Keywords
Ionizing radiation, cardiovascular disease, medical exposure, telomere, deoxyribonucleic acid damage response, senescence-associated secretory phenotypeLow-dose ionizing radiation and cardiovascular disease
Cardiovascular diseases (CVDs), especially acute and chronic coronary artery disease, remain the leading cause of morbidity and mortality [1].
In 2019, it was estimated that there were 12.7 million new cases of CVDs in European countries, and every year, more than 1.7 million people die from CVDs, representing around 37% of all deaths [1].
In recent years, the risk of CVDs has been associated with exposure to environmental risk factors, such as ambient and indoor air pollution, noise, second-hand smoke and chemicals [2–4].
According to a recent report published by the European Environment Agency [4], environmental factors have been estimated to cause over 18% of CVDs-related deaths in Europe [4], highlighting the importance of reducing exposure risks in order to significantly reduce deaths from the burden of CVD.
Ionizing radiation (IR) is the most extensively studied environmental risk factor [International Agency for Research on Cancer (IARC): group 1] for cancer, but exposure to IR might also increase the risk of adverse non-cancer outcomes, particularly with CVDs such as ischemic heart disease and stroke [5–7].
Currently, it is well-established that high doses of IR, such as those used for radiotherapy can produce direct damage to the heart and arteries, leading to an increased risk of CVD [5–7]. However, evidence is emerging that IR exposure may also increase the long-term risk of cardiovascular effects even at low levels of doses less than 0.1 Gray (Gy) or 0.1 sievert (Sv; 1 Sv is equivalent to Gy in medical imaging because the radiation weighting factor for X-rays and gamma rays is 1.0), which are characteristic of doses from medical diagnostic exposures [8, 9].
Indeed, the association between CVDs and low-dose exposure has been recently confirmed by a large comprehensive meta-analysis performed on 93 cohort studies, including individuals who had been exposed to radiation in various settings (mostly radiotherapy and occupational exposures, but also diagnostic radiology and environmental exposures). The authors found a significantly increased excess risk per unit dose for ischaemic heart disease, cerebrovascular disease and all CVD [10].
For most types of CVDs, the incremental excess risk per radiation dose was higher at lower doses (< 0.1 Gy), and lower dose rates (protracted exposures over hours to years). The authors observed an increased excess lifetime risk of 2.3 to 3.9 cardiovascular deaths per 100 persons exposed to 1 Gy of radiation on a population basis. This is in addition to the high natural incidence rate. Since cardiovascular mortality accounts for 37% of all deaths in European countries, a person exposed to 1 Gy of radiation will have a relatively higher of 39% to 41%, risk of dying from CVD. Therefore, the estimates of population-based excess mortality risks for circulatory disease may be similar to those for radiation-induced cancer (the lifetime risk of fatal cancer to 1 Gy exposure is ~5% based on the linear no-threshold model).
These findings suggest that the existing radiation-induced health risks might have been significantly underestimated, implying that radiation protection standards at low doses should be reconsidered [9, 10].
Clinical implications
The possible excess risk of CVDs following exposure to low doses is of great clinical concern for patients who undergo recurrent diagnostic and therapeutic procedures with high doses of radiation exposure, such as cardiac fluoroscopic interventions, cardiac computed scan and myocardial perfusion imaging with radionuclides [11, 12]. Indeed, cardiac imaging procedures employing IR have an effective dose in the range of 2–30 millisieverts (mSv) depending on the procedure [11]. Many patients can be exposed to high cumulative radiation doses since they often undergo recurrent imaging procedures, which can result in doses higher than Gy or Sv.
Young adults and children may be most at risk for developing radiation-induced health effects because their tissues are more susceptible to radiation damage and because they have a longer lifetime risk than older patients [13].
For instance, an assessment of organ doses in a large cohort of children and young adults treated with fluoroscopically guided cardiac catheterization showed that the organs receiving the highest doses per procedure were the lungs (20.5 mSv), heart (19.7 mSv), and breasts (14.1 mSv). Of particular concern, around 13% of patients received cumulative doses to the heart and lungs of greater than 100 mSv [14].
Thus, the benefits of cardiovascular imaging with IR must be weighed against the potential harm from low-dose IR and individualized after careful clinical evaluation and assessment. Patients age and the probability of other serious diseases must be taken into consideration.
In addition, cumulative occupational exposure to low doses of radiation may increase the risk of CVDs in healthcare workers [15–17].
Currently, cardiologists who perform invasive cardiovascular procedures are among the most highly exposed physicians. By using current equipment and protection practices, the operator dose per procedure correlates somewhat with the patient dose with an average of 8 μSv to 10 μSv, receiving a cumulative lifetime dose of around 300 mSv after a 30-year active professional career [15].
Therefore, it is important to optimize procedures and enhance adherence to new protocols, which significantly reduces radiation exposure to both patient and physician by using the lowest radiation dose required while maintaining diagnostic image quality [11].
Currently, the cumulative radiation dose from hybrid imaging modalities, such as combined positron emission tomography (PET)-computed tomography (CT) scanning is also a topic of great discussion [18].
Indeed, combined PET-CT imaging has recently become a common functional imaging modality, providing simultaneously functional with anatomical information in a single study.
However, radiation exposure from the PET/CT examinations increases patient exposure compared with individual CT or PET examinations as the effective dose is a combination of the dose from PET and the dose from CT.
The radiation exposure from PET/CT imaging is considerable, especially for those patients undergoing regular follow-up with repeated imaging receiving a cumulative effective dose of ≥ 100 mSv [18]. Once again, any effort should be made to minimize radiation exposure by justifying and optimizing PET/CT examinations, and when possible utilizing PET/magnetic resonance imaging (MRI) as a good alternative for providing anatomical information without CT radiation exposure.
Undoubtedly, the knowledge about the potential health risk from low IR is one of the most effective strategies for developing a radiation safety culture that minimizes radiation exposure both to patients and doctors.
Persistent deoxyribonucleic acid damage response and senescence-associated secretory phenotype as the main triggers of radiation-induced cardiovascular effects
The biological evidence on the cardiovascular effects following low-dose IR is still inconclusive and the underlying molecular mechanisms are relatively poorly understood.
Several biological processes by which IR could affect the cardiovascular system have been proposed including, endothelial dysfunction, inflammation, oxidative stress, alterations in coagulation and platelet activity, vascular senescence, apoptosis and autophagy [6, 7].
It is well established that deoxyribonucleic acid (DNA) damage is the most important cellular target damaged by exposure to IR. Radiation-induced double-strand breaks (DSBs) are the most severe lesions, which are induced directly, but also indirectly through the formation of reactive oxygen species (ROS) [13, 19].
In response to DNA DSB, complex signaling is initiated, known as the deoxyribonucleic acid damage response (DDR), which activates cell cycle checkpoints that slow down or stop cell cycle progression while coordinating DNA repair events [19]. When the cells fail to repair their DNA, they can enter into an irreversible state of cell-cycle arrest, a cell condition associated with multiple pathophysiological cellular and molecular changes [20], also known as senescence-associated secretory phenotype (SASP).
The SASP involves the secretion of multiple pro-inflammatory cytokines and chemokines, various growth factors and proteases as well as an increased production of ROS, which can induce and amplify senescence in surrounding cells in an autocrine manner [20]. In particular, telomeric DNA regions are particularly sensitive to oxidative stress compared to the rest of the genome due to their high content of guanine triplets, which are highly susceptible to oxidative modifications and less efficiently repaired when subjected to IR-induced damage [21, 22].
The oxidative DNA damage that preferentially persists at telomeres leads to permanent DDR activation over time, highlighting their specific relevance as targets of genotoxic stress in vitro and in vivo [21, 22].
In recent years, a wealth of studies from cell cultures, animal models and human tissues provided strong evidence that oxidative DNA damage and telomere dysfunction are major players causing vascular aging and the development of atherosclerosis [23, 24].
Of particular importance, current studies consistently showed that telomere dysfunction is involved in the development of age-associated CVDs, especially coronary atherosclerosis, myocardial infarction, ischemic heart disease, stroke, and heart failure [25, 26]. Critically shortened telomeres induce senescence in both vascular endothelial cells (ECs) and vascular smooth muscle cells (VSMCs) in human and mouse models, leading to an increased pro-inflammatory response and endothelial activation [26].
Of relevance, long-term exposure to scatter radiation in the cardiac catheterization laboratory has been associated with an increased subclinical carotid intima-media thickness and telomere length shortening, suggestive of accelerated vascular aging and early atherosclerosis [27].
Thus, it is plausible to propose a unifying model of radiation-accelerated vascular injury, according to which IR exposure exacerbates vascular aging by induction of a premature senescence leading to a functional decline of cells and tissue [28].
In this model, severe irreparable DNA DSBs and telomere shortening, induce SASP which promotes chronic inflammation and detrimental effects on extracellular structures and neighboring cells, leading to progressive vascular dysfunction over time (Figure 1).
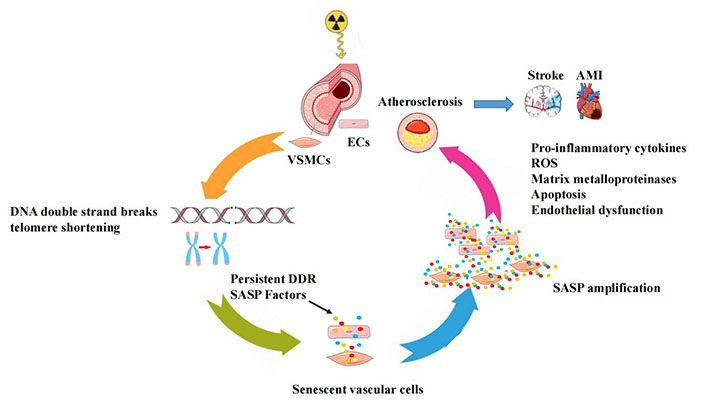
A conceptual model for the radiation-induced vascular effects. In vascular cells, IR may initially induce irreparable DNA damage and telomere erosion, which activate permanent DDR-inducing vascular cellular senescence with increased SASP signals, that promote an increased pro-inflammatory response in the vessel wall and accelerate the development of atherosclerosis leading to cardiovascular complications. AMI: acute myocardial infarction
Research perspectives
In summary, the important work presented by Little et al. [10] showed a significant association between low-dose radiation exposure and increased lifetime risk of heart disease, suggesting the need for increased vigilance around medical and occupational exposure to IR.
However, further research is warranted to determine the possible modifying effects of traditional risk factors on radiation risk, including age, gender, smoking, obesity, diabetes, high blood pressure and high cholesterol [10].
Moreover, future studies should focus on investigating the magnitude of cardiovascular effects arising from the interaction of different environmental risk factors, especially from the possible synergistic effect between pollution and medical ionizing exposure.
In general, combined environmental exposure may be a potential pathological contributor that may accelerate the physiological shortening of telomeres and induce premature senescence of cells, which may be causally linked to the acceleration of the aging process and the high morbidity and mortality of age-related diseases [29].
In recent decades, genetic research has produced compelling evidence regarding the variability of individual responses to IR or other environmental toxicants, demonstrating that some subjects are more susceptible than others. With this in mind, future studies on low-dose IR exposure should also determine and identify genetic factors that can substantially impact the individual response to CVDs risk.
Finally, molecular epidemiological studies should further elucidate the complex underlying biological mechanisms of radiation-induced cardiovascular injury at low doses, including the epigenetic regulation mechanisms, such as DNA methylation, histone and chromatin modifications, as well as the expression of non-coding RNAs, which can alter gene expression without altering DNA sequences [30].
This future research will contribute to a more comprehensive understanding of cellular response to radiation and the identification of target molecules or biomarkers that can be used for improving surveillance with screening protocols and preventive measures able to protect the most vulnerable patients.
Abbreviations
CT: |
computed tomography |
CVDs: |
cardiovascular diseases |
DDR: |
deoxyribonucleic acid damage response |
DNA: |
deoxyribonucleic acid |
DSBs: |
double-strand breaks |
Gy: |
Gray |
IR: |
ionizing radiation |
mSv: |
millisieverts |
PET: |
positron emission tomography |
ROS: |
reactive oxygen species |
SASP: |
senescence-associated secretory phenotype |
Sv: |
sievert |
Declarations
Author contributions
MGA: Conceptualization, Investigation, Writing—original draft, Writing—review & editing.
Conflicts of interest
The author declares that she has no conflicts of interest.
Ethical approval
Not applicable.
Consent to participate
Not applicable.
Consent to publication
Not applicable.
Availability of data and materials
Not applicable.
Funding
Not applicable.
Copyright
© The Author(s) 2023.