Abstract
Aim:
Biofilms of pathogenic bacteria are phenotypically resistant to antibiotics and other antimicrobial agents, host immune systems, and adverse environmental conditions, and are responsible for most chronic infections. Antimicrobial photodynamic therapy (APDT) is based on using of photosensitizers (PS), which generate reactive oxygen species when exposed to visible or infrared light. Previously, we demonstrated the high efficacy of new polycationic bacteriochlorins as PS against biofilms of Gram-negative bacteria Pseudomonas aeruginosa in vitro. Now, we compared the toxicity of these PS to bacteria and human fibroblasts, under irradiation and in the dark. Another goal was to study the interaction of the PS with the biofilm matrix without irradiation.
Methods:
Photodynamic inactivation of eucaryotic cells was obtained with MTT test, and with plating of planktonic bacteria P. aeruginosa and biofilms after disrupting. The interaction of PS with the matrix was studied with electron microscopy, alcian blue staining, and quantitative evaluation of polysaccharides.
Results:
The absence of dark toxicity of polycationic bacteriochlorins BCl-6 and BCl-7 for human fibroblasts WI-38 at concentrations up to 200 µM and the selectivity of the studied PS to P. aeruginosa 32 bacteria, including these in biofilms, under irradiation, were found. After incubation of P. aeruginosa 32 biofilms with tetracationic BCl-6 and BCl-5 without irradiation, gross disturbances in the structure of the biofilm matrix were observed by SEM, as well as a significant reduction of Alcian blue staining and polysaccharides in the matrix.
Conclusions:
Polycationic bacteriochlorins BCl-6 and BCl-7 had no dark toxicity for human fibroblasts and were selective to P. aeruginosa 32 bacteria, including these in biofilms, under irradiation. The incubation of biofilms of Gram-negative bacteria with polycationic bacteriochlorin led to the destruction of the matrix without irradiation.
Keywords
Photosensitizer, photodynamic inactivation, polycationic bacteriochlorin, human fibroblasts, biofilms, Pseudomonas aeruginosa , polysaccharidesIntroduction
Bacterial biofilms, being a stage of a live cycle, are associations of microorganisms, sessile or floating, surrounded with exopolymer matrix, produced by them. Biofilms of pathogenic bacteria in the body of an infected host are complex structures, often formed by microorganisms of different species. They are phenotypically resistant to antibiotics and other antimicrobial agents, host immune systems and adverse environmental conditions, exhibit increased survival, and are responsible for most known chronic infections [1, 2]. This situation required an intensification of the search for alternative ways to combat biofilm infections.
Photodynamic therapy (PDT) is proposed as a promising method of inactivation of antibiotic-resistant pathogenic microorganisms in biofilms [3, 4, 5]. Antimicrobial photodynamic therapy (APDT) is based on the capabilities of modern photochemistry. The essence of the method is that photosensitizers (PS) when exposed to visible or infrared light with wavelengths corresponding to their absorption spectra, generate the formation of cytotoxic reactive oxygen species (ROS) (including singlet oxygen, superoxide ion, hydroxyl radical, H2O2, etc.) that cause oxidative destruction of the life-supporting structures of microbial cells. Unlike antibiotics, each of which specifically affects a specific target in a microbial cell—the cell wall, cytoplasmic membrane, DNA replication, transcription or translation, ROS, generated by PS, cause oxidative damage to components of both lipid and protein nature, as well as nucleic acids [6]. It is believed that such a multiple oxidative nature of damage to cellular components makes not so easy the development of resistance to APDT, and therefore, this method is considered a promising way to combat pathogens resistant to traditional medicines. By now, there are more and more publications that present data on the study of photodynamic inactivation (PDI) of microbial cells or viruses in vitro. A wide range of compounds are used as PS, including tetrapyrroles (porphyrins, chlorins, bacteriochlorins, phthalocyanines) and phenothiazine dyes [MB (methylene blue) and TBO (toluidine blue O)], activated by light of a certain wavelength [7]. Bacteria and cells that have accumulated PS (sensitized) die due to multiple damages caused by ROS: damage to the integrity and barrier function of membranes, damage to the active centers of enzymes and metabolic disorders, damage to RNA and violations of translation, finally, DNA damage that cannot be repaired [8]. It is important that PS must be selective towards bacteria and have low dark toxicity for eukaryotic cells, to limit the possible adverse effects within an irradiated field.
The APDT can be especially effective against diseases associated with the formation of biofilms by microorganisms, which are often located in various cavities of the patient’s body and can be accessed using fiber-optic technology (long-term non-healing complicated wounds of the skin and mucosa, trophic ulcers, bedsores, ulcers of diabetic feet, chronic urinary tract infections, including a catheter-associated, fouling of intubation tubes, endoprostheses) [2]. The most common pathogens of infections associated with biofilm formation and nosocomial infections are grouped with the acronym “ESKAPE” (Enterococcus faecium, Staphylococcus aureus, Klebsiella pneumonia, Acinetobacter baumannii, Pseudomonas aeruginosa, Enterobacter spp.) [9]. They are characterized by virulence and multiple resistance to antimicrobials and disinfectants. It has been shown, that APDT is more effective against Gram-positive bacteria, for example, E. faecalis, and less effective against Gram-negative ones—Klebsiella pneumoniae [10]. Nevertheless, a number of PS have proven effective against biofilms of ESKAPE bacteria, such as cationic PS of the phenothiazine class (MB and TBO) [11], cationic derivatives of chlorin [12], polycationic phthalocyanines and bacteriochlorins [13], and cationic porphyrins [14].
The problem is that bacterial biofilms that cause chronic diseases usually consist of both Gram-negative and Gram-positive species. Therefore, to create a strategy for APDT, one of the most important tasks is to study the structural features of bacterial communities that affect sensitivity to photodynamic effects, and to develop optimal approaches and means for conducting APDT of chronic diseases. In various laboratory models for bacterial infections in animals [15], oral infections treatment and dentistry [16, 17], and the treatment of chronic wounds [18], it has been demonstrated that APDT is a promising technology for combating chronic infections.
Only cationic PSs effectively interact with Gram-negative bacteria. Cationic PSs bind to the surface of Gram-negative bacteria due to electrostatic interaction and more or less successfully penetrate into the cell through porin channels (small molecules of phenothiazine PS) or across the membrane using self-promotion uptake mechanism (polycationic tetrapyrroles) [19, 20]. They are also effective against Gram-positive bacteria.
From 5% to 35% of the biofilm volume consists of microorganisms, while the remaining volume is an extracellular polymer matrix [21]. Matrix polymers are represented by polysaccharides, proteins, lipids, and nucleic acids (RNA and extracellular DNA) [22]. One of the functions of the matrix is to protect bacteria from adverse effects, including antibacterial drugs and host immune defense factors; the presence of the matrix is one of the reasons for the phenotypic resistance of microorganisms in biofilms [23]. Bacteria in biofilms are also more resistant to APDT. Apparently, the extracellular polymer matrix plays an important role in the interaction of PS molecules with bacterial biofilms.
Some of the PS molecules, associated with the biofilm, interact directly with the matrix without reaching bacterial cells. In a number of works, the authors microscopically visualized the destruction of the biofilm matrix structure after PDI with PS. After PDI with TBO of Staphylococcus epidermidis and S. aureus biofilms, changes in the structure of biofilms were revealed, expressed in a decrease in their density and a decrease in the number of adhered bacterial cells as the light dose increased [24]. Another group of researchers, after PDI of E. faecalis biofilms with MB, revealed a reduction in biofilm biomass by 88.7%, while the remaining adhesive bacteria were combined into small chains. In mixed biofilms of Gram-positive E. faecalis and Gram-negative P. aeruginosa, the both loss of biofilm biomass and changes in cellular morphology in the form of damage to the cytoplasmic membrane were observed [25]. The effect of PDI using polycationic porphyrin (Tetra-Py+-Me) on biofilms of P. aeruginosa was studied using quantitative determination of polysaccharides by the phenol-sulfuric acid method [14]. The authors concluded that the PS molecules interact with matrix polysaccharides, leading to the destruction of the biofilm after irradiation. Apparently, PDI affects both, the cells of microorganisms, leading to disruption of the cell wall, and the extracellular matrix, destroying the structure of the biofilm.
When a sensitized biofilm is excited by light from a certain wavelength, a significant part of the light is absorbed by the PS molecules associated with the matrix. In this case, ROS are released at a distance exceeding the free range of singlet oxygen (from 1 µm in water to 20 nm in the cytoplasm) from bacterial cells [26]. Therefore, a significant amount of the ROS will not reach bacterial cells, causing damage to the matrix structure and loss of biomass of bacterial biofilms.
At the same time, there are publications on the destruction of biofilms in the presence of PS even without irradiation [14, 27].
The polycationic bacteriochlorins studied in this work are promising PS for APDT, since they have a maximum absorption in the near-infrared region—the “optical window”—of biological tissues, which makes it possible to effectively irradiate deeper layers and fight local infections caused, in particular, by P. aeruginosa. When choosing PS for APDT, it should be borne in mind that the depth of foci of infectious lesions with P. aeruginosa bacteria can reach 12–15 mm [28]. Previously, we have demonstrated the high efficacy of new polycationic bacteriochlorins against planktonic bacteria and biofilms of Gram-positive S. aureus and Gram-negative P. aeruginosa in vitro. The growth of planktonic P. aeruginosa 32 bacteria was not detected at about 3 to 6 μM of PS, sensitization for 30 min, and light dose density 30 J/cm2 at λ = 760 nm. For planktonic S. aureus 15 the absence of growth was achieved at about 0.8 to 3 μM of these PS. The amount of viable bacteria P. aeruginosa 32 depended on PS concentration of all three PS and decreased for about 5log10 with 250 μM and light energy of 100 J/cm2. For S. aureus 15 almost the same result was obtained with 50 J/cm2. The efficacy of inactivation of biofilms enhanced with the time of incubation and the light dose [29]. In this paper, we compared the toxicity of these PS to bacteria and human fibroblasts, both under irradiation and in the dark. Another goal was to study the mechanism of the damaging effect of polycationic derivatives of bacteriochlorin on biofilms without irradiation and the interaction of the studied PS with the matrix using various methods: electron microscopy, alcian blue staining and quantitative evaluation of polysaccharides by the phenol-sulfuric acid method.
Materials and methods
Photosensitizers
Three new synthetic PS, derivatives of bacteriochlorin, were synthesized at the Organic Intermediates and Dyes Institute, Moscow, Russia [29].
PS differed in the number of cationic groups in the molecule, the degree of lipophilicity due to alkyl substituents (Figure 1) and have an absorption maximum in aqueous solution at λ = 760 nm [13, 30]. They are listed below amphiphilic BCl-54+ (meso-tetrakis(1-heptyl-3-pyridyl)bacteriochlorin tetrabromide) in 4% Kolliphor ELP (BASF, Germany) dispersion in distilled water, hydrophylic BCl-64+ (meso-tetrakis[1-(2'-bromoethyl)-3-pyridyl]bacteriochlorin tetrabromide) and hydrophylic BCl-78+ (meso-tetrakis[1-(2'-pyridinioethyl)-3-pyridyl]bacteriochlorin octabromide). Stock solutions (1 mM) in distilled water were prepared immediately before experiments.
Investigation of cytotoxicity of photosensitizers for eukaryotic cells (human fibroblasts WI-38)
The immortalized human fibroblast cell line WI-38 was used in the work. Cells were seeded into 96-well plates (Thermo Scientific, USA) in the amount of 8,000 cells per well in 100 µL of DMEM medium (Lonza, Switzerland) containing 2 mM glutamine, a solution of an antimycotic-antibiotic (100 EU penicillin, 100 µg streptomycin and 250 ng amphotericin in 1 mL) with the addition of 10% of calf fetal serum (HyClone, USA) and cultured at 37°C and 5% CO2 in a laminar system [BAVp-01-Laminar-S-1.5(220.150), Lamsistems, Russia]. After 24 h, PSs were added to the test concentrations, each point in triplicate. The cells were incubated with PS solutions for 30 min or 1 h, after which they were washed twice with DMEM medium. The irradiation was carried out with a LED (light emitting diode) light source “SPD-M-760 LED” (BIOSPEC, Russia) with a power density of 25 mW/cm2. The time of irradiation was 8 min and the surface dose of light energy was 12 J/cm2. The control plate was not irradiated. The plates were placed for 24 h in an incubator at 37°C and 5% CO2.
The effect of PS on cell survival was determined by analyzing the metabolic activity of cells using the MTT test. A solution of tetrazolium dye was introduced into each well of the plate (0.5 mg/mL), which, under the action of mitochondrial dehydrogenases of viable cells, turns into a violet-colored formazane insoluble in water, and was incubated for 2 h at 37°C with 5% CO2 in a laminar system (Lamsistems, Russia). After 2 h, the contents were taken from each well and a dimethyl sulfoxide (DMSO) solution was introduced to extract formazane crystals. The optical density of formazane in DMSO was measured on a spectrophotometer (Thermo Scientific, USA) at a wavelength of 540 nm.
Photodynamic inactivation of planktonic bacteria
Clinical isolates of Gram-negative bacteria P. aeruginosa 32 and Gram-positive bacteria S. aureus 15 from the collection of the N.F. Gamaleya Research Center of Epidemiology and Microbiology were employed. LB-broth [(Luria-Bertani broth) Amresco, USA] was used for the cultivation of bacteria. To prepare a solid nutrient medium, 1.5% Agar Bacteriological (Helicon, Russia) was added to the LB-broth.
The bacterial culture was grown in LB-broth at 37°C for 18 h, diluted 20 times with LB-broth, and grown with intensive stirring for 1.5 h to an exponential growth phase. The culture was centrifuged at 4,330 g for 10 min. The precipitate was suspended in sterile saline to a turbidity of the McFarland standard ~1 × 108 CFU/mL.
Solutions (or dispersions) of PS in the tested concentration were added to the suspension of bacteria (~1 × 108), and saline at the same volume was added to the suspension of bacteria as a control. The samples were inserted into 96-well plates of 100 µL per well (4 wells per point) and incubated at 37°C for 30 min in the dark. After incubation, one plate was irradiated with a LED light source “SPD-M-760 LED” (BIOSPEC, Russia), while the control plate stayed in the dark. The dark control was maintained at the same conditions as the samples. The dose of light energy on the surface was D = 12 J/cm2. Next, six tenfold dilutions from every well were prepared in saline and 20 µL were inoculated on Petri dishes with a solid nutrient medium. Viable colonies were counted 24 h after incubation at 37°C.
Photodynamic inactivation of bacteria in biofilms
Bacterial biofilms were grown in a 96-well plate for 24 h at 37°C in 100 µL of LB per well. The liquid was aspirated, 100 µL aliquots of PS solutions were added to the tested concentrations, and saline was added to the control wells at the same volume. The samples were incubated at 37°C in the dark for 30 min, then the solutions were aspirated, 100 µL aliquots of distilled water were put in every well and the plate was irradiated with an LED light source “SPD-M-760 LED” (BIOSPEC, Russia) at a dose of 12 J/cm2. The control plate was not irradiated. To destroy the biofilm, 11 µL of BFR solution (composition of enzymes and surfactants) (BFR Products, Russia) was added to the wells at a final concentration of 0.1%. After 10 min, they were intensively pipetted, and a series of tenfold dilutions were inoculated in 20 µL on a Petri dish with LB-agar for further colony counting after 24 h. CFU/mL was calculated by taking into account the volume of seeding on the dish and dilution.
Electron microscopic analysis of biofilm structure
P. aeruginosa 32 biofilms were grown on silicon substrates in a Petri dish with LB-broth for 48 h at 37°C. The culture medium was removed, the substrates were washed with distilled water, and aqueous solutions (or dispersions) of PS were added at a concentration of 250 µM; the control sample was incubated in distilled water, as usual for microscopy. After 1 h of incubation in a thermostat, the solutions were removed, the substrates were washed with distilled water, and the preparations were fixed with vapors of a 10% formaldehyde solution for 24 h. All operations were performed in a darkened room. Next, the samples were sputtered with gold (layer thickness 5 nm) in a SPI-MODULE Sputter Coater setup (SPI Supplies, USA). Electron microscopic analysis of biofilms was carried out using a Quanta 200 3D dual-beam scanning ion electron microscope (FEI Company, USA) in high vacuum mode at an accelerating voltage of 5–7.5 kV.
Visual assessment of biofilms with alcian blue staining
Visualization of changes in the biofilm matrix after treatment with a solution of the PS BCl-6 was carried out using alcian blue staining, which stains acidic polysaccharides. Bacterial biofilms were grown at 37°C for 24 h on glasses placed in the wells of a 24-well plate with 500 µL of bacterial suspension 1 × 106 CFU/mL in LB-broth. Then the supernatant was removed, and 500 µL of PS solution at a concentration of 250 μM was added to the wells. As a control, distilled water was added in the same volume. After incubation for 60 min at 37°C, the solutions were removed, and 500 µL of a 0.1% alcian blue aqueous solution was added to each well and incubated for 1 h. Then the dye was removed, the biofilms were washed three times with distilled water, and the slides from the wells were transferred to Petri dishes and dried.
Quantitative evaluation of biofilm matrix polysaccharides with alcian blue staining
Bacterial biofilms were grown in a 24-well flat-bottom plate for 24 h at 37°C, in 500 µL of LB-broth per well. The supernatant was aspirated and 500 µL of 250 μM solution of PS BCl-6 was added. In control wells, distilled water was added in the same volume. After incubation for 60 min at 37°C, the solutions were aspirated, 500 µL of a 0.1% alcian blue solution was added and incubated for 1 h. After staining, the biofilms were washed three times with distilled water and 500 µL of 33% acetic acid was added to the wells to extract the dye bound to the biofilms. After 1 h, the intensity of the extracted dye was measured using a photometer Multiskan FC (Thermo Scientific, USA) in a 96-well plate, 100 µL per well at a wavelength of 620 nm.
Evaluation of polysaccharides in P. aeruginosa biofilms using the phenol-sulfuric acid method
We used the previously described technique [14], with modifications.
Biofilms of P. aeruginosa 32 were grown for 72 h in Petri dishes with diamer10 cm in a volume of 20 mL at 37°C. The supernatant was aspirated and 20 mL of 250 µM aqueous solution of BCl-6 was added. In a control dish saline was added at the same volume. After incubation for 60 min at 37°C, the solutions were removed, 20 mL of 1 M NaCl solution was added to destroy biofilms and incubated for 30 min. The samples were transferred into a 50 mL tube and TCA (trichloroacetic acid) was added to a final concentration of 17% and centrifuged for 20 min at 10,000 g, 4°C. Next, the supernatant was collected and 4 volumes of 96% ethyl alcohol were added to it. The samples were centrifuged for 20 min at 10,000 g, 4°C to sediment the polysaccharides. The supernatant was well removed with an aspirator and 200 µL of distilled water was added. To determine the amount of polysaccharides, the phenol-sulfuric acid method with glucose calibration was used: 50 µL of test solutions of polysaccharides or 50 µL of a glucose solution at a known concentration, 150 µL of concentrated sulfuric acid and 30 µL of 4% phenol were added to the wells of a 96-well plate and incubated for 30 min [31]. The absorption was measured on a photometer Multiskan FC (Thermo Scientific, USA) at a wavelength of 450 nm.
Statistical processing of results
Statistical data processing and construction of graphs and diagrams were carried out using the GraphPad Prism 9 program. The statistical significance of differences between groups with quantitative values was assessed using the ANOVA criterion. Differences were considered statistically significant at P < 0.05 and P < 0.01.
Results
Comparison of toxicity of photosensitizers for eukaryotic cells and bacteria
Cationic bacteriochlorins BCl-5, BCl-6, and BCl-7 were tested on human fibroblasts WI-38 at different concentrations and sensitization times—30 min and 60 min, the dose of light energy was 12 J/cm2. Cytotoxicity was determined using the MTT test and the viability of the cells in the dark and after irradiation is presented in Figure 2. The values for the cells without PS were taken as 100%. IC50 (the concentration of a substance that leads to a decrease in the number of viable cells to 50%) calculated from the graphs for all three PS without and after irradiation are summarized in Table 1.
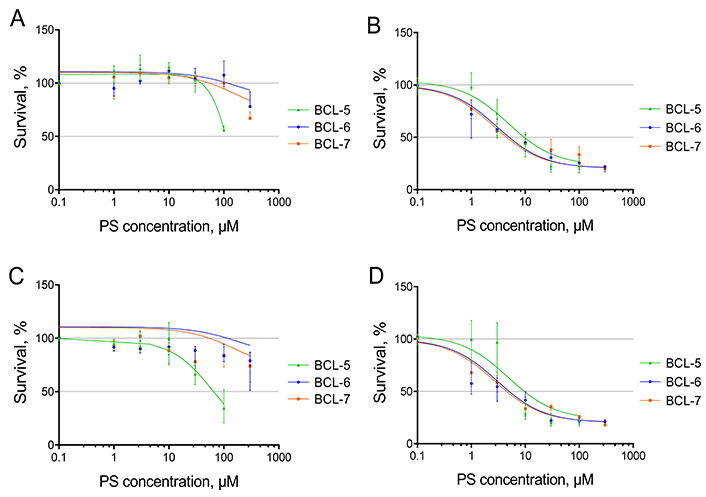
The dependence of the survival of human fibroblasts WI-38 on the concentration of PS (photosensitizers). A and C—0 J/cm2, B and D—12 J/cm2. Incubation time with PS: A and B—30 min, C and D—60 min. The values for the cells without PS were taken as 100%. For details on the IC50 curve plotting method, please refer to the Materials and methods section
Calculated IC50 (µM) of cationic bacteriochlorins for human fibroblasts WI-38 without irradiation and with irradiation of 12 J/cm2
Photosensitizer | IC50 (µM) | |||
---|---|---|---|---|
Sensitization time | 30 min | 60 min | ||
Light dose | 0 | 12 J/cm2 | 0 | 12 J/cm2 |
BCl-5 | n | 9.55 | 65.0 | 11.65 |
BCl-6 | n | 4.95 | n | 3.40 |
BCl-7 | n | 4.50 | n | 4.20 |
n: not achieved
The dark cytotoxicity of the BCl-6 and BCl-7 during sensitization for 30 and 60 min for human fibroblasts WI-38 was insignificant (Figure 2A, C and Table 1). In the range of tested concentrations (up to 200 µМ), IC50 was not achieved without irradiation. However, BCl-5 had a more pronounced cytotoxic effect compared to other PS during prolonged incubation (60 min). Under irradiation, the cytotoxicity of all the studied PS increased (Figure 2B, D and Table 1).
We conducted experiments on PDI under the same conditions for planktonic bacteria P. aeruginosa and they showed that at concentrations of PS close to IC50 for human fibroblasts (BCl-5: 10 µM, BCl-6 and BCl-7: 5 µM), the growth of only single colonies in undiluted samples was observed after irradiation with 12 J/cm2 (see Materials and methods for details). There was no dark toxicity of the studied PS for bacteria in these concentrations. The initial titer of bacteria was about 1 × 108 CFU/mL, and the detection limit was 50 CFU/mL, i.e. the death of planktonic bacteria at concentrations of PS, close to IC50, was more than 6log10.
In biofilms of P. aeruginosa bacterial death under the same conditions was 10- to 100-fold, as can be seen from Figure 3. It has been shown previously, that in the dark, BCl-5, BCl-6, and BCl-7 even at higher concentrations did not cause the death of bacteria in P. aeruginosa biofilms, as well as the light alone [29]. These results indicate the absence of dark toxicity of BCl-6 and BCl-7 for human fibroblasts at concentrations up to 200 µM and the selectivity of the studied polycationic bacteriochlorins towards bacteria under irradiation.
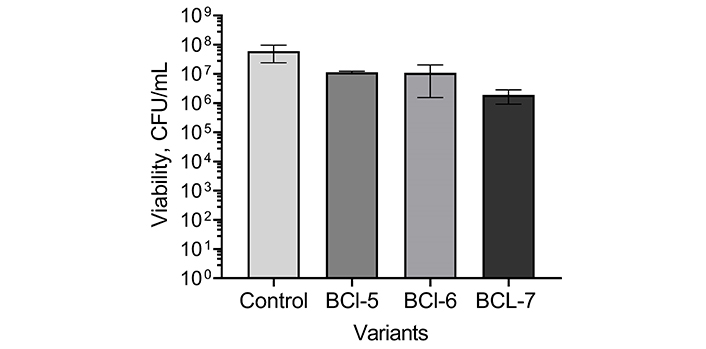
Photoinactivation of bacteria in Pseudomonas aeruginosa biofilms using polycationic bacteriochlorins. BCl-5: 10 µM; BCl-6: 5 µM; BCl-7: 5 µM; Control: no PS (photosensitizers). Biofilms were treated for 30 min and irradiated with 12 J/cm2
Electron microscopy of biofilms after exposure to photosensitizers
The interaction of polycationic PS with the matrix of P. aeruginosa 32 biofilms was studied using electron microscopy with a Quanta 200 3D dual-beam scanning ion electron microscope (FEI Company, USA). Unlike a conventional scanning microscope, the Quanta 200 3D does not require sample dehydration and thus preserves the structure of bacterial biofilms. Samples of intact biofilm, biofilm after incubation for 60 min with water as a control, and biofilm after sensitization with BCl-5 and BCl-6 for 60 min without irradiation were studied (Figure 4). From our experience, the solutions of our bacteriochlorins in saline and distilled water do not differ in impact on colony forming ability of planktonic P. aeruginosa. We used distilled water in experiments on electron-ion microscopy for analysis of the matrix structure and consequent experiments, not connected to assessment of viability.
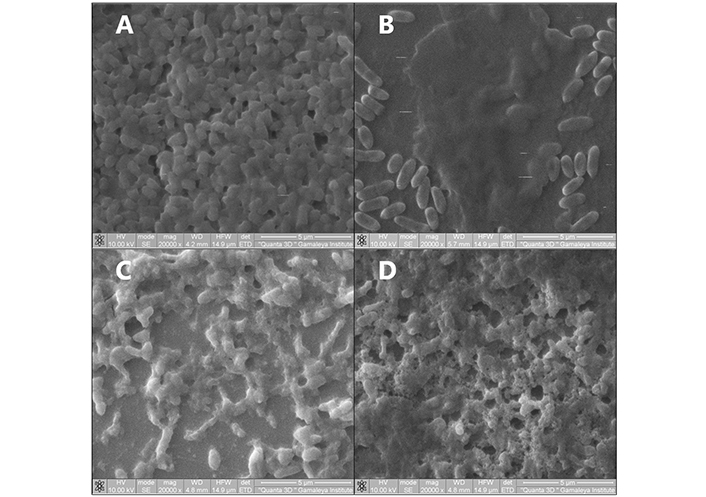
Scanning electron microscopy of Pseudomonas aeruginosa 32 biofilms. (A) intact biofilm. Biofilms were exposed to (B) distilled water, (C) BCl-5 (250 µM), (D) BCl-6 (250 µM) without irradiation for 60 min. Magnification: 20,000×; scale bar: 5 µm
When examining an intact P. aeruginosa 32 biofilm using SEM, bacteria are visible protruding through the smooth, uniform surface of the matrix (Figure 4A). Incubation with water as a control led to a partial loss of the biofilm matrix, but where it remained, no structural change in the matrix was observed: The polymer matrix retained its smooth structure (Figure 4B). At the same time, when samples were incubated with solutions of tetracationic BCl-6 and BCl-5 without irradiation, gross disturbances in the structure of the biofilm matrix were observed: the matrix was absent in places, clots, and perforations formed in the structure of the biofilms (Figure 4C and D).
Study of the effect of photosensitizers on biofilm matrix polysaccharides using alcian blue staining
We tried to answer the question of whether matrices were destroyed when one of the PSs (BCl-6) was exposed to a biofilm without irradiation.
The effect of BCl-6 on the biofilm matrix was studied using alcian blue staining, which specifically stains polysaccharides bright blue. For comparison, experiments with Gram-positive S. aureus 15 were performed. The effect of the BCl-6 on the matrix was visualized using P. aeruginosa 32 and S. aureus 15 biofilms formed on glasses, followed by staining with alcian blue (Figure 5).
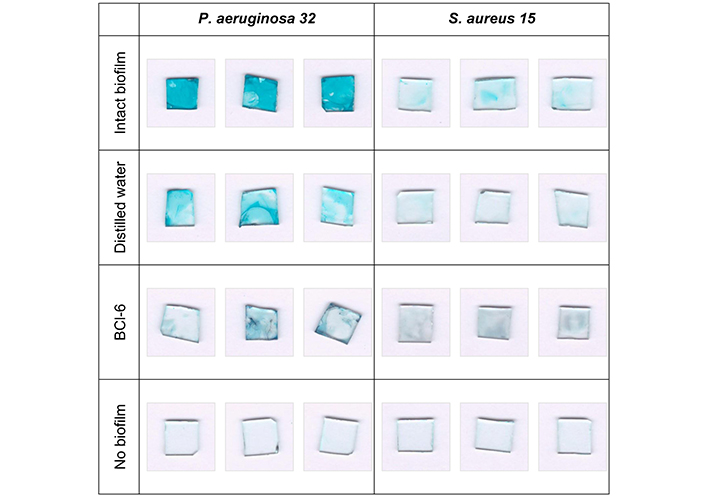
Photographs of Pseudomonas aeruginosa 32 and Staphylococcus aureus 15 biofilms stained with alcian blue. Biofilms on the glasses were incubated with distilled water or aqueous solution of BCl-6 for 60 min, each option in triplicate, without irradiation
Glasses with intact biofilms of P. aeruginosa 32 were brightly colored, which indicates a large amount of polysaccharides. Incubation with distilled water led to a decrease in the color intensity of the glasses compared to the intact biofilm. However, after exposure of P. aeruginosa 32 biofilms to BCl-6, the color of the glasses practically disappeared, which may indicate the destruction of matrix polysaccharides in the presence of a PS without irradiation, because all procedures were performed in the well-shadowed room. The destroyed polysaccharides could go into solution and be removed by an aspirator when washing the samples. The color of the intact S. aureus 15 biofilm looked much less bright and the differences in the color intensity of the intact biofilm after incubation with water and incubation with BCl-6 were not significant. This is likely due to the different matrix composition of Gram-positive and Gram-negative bacteria; it is known that proteins predominate in the matrix of Gram-positive S. aureus, and polysaccharides are contained in significantly smaller quantities [32].
Similar results were obtained in the following experiments, where the amount of alcian blue bound to biofilms was assessed. The color intensity of the bound dye after extraction with acetic acid, expressed in extinction units, for biofilms treated with BCl-6, distilled water, and intact ones, is shown in Figure 6.
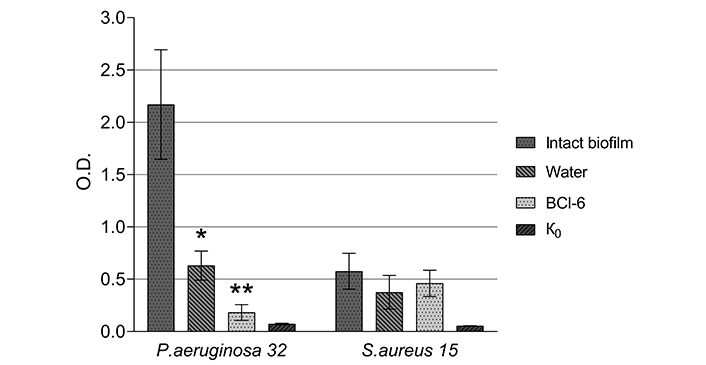
Staining of Pseudomonas aeruginosa 32 and Staphylococcus aureus 15 biofilms with Alcian blue after incubation with BCl-6 (250 µM) and water for 60 min. K0: control (wells after incubation with LB-broth without bacteria). Values are means, errors are standard deviation (SD). *: Differences in optical density (at 620 nm) between intact biofilm and those incubated with water for 60 min are significant: P < 0.05; **: the differences in optical density (at 620 nm) between the biofilm incubated for 60 min with water and with BCl-6 are significant: P < 0.05
Incubation of P. aeruginosa 32 biofilms with BCl-6 without irradiation significantly reduced the color of extracts. Incubation with water also leads to a decrease in the intensity of staining of samples compared to the intact biofilm, but to a lesser extent.
The color intensity of the intact S. aureus 15 biofilm was significantly less compared to the P. aeruginosa 32 biofilm and changes in the color intensity of S. aureus 15 biofilms after incubation with distilled water and a solution of the cationic PS BCl-6 insignificant. This may be due to the low content of polysaccharides in the matrix of biofilms of Gram-positive bacteria.
The effect of BCl-6 on polysaccharides of P. aeruginosa biofilm matrix studied with the phenol-sulfuric acid method
The phenol-sulfuric acid method is based on photometric measurement of the absorption of the products of the interaction of phenol with sugars, which are formed as a result of the destruction of polysaccharide molecules in concentrated sulfuric acid [31]. The amounts of polysaccharides are expressed in glucose equivalents and calculated using a calibration graph of the dependence of the optical density of the solution on the glucose concentration.
The experiment design was as follows: A 72-hour biofilm of P. aeruginosa 32 was incubated with water and with BCl-6 at a concentration of 250 µM for 60 min without irradiation. An intact biofilm was a control. Next, polysaccharides were isolated as described in Materials and methods. Their quantity was determined using the phenol-sulfuric acid method.
The results of 3 experiments are presented in Figure 7, which shows a significant decrease in the amount of polysaccharides in the biofilm matrix after exposure to BCl-6 compared to an intact biofilm. This may indicate that the matrix polysaccharides were destroyed when exposed to PS and passed into solution from the biofilm. Incubation of biofilms with water also led to a decrease in the amount of biofilm matrix polysaccharides, but to a lesser extent. Summarizing the data obtained, we can conclude that incubation of biofilms of Gram-negative bacteria with polycationic bacteriochlorin led to the destruction of the matrix without irradiation.
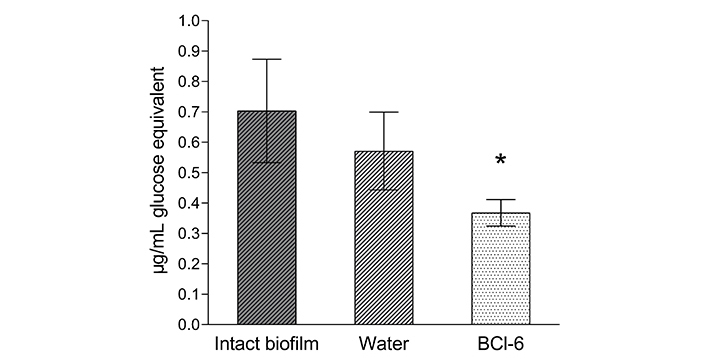
Polysaccharides content of the biofilm matrix of Pseudomonas aeruginosa 32 as glucose equivalent. Biofilms were incubated with 250 µM BCl-6 for 60 min in the dark, distilled water was a control. *: The difference with the control is significant P < 0.05
Discussion
As a result of this work, it was shown that at the same photodynamic parameters, polycationic bacteriochlorins BCl-5, BCl-6, and BCl-7 caused a decrease in the number of viable human fibroblast cells by 50% and CFU/mL of planktonic bacteria P. aeruginosa 32 by 99.9999%. At the same time, the number of viable bacteria in P. aeruginosa 32 biofilms decreased by about 10- to 100-fold. Thus, the selectivity of the PS in the study towards bacteria was shown. We observed a decrease in the viability of fibroblasts after irradiation with LED at 760 nm. So, we have chosen a low dose, 12 J/cm2, where the decrease in the viability of WI-38 cells was 15%. However, a significant decrease in the number of viable bacteria in biofilms (3log10 or more) requires a higher concentration of these PS—250 µM at a dose of light energy of 25 J/cm2, as was shown previously [29].
A question arises: will PDT with polycationic bacteriochlorins be sufficiently effective against biofilms in vivo?
It is known that sub-lethal doses of APDT cause a decrease in the ability to form biofilms and the enzymatic activity of secreted proteases, as well as a decrease in the metabolic activity of bacteria [33]. Sub-lethal doses of APDT lead to a decrease in the activity of Quorum Sensing systems, and consequently to the suppression of the synthesis of exopolysaccharides and the formation of biofilms [34]. The expression of virulence genes is also reduced in bacteria that survived sub-lethal PDI with TBO, while the susceptibility to several tested antibiotics is enhanced [35].
The immunomodulatory effect of PDT is also known. APDT of local microbial infections achieves a therapeutic effect not only by acting directly on bacteria, but also through the bactericidal effect of host neutrophils, namely, the attraction of neutrophils, which is stimulated by PDT [36]. Experiments on animal models with infected wounds will help answer this question. Moreover, higher concentrations of PS (up to 200 µM) and doses of light can also be used in local therapy, since the main criterion is the absence of dark toxicity of PS for eukaryotic host cells, and the studied polycationic bacteriochlorins correspond to this condition.
As for the interaction of PS with biofilm, matrix exopolymers can serve as a “trap” for PS molecules. For example, the binding of polycationic bacteriochlorins to the matrix of P. aeruginosa 32 biofilms has been shown in the work of Meerovich et al. [37]. In several works it was demonstrated by SEM, CLSM, crystal violet staining, or evaluation of polysaccharides, that biofilm was disrupted after photodynamic treatment [10, 14, 24, 25]. In our experiments, we observed the deformation and partial destruction of the matrix of P. aeruginosa 32 biofilms in the dark under the action of polycationic bacteriochlorin BCl-6 by various methods: SEM, measurement of the optical density of extracts during staining with Alcian blue, specific for polysaccharides, as well as the evaluation of polysaccharides in the matrix by phenol-sulfuric acid method. These data indicate the interaction of the studied polycationic bacteriochlorins not only with bacteria, but also with the biofilm matrix, which led to a disturbance of its structure even without irradiation.
To our knowledge, there are two publications, where the decrease of biofilm matrix after incubation with another PS in the dark was observed. Cationic PS SAPYR (7-perinaphtenone) was shown not only effectively eliminate E. faecalis bacteria in biofilm after irradiation, but also lead to a decrease in the mass of biofilm without irradiation, which was registered by staining with crystal violet [27]. The authors attributed this to the destruction (detachment) of biofilms during incubation with PS. The other group observed the decrease of polysaccharides amount in P. aeruginosa biofilm after incubation with cationic porphyrin (Tetra-Py+-Me) in the dark by phenol-sulfuric acid method [14]. The authors hypothesized the disruption of the matrix because of a decrease in cohesiveness.
The mechanism of interaction of porphyrin with biofilm matrix was discussed by Patel et al. [38]. The authors have discovered that ZnPor (cationic metalloporphyrin with zinc as the central metal ion) can directly kill bacteria P. aeruginosa without photoactivation, as well as destroy the biofilm matrix in both the presence and absence of light. The disruption of the biofilm matrix was registered by the decrease in extracellular DNA (eDNA) content. The same features another porphyrin-5,10,15,20-tetrakis(1-methylpyridino)-21H,23H-porphine, tetra-p-tosylate salt (TMP) possessed, being a PS [39]. Based on their own and previous investigations, the authors support the interaction of eDNA in the biofilm matrix and the cationic porphyrin in the dark. Indeed, in the rather old work, it was demonstrated that cationic porphyrins (such as H2T4), amphiphilic, water-soluble molecules have a natural affinity for the DNA [40]. It is highly likely that it would intercalate into the DNA or aggregate with [41].
The interaction of polycationic tetrapyrroles with exopolysaccharides also cannot be excluded. The matrix structure is maintained due to the electrostatic and hydrogen interactions of exopolymers and in the presence of polycationic molecules, like cationic tetrapyrroles, the gel structure can be destroyed. Sheinin et al. [42] studied the interaction of alginate with Cholosens (a mixture of octa- and hepta-cationic Zn phthalocyanines). Cholosens interaction with sodium alginate led to a conformational shift of alginate and disturbance of the gel structure. It can be assumed that polycationic bacteriochlorins can interact with DNA and polysaccharides of the matrix, which leads to its destabilization and detachment.
Thus, the mechanism of damage to biofilms during APDT can be represented as follows and concerns two stages: The first-polycationic PS penetrates the biofilm and binds partially to bacteria, and partially to the matrix, while the matrix is disturbed and partially destroyed as a result of electrostatic and non-covalent interactions; the second-the partial destruction of the matrix facilitates the access of PS and oxygen molecules to bacteria, which enhances their effective inactivation during irradiation as well as the further damage of the matrix.
In conclusion, the results of this work indicate the absence of dark toxicity of polycationic bacteriochlorins BCl-6 and BCl-7 for human fibroblasts WI-38 at concentrations up to 200 µM and the selectivity of the studied polycationic bacteriochlorins as PS against P. aeruginosa 32 bacteria, including these in biofilms, under irradiation.
The observed disturbance and partial destruction of the biofilm matrix of P. aeruginosa 32 under the action of polycationic bacteriochlorin BCl-6 in the dark indicate the interaction of the studied polycationic bacteriochlorin not only with bacteria, but also with the biofilm matrix, which leads to a damage of its structure even without irradiation.
Abbreviations
APDT: | antimicrobial photodynamic therapy |
IC50: | the concentration of a substance that leads to a decrease in the number of viable cells to 50% |
LB-broth: | Luria-Bertani broth |
LED: | light emitting diode |
PS: | photosensitizer |
PDI: | photodynamic inactivation |
ROS: | reactive oxygen species |
TBO: | toluidine blue O |
Declarations
Author contributions
IT: Conceptualization, Investigation, Writing—original draft, Writing—review & editing. YZ and ET: Investigation, Methodology, Formal analysis. EM: Investigation, Writing—review & editing. AB and NS: Investigation, Formal analysis. VZ and MB: Methodology, Writing—review & editing, Validation. YR: Writing—review & editing. All authors read and approved the submitted version.
Conflicts of interest
The authors declare that they have no conflicts of interest.
Ethical approval
Not applicable.
Consent to participate
Not applicable.
Consent to publication
Not applicable.
Availability of data and materials
The raw data supporting the conclusions of this manuscript will be made available by the authors, without undue reservation, to any qualified researcher.
Funding
Not applicable.
Copyright
© The Author(s) 2024.