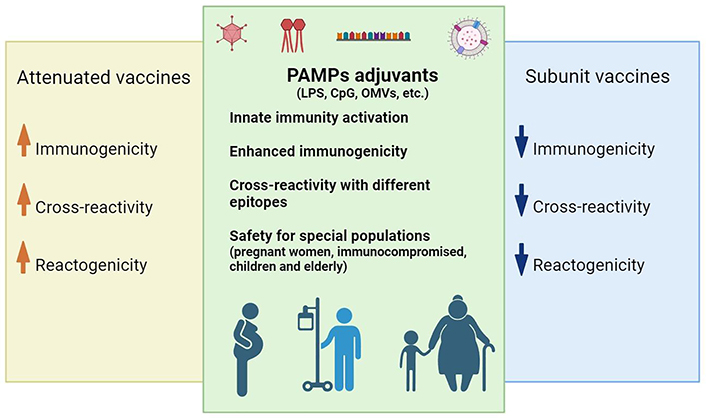
Contributions of PAMPs adjuvants to subunit vaccines. While subunit vaccines are safer, presenting less reactogenicity, attenuated vaccines are more immunogenic and likely to enhance cross-reactivity (because there are more antigens available) and trained-immunity (because of PRRs recognition). PAMPs, such as outer membrane vesicles (OMVs) from Gram negative bacteria, lipopolysaccharide (LPS), and other PRR agonists activate the innate response, similarly to attenuated vaccines, potentially working on trained immunity; thus, they might confer cross-reaction because they are expressed by different pathogens. Finally, they enhance the immunogenicity of subunit antigens, which are safer and preferable for special populations, such as pregnant women, immunosuppressed patients, children, and the elderly. CpG: cytosine-phosphate-guanine
Trained immunity benefits
Trained immunity is defined as the functional reprogramming of innate cells in response to PRRs unspecific stimuli. Trained immunity happens on a long-term basis and allows the immune system to respond with more or less intensity, adapting itself to the context and reacting adequately upon a second stimuli [17]. As it is known, the innate activates the adaptative immunity, secreting cytokines and chemokines to recruit effector cells and modulate the overall response [18]. This type of “memory” of innate cells is crucial for the individual homeostasis, establishing patterns for activation or tolerance [19]. Considering anti-viral response, trained immunity results in increased receptors expression, enhanced phagocytic and killing capacity and production and secretion of adequate cytokines for anti-viral response [20].
Certain live-attenuated vaccines were shown to induce heterologous effect, which has been attributed to trained immunity: smallpox-vaccinees were less likely to be hospitalized by different infectious diseases [21]; measles and yellow fever immunization were associated with reduced carriage of Haemophilus influenzae and pneumococci [22]; administration of measles-mumps-rubella (MMR) vaccine was associated with reduced hospitalization [23] and combined vaccination with oral polio and BCG resulted in decreased children mortality [24]. BCG has been extensively studied for its heterologous effects, which are the reduced prevalence of other mycobacterium infections, as leprosy [25], prevention of hospitalization by respiratory infections and sepsis [26], infant survival [27], protection against yellow fever attenuated virus [28] and, more recently, reduced COVID-19 fatal cases [29].
The trained immunity phenomena highlight how vaccines present several benefits, contributing to non-specific protection from different pathogens and regulation of tolerance mechanisms [16]. Unfortunately, the increasing anti-vaccine movements and the decay in vaccine coverage in various countries are preventing people from obtaining these benefits and, during the pandemic, the problem worsened [30–32].
Cross-reactive responses
Vaccinia is an orthopoxvirus, which had been used for attenuated vaccines that allowed the eradication of smallpox. As soon as the monkeypox outbreak started, there were questioning about the efficacy of vaccinia to prevent the infection [33]. However, it should be noted that, after smallpox eradication, the production of such vaccines became limited: now, there are modified vaccinia Ankara (MVA), nonreplicating; ACAM, replicant competent and LC16, minimally replicating [34]. Bioinformatics analysis predicted that cross-reactivity was likely to happen because of similar sequences in vaccinia and monkeypox viruses [35].
Immunization with vaccinia induces a broad response characterized by neutralizing antibodies with different specificities, which supports the cross-reactive response for different poxviruses [36]. A study found memory B cells in volunteers vaccinated with vaccinia virus over 40 years ago and, even though these cells were rare, the group managed to characterize a monoclonal antibody which was promising as therapeutic and prophylactic treatment against poxviruses [37]. In addition, a serologic investigation verified circulating neutralizing antibodies in 33–53% of people older than 45 years, who were likely to have been vaccinated against smallpox [38].
It was described that neutralizing antibodies correlate with protection against poxvirus [39, 40]; however, CD4+ cells are just as important, provided that they support antibody secretion, seem to be longer-lived than CD8+ cells and major histocompatibility complex (MHC)-II epitopes are likely to present cross-reaction with different poxviruses [40–42]. Moreover, animal studies highlighted previously that cytotoxic response triggered by vaccinia seems to be related to the administration site: scarification would confer a better CD8+ response, because of skin-resident T cells, which would be better than injection for protection upon dermal challenge [43, 44]. Furthermore, mice studies found that vaccinia inoculation induced trained natural killer (NK) cells, which protected the animals depleted from T and B lymphocytes from viral challenge, suggesting how vaccinia immunization might result in polarization of different arms of the immune response, which are likely to protect the recipients [45].
From human, real-world evidence, an observational study estimated the vaccinia efficacy against monkeypox to be 79%, although it has not been peer-reviewed yet [46]. A study conducted 5 years after discontinuation of smallpox vaccination predicted that the population would be 85% protected against monkeypox [47]. However, as Fine and collaborators [47] highlighted in the manuscript, the waning immunity over the years is likely to decrease this level of protection. Indeed, a serological survey which comprised European, South American, Asian, and African samples verified low neutralizing antibodies in people vaccinated decades before, as well as in unvaccinated volunteers [48]. Another study found 400 monkeypox cases in 7,339 vaccinees who received one dose of MVA, highlighting that the two-dose regimen is required for better efficacy [49]. On the other hand, another study found that when unvaccinated people are compared to people vaccinated with one dose of a non-replicant vaccinia virus, they present a 7.4 higher risk of getting infected by monkeypox; when compared to people vaccinated with two doses, the risk increases to 9.6—showing that even one dose can confer some level of protection, what might be important to respond to outbreaks, when there is a limited vaccine supply [50]. Altogether, these studies support the importance of studying immunization strategies to control orthopoxviruses outbreaks, whether using existing smallpox vaccines or searching new ones.
To note, immunosuppressed patients and pregnant women present poorer outcomes to monkeypox infection [33] and, during the COVID-19 pandemic, it was pointed out how these groups were underrepresented in clinical trials, hampering the vaccination policies for these populations [51]. All that considered, it would be important investigate subunit vaccines, which are safer options for these groups [52]. Cross-reactive epitopes of vaccinia virus were identified previously [42, 53], intranasal-delivery of recombinant vaccinia antigen was promising for mucosal protection [54] and, compared to DNA, recombinant proteins were more immunogenic and protective [55]. Despite the lack of studies comparing protein antigens to the attenuated virus, the search for subunit candidates for poxviruses vaccines should be encouraged.
Could subunit vaccines mimic trained-immunity and enhance cross-reactivity?
Attenuated and inactivated vaccines present several PAMPs, which are likely to promote trained immunity, consequently conferring heterologous effects for these vaccines [16, 56]. Even though subunit vaccines are safer, the scientific community can explore the trained immunity benefits of PAMPs investigating them as adjuvants [19, 52]. In addition to enhanced immunogenicity and targeting of trained immunity, PAMPs are affordable options for developing countries, allowing them to establish their own manufacturing, consequentially improving vaccine coverage [57, 58].
Excellent revisions described the benefits of PAMPs as adjuvants [57, 59], some of them focused on COVID-19 [60, 61]. The combination of PAMPs or chemically synthetized PRRs agonists to other adjuvants and delivery systems is another strategy which has been studied, not only to enhance immunogenicity but also to modulate the immune response [59].
Scaria et al. [62] observed a T helper 1 (Th1)-biased immune response when conjugating the receptor binding domain (RBD) of severe acute respiratory syndrome coronavirus 2 (SARS-CoV-2) to detoxified diphtheria toxin (EcoCRM®) and Adjuvant System (AS) 01 [a liposome-based adjuvant which contains monophosphoryl lipid A (MPL), a toll-like receptor (TLR)-4 agonist, and the saponin QS-21]. The quantity and functionality of antibodies was robust even when a low antigen concentration was used. In Jangra et al. [63], the subunit 1 of spike SARS-CoV-2 protein was complexed with a nanoemulsion containing in vitro transcribed (IVT) messenger RNA (mRNA) adjuvant, a retinoic acid-inducible gene I (RIG-I) agonist, and induced functional antibodies, which could neutralize other SARS-CoV-2 variants and protect naive mice from virus challenge. The L1 envelope protein of vaccinia adsorbed in alum induced the higher neutralization percentage when combined with CpG, a TLR-9 agonist [64]. Although most studies exploring adjuvants combinations use animal models, the COVID-19 vaccine Soberana 01 is on clinical trials. It is composed by recombinant RBD adsorbed in alum and adjuvanted by N. meningitidis OMVs and induced antibodies which neutralized the D614G variant, which presents enhanced transmission. Importantly, most of the adverse effects related were local reactions with mild intensity [65]. Another example from clinical trials is the vaccination with Cervarix® (GlaxoSmithKline), which employs AS04 as adjuvant (composed of MPL adsorbed in alum) and decreased human papillomavirus (HPV) infections apart from types 16 and 18, probably due cross-reactive antibodies [66].
Reactogenicity concerns
An important concern about attenuated and inactivated vaccines is the reactogenicity, and the same could happen with PAMP adjuvants [52]; however, several PAMP-containing adjuvants have been tested in clinical trials, presenting acceptable adverse events, as summarized in Table 1.
Adverse events reported in clinical trials using PAMP-based adjuvants
Adjuvant | Composition | Vaccines | Adverse events |
---|---|---|---|
AS01 | MPL and saponin | SUIVs—influenza (NCT03275389) [67] | Injection site pain, fatigue, headache, myalgia |
RSVPreF3—respiratory syncytial virus (NCT03814590) [68] | Injection site pain, fatigue, headache | ||
RTS,S/AS01—Malaria (NCT00866619) [69] | Fever, irritability, drowsiness, loss of appetite | ||
M72/AS01—tuberculosis (NCT01755598) [70] | Injection-site pain and influenza-like symptoms | ||
AS04 | MPL and alum | Cervarix®—papillomavirus (approved vaccine) [71] | Injection site pain, redness and swelling, fatigue, gastrointestinal symptoms, headache |
Fendrix®—hepatitis B (approved vaccine) [72] | Injection site pain, fatigue, headache, fever | ||
CpG 1018 | CpG oligodeoxynucleotides | HEPLISAV-B®—hepatitis B (approved vaccine) [73] | Injection site pain, headache, fatigue |
Alum/OMVs | Alum and OMVs from N. meningitidis | Soberana 01—COVID-19 (RPCEC00000338) [65] | Injection site pain and redness |
SUIV: supra-seasonal universal influenza vaccines
Nonetheless, it is important to highlight that the adverse events might change according to each vaccine, when the antigen or the combination of adjuvants is considered. For example, most studies using AS01 reported mainly mild or moderate adverse events [67, 68], but a clinical trial using this adjuvant for a tuberculosis vaccines reported a case of pyrexia and two cases of immune-mediated disorders [70]. Moreover, a follow-up phase III study comprising approximately 15,000 patients found 16/5,949 and 9/4,358 cases of meningitis in children and infants, respectively, following vaccination with P. falciparum antigen and AS01 adjuvant [74], showing the relevance of conducting follow-up studies.
Conclusions
This brief review summarizes important aspects related to immunization that were relevant in recent public health emergencies. Trained immunity conferred by BCG probably reduced the burden of COVID-19 in some populations. On the other hand, the immune response to vaccinia might have cross-reacted with monkeypox. These aspects were not enough to contain any of the outbreaks; however, they show the heterologous benefits of vaccines and support the claims for better vaccine coverage worldwide. Furthermore, novel adjuvant options and combinations should be investigated, aiming similar features for subunit vaccines.
Abbreviations
AS: |
Adjuvant System |
BCG: |
Bacille Calmette-Guerin |
COVID-19: |
coronavirus disease 2019 |
CpG: |
cytosine-phosphate-guanine |
MPL: |
monophosphoryl lipid A |
OMVs: |
outer membrane vesicles |
PAMPs: |
pathogen-associated molecular patterns |
PRRs: |
pattern-recognition receptors |
SARS-CoV-2: |
severe acute respiratory syndrome coronavirus 2 |
Declarations
Author contribution
AIP: Conceptualization, Investigation, Writing—original draft. EDG: Conceptualization, Investigation, Supervision, Writing—review & editing.
Conflicts of interest
The authors have no conflicts of interest regarding the publication of this article.
Ethical approval
Not applicable.
Consent to participate
Not applicable.
Consent to publication
Not applicable.
Availability of data and materials
Not applicable.
Funding
This work was supported by
Copyright
© The Author(s) 2023.