Abstract
Left ventricular ejection fraction is the critical parameter used for heart failure classification, decision making and assessing prognosis. It is defined as a volumetric ratio and is essentially a composite of arterial and ventricular elastances, but not intrinsic contractility. The clinician should be aware of its numerous limitations when measuring and reporting it. And make a step toward more insightful understanding of hemodynamics.
Keywords
Left ventricular ejection fraction, ventriculo-arterial coupling, left ventricular functions, hemodynamicsIntroduction
Left ventricular ejection fraction (LVEF) is the critical parameter used heart failure (HF) [1]. Today, more than 50 years after the first reports describing it [2], LVEF likely remains the most used parameter to assess LV systolic performance, because of the simplicity of its calculation by whatever non-invasive imaging method, the ease of understanding it on the part of clinicians (cardiologist or otherwise), and its relevance to clinical decision-making and prognosis. Its core clinical value is to define HF with reduced ejection fraction (HFrEF) that triggers the use of neurohormonal medications that improve outcomes [1].
The clinician should consider its limitations and whenever possible, make use of other parameters to further characterize the hemodynamics of patients with heart disease.
LVEF definition
LVEF represents the proportion of diastolic volume ejected during ventricular contraction and is calculated as a dimensionless ratio between stroke volume (SV) and end-diastolic volume (EDV) [3].
LVEF measurement
LVEF calculation is possible using invasive angiographic methods (which used to be considered the gold standard) or by widely available non-invasive imaging. Each technique has its draw-backs and sources of error, and there are differences in methodologies and algorithms.
Most experts calculate LV volumes based on the geometrical approximation of the normal ellipsoid shape of the LV, which becomes a source for error when LV remodeling in the context of eccentric hypertrophy (i.e. mode M derived LVEF [4] or the area-length method). Two-dimensional (2D) echocardiographic calculation of the LVEF using the recommended Simpson method of disks [3] is easy to perform, using widely available echo machines; nonetheless accuracy suffers from image quality.
These issues have led to the development of less geometrical methods and techniques which rely on good border delineation, including three-dimensional (3D) echocardiography or computer tomography (CT). On the other hand, improvement of endocardial border delineation with contrast 2D echocardiography has been shown to derive more reproducible values [5], more similar to cardiac magnetic resonance (CMR).
There is generally good agreement between methods, as described in Table 1 [5–10], even though the same studies showed that LV volumes tend to be smaller when compared to CMR imaging and had significant variability. The same applies for radionuclide techniques: in a large community study, Single-Photon Emission Computed Tomography (SPECT) did not correctly discern between the left atrium and LV, yet the sensitivity and specificity for determining LVEFs of < 40% were 90% and 86%, respectively [11].
Comparison of LVEF as measured by different non-invasive imaging methods, reported mean difference and agreement
Echo 3D vs. CMR | SPECT vs. CMR | MDCT vs. CMR | Echo3D vs. SPECT | |
---|---|---|---|---|
Mannaerts et al. 2003 [6] | MD 1.8% ± 11.6% | |||
Persson et al. 2005 [7] | MD 4% ± 7% (P < 0.001) | |||
Belge et al. 2006 [8] | MD 1% ± 21% (P = NS) r = 0.95; P < 0.001 | |||
Soliman et al. 2008 [9] | MD 0.2% ± 6.2% | |||
Beitner et al. 2019 [10] | MD 0.56% ± 15% | MD 0.75% ± 10% | 1.3% ± 20% | |
ICC 0.71 | ICC 0.89 | ICC 0.51 | ||
β = 0.72, P = 0.003 | β = 0.90, P < 0.001 |
Echo2D: bidimensional echocardiography; Echo3D: three dimensional echocardiography; MDCT: gated multidetector row computed tomography; MD: mean difference; NS: not significant; r: correlation index; ICC: intraclass correlation coefficient
CMR has become the new gold standard, against which all methods are compared, due to the excellent image resolution and thus accurate border detection, good reproducibility, low test-retest variation [10–12].
Nonetheless, echocardiography can correctly identify patients with depressed and non-depressed LVEF [5, 11]. Echocardiographic calculation of stroke volume by measuring the velocity time integral, in the absence of left sided-regurgitation, can be a fair practical method to overcome the difficulties of delineating trabeculations in a small LV cavity at end-systole.
Normal range of 55% to 65% for LVEF
Defining the normal range of values is an overlooked issue in clinical practice.
First of all, the aforementioned differences in methodology and algorithms between diagnostic modalities make unlikely a fixed value [5]. Wood et al. [5], observed in a 2014 analysis that the reference values are based on studies involving cohorts as low as 60 patients ranging up to 1, 200, suggesting that further validation studies are required.
Second, abnormal LVEF values were clearly defined below 50%; the dismal prognostic value of abnormal LVEF was observed in several cohorts [13–15]. Asymptomatic left ventricular systolic dysfunction defined as LVEF < 50%, although uncommon (up to 2% [14]) was an early marker of evolution toward severe congestive HF and death, nearly five-fold higher than in control subjects in the multi-ethnic study of atherosclerosis study [14].
The borderline gray zone between 50% and 55% has been considered the lower range of normal values. A recent study by Tsao et al. [16], showed that persons with LVEF of 50% to 55% in the community have greater risk for morbidity and mortality relative to persons with LVEF > 55%. This led Fonarow and Hsu to question the paradigm and suggest that LVEF of 50% to 55% should not be considered within the “normal” range [17].
Third, there are recognized age, gender, and ethnicity differences. CMR data showed that women have higher LVEF than men in the general population, with higher SV for a given EDV, independent of known potential confounders (low LVEF defined as below the 2.5th percentile of a healthy subset was 61% in women and 55% in men) [18]; current guideline recommendations describe separate cutoffs [3]. A very large echocardiographic study in more than 8, 000 healthy volunteers < 70 years suggested ethnic-specific cutoffs for EF normal ranges [19]. LVEF was also shown to increase with age [20, 21], with significant difference between the extreme age groups, more pronounced in females (as demonstrated in one large population study 62% ± 0.5% [age 7–20 years] vs. 65% ± 0.3% [age 60–80 years] [20]).
A fourth issue involves the physiologic variations of LVEF.
During exercise, increased LVEF was demonstrated. Athletes express lower rest EF values (52.9% ± 5.86% compared to non-athletic controls 58.2% ± 6.05%, P < 0.001), but with more significant ΔLVEF increase of 16.5% ± 8.51% during exercise and with greater LV contractility reserve vs. non-athletes [22].
There is also evidence for circadian and sleep-induced LVEF changes. A small study in the 1990s identified a temporary (up to 30 min) dramatic decrease of scintigraphy-measured LVEF at waking up in the morning, to as low as < 30% in some normal subjects [23]. The observation was not further investigated; however, given the recognized circadian changes in all other hemodynamic determinants of cardiac performance, it is to be regarded as noteworthy.
Large population studies show a Gaussian distribution of LVEF, with a mean value around 62% (measured by echo or CT), which is probably ideal [20, 24]. Another recently published analysis of a very large clinical dataset (403, 977 echocardiograms from 203, 135 unique patients), emphasizes the presumably “physiological” range of 60–65%, which is associated with best survival; it also suggests the existence of a supra-normal LVEF phenotype associated with reduced survival [25].
LVEF is not an intrinsic contractility parameter
LVEF is regarded as an essential measure of LV systolic function [3], even though mathematical reasoning and early experimental work [26] have shown it is load-dependent.
Nonetheless, early experimental work on the sarcomere length-tension curve and Starling’s law for heart contraction revealed a good correlation between sarcomere performance and EF [27]. More recent studies of structural and functional reverse remodeling reveal good correlation between changes in gene expression and LVEF [28, 29], more so than to other hemodynamic parameters, thereby demonstrating the ability of LVEF to reflect the contractility state of the myocardium [30].
In the search of a more integrative hemodynamic perspective, cardiac physiologists turned toward analysis of pressure-volume loops (PVL). This provides the basis for the calculation of arterial (Ea) and ventricular (Ees) elastances. Their ratio (Ea/Ees) represents the ventriculo-arterial coupling (VAC); its diagnostic and prognostic value has recently been recognized once more, in a consensus document of the Heart Failure Association [31]; this is particularly relevant for HFrEF.
Although accurate measurements require invasive techniques (methods and updated clinical utility are briefly described in a review by Bastos et al. [32]), Chen et al. [33], and Shishido et al. [34], introduced the “single-beat” Ees estimation derived from non-invasive studies.
The mathematical formulas are complicated; however, current widespread technology can compute these easily (i.e. including the iPad App simulation of PVL [35]) and could gain clinical utility [36].
Kass et al. [37], and Robotham et al. [38], have published on the relationship between LVEF and Ea and Ees, defining EF by the following formula: LVEF = Ees[1 − (Vo/EDV)] / (Ea + Ees), where Vo = the volume intercept at 0 mmHg pressure [37].
It becomes understandable that LVEF results from permanent cross-talk between Ees and Ea, being subordinated to the VAC and explaining the lesser sensitivity of the LVEF to contractility itself [39]. Although, not a strong contractility discriminator, when regarded as a composite result of Ees and Ea, LVEF is a powerful predictor [39]. By mathematical consequence, for a considerable increase of the contraction force, a mild increase in Ea, demonstrates a net blunting effect over the final LVEF augmentation, LVEF being thus predominantly afterload dependent, especially with decreased contractility [39] and experimental work already confirmed this [37, 40]. Therefore, the understanding of left ventricular contractility should not rely only on the calculation of LVEF.
As pointed out by Kass et al. [37], in a pivotal article, PVL analysis allows for a more complete characterization of pump performance, separating loading factors from ventricular properties and, relevant for critical care practice, it enables the prediction of stroke volume and stroke work response to various interventions. In this context, Ees was adopted as an index of intrinsic LV contractility [41, 42]; it remains imperfect, being a time-varying non-linear function derived from single-point steady state formulas.
For patients with HF with preserved EF (HFpEF), EF cannot be discriminatory enough for the severity of the myocardial disease; the same is true for VAC as a dimensionless index alone; the integrated analysis of the parameters relating to structural changes and describing the coupling of LV and arterial system, including the absolute values of Ea, arterial compliance, Ees, Vo (Vo is the theoretical volume intercept at 0 mmHg pressure and correlates as well to contractile function [43]), end-diastolic volume could further differentiate the severity of hypertrophied LV when facing high afterload (e.g., in hypertension or aortic stenosis) [44–46].
The influence of heart rate on PVL is often neglected; it influences cardiac contractility by the force-frequency relation; however, it is also a major contributor to Ea, as shown by Segers et al. [47], in a mathematical heart-arterial interaction model.
An important contractility determinant is LV geometry. The variations in LV shape and size that occur with pathological remodeling, reflected by the LV volumes [both end-systolic (ESV) and EDV], decrease contractile efficiency and predict outcome [44, 48]. ESV is one of the essential components of Ees and VAC, while having a non-linear relationship with EF, as described in pivotal work by Kerkhof et al. [49, 50], on the volume regulation graph (VRG). Any change in the inotropic state will impact ESV [49, 50]. Based on the premises, Kerkhof [44] and Mann et al. [48], proposed plotting LVEF as a function of end-systolic volume index, for a more accurate description of LV working point and improved classification of HF.
Monge García et al. [40], also acknowledge the limitations of LVEF as a contractility discriminant and propose an afterload (Ea)-adjusted LVEF; using the square root of Ea would account for the greater impact of afterload on VAC at low levels of contractility and significantly improve the ability to track LV contractility: LVEF Ea = [square root of (PesXSV)] / EDV [40], which is not dimensionless, in contrast to the traditional EF.
PVL and the derived VRG could further deepen the hemodynamic understanding of LV systolic function (Figure 1 depicts various interventions on the LV and the subsequent change in PVL).
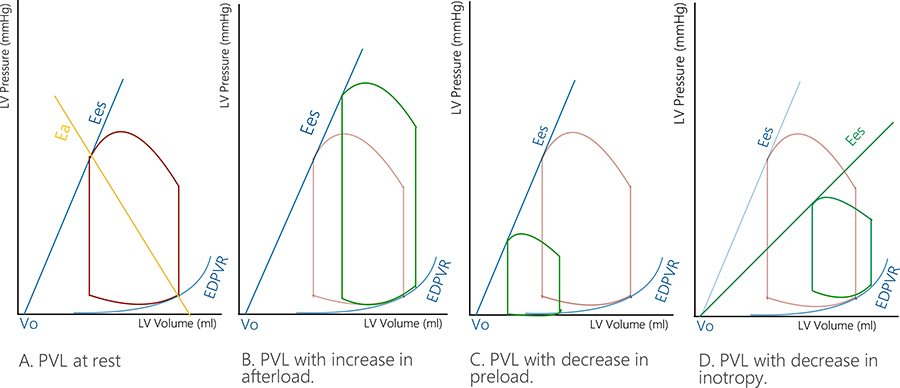
Various interventions on the PVL loop. A. LV PVL at rest; B. Increase in afterload (the PVL changes to the right and upwards); C. Decrease in preload (the PVL changes to the left and downwards); D. Decrease in inotropism (the PVL will change downwards and to the left)
Searching for other contractility indices
There has been a consistent search for contractility parameters other than LVEF.
The maximum rate of left ventricular pressure rise (dP/dt) and its relation to EDV as described by Little [51] was very sensitive to inotropic stimulation, independent of the load.
Tissue Doppler Imaging (TDI) has been used for the past 30 years and validated as a sensitive tool for subclinical detection of longitudinal dysfunction for both left and right ventricles [52, 53].
Seo et al. [54], in an animal study, compared the S’ wave and LVEF against LV dP/dt: S’, reflecting both LV longitudinal shortening and torsional deformation proved to be a more sensitive index of global LV contractility than LVEF, as it had a much stronger correlation to dP/dt than LVEF (r = 0.665 vs. r = 0.40, respectively).
Beyond the measurement of TDI wave amplitude, precise time interval measurement is sometimes a neglected advantage. Myocardial performance index (MPI), derived by measuring systolic and diastolic time intervals using TDI M-mode through the mitral valve, was significantly associated within invasive (dPmax/dt) and non-invasive (LV global longitudinal strain and strain rate) measures of LV systolic function; conventional measurement of MPI (using pulsed wave in the left ventricular outflow tract) was less reliable [55].
The superiority of myocardial deformation parameters (strain, strain rate) in risk assessment and as sensitive contractility indices have extensively been reviewed whenever the limitations of LVEF as a contractility marker are discussed [12, 56] and are now integrated into the essential recommendations for the echocardiographic assessment of LV function [3].
No less significant is the evaluation of LV contractile/flow reserve (as the ability to augment its performance or provide adequate SV augmentation according to inotropic stimulation) [39, 57]. SV augmentation during dobutamine stress echo was found to be a powerful predictive parameter for good surgical outcomes in very high-risk surgery whether undergoing LV remodeling procedures [58] or aortic valve replacement [59].
The most important clinical limitation of LVEF is its inability to describe the HFpEF population. The hemodynamics of these patients is presumably better described using the VAC (which has normal or lower than normal values), and even more so by the absolute values of Ees (which are generally elevated) and the correspondingly abnormally elevated value of Ea at rest [60].
The interaction of the LV with the other cardiac chambers is equally important. Left atrial enlargement due to pressure and volume overload causes structural changes in the other chambers, including concomitant tricuspid annulus dilation, increased mobility of the tricuspid leaflets tricuspid regurgitation, and decreasing RV performance. This issue was addressed in detail by Zakir et al. [61], who demonstrated the correlation of LV diastolic function with RV systolic dysfunction, based on the invasive measurement of the pulmonary venous system. LV diastolic dysfunction causes increased left atrial filling pressure, that can be transmitted backward, leading to pulmonary arterial hypertension and RV pressure overload. Brand et al. [62], demonstrated that systolic right heart function is significantly altered in patients with diastolic dysfunction and enlarged left atrium.
Conclusion
Although LVEF is essentially a volumetric index, it remains a powerful clinical parameter of LV myocardial function that represents the interplay between LV contractile state and the arterial afterload. To be able to adequately utilize it in clinical practice, the clinician should acknowledge its limitations as a contractility marker, the pitfalls that occur with measurement for the various imaging techniques and methods, and the significance of normal values. EF, can only provide limited information on cardiac performance; therefore, a more in-depth understanding of hemodynamics should always be sought.
Abbreviations
CMR: | cardiac magnetic resonance |
CT: | computer tomography |
dP/dt: | maximum rate of left ventricular pressure rise |
Ea: | arterial elastance |
EDV: | end-diastolic volume |
Ees: | ventricular elastance |
EF: | ejection fraction |
ESV: | end-systolic volume |
HF: | heart failure |
HFpEF: | HF with preserved EF |
HFrEF: | HF with reduced EF |
LV: | left ventricle |
LVEF: | left ventricular ejection fraction |
MPI: | myocardial performance index |
Pes: | end-systolic pressure |
PVL: | pressure volume loop |
SPECT: | single-photon emission computed tomography |
SV: | stroke volume |
VAC: | ventriculo-arterial coupling |
Vo: | volume axis intercept for 0 mmHg |
VRG: | volume regulation graph |
Declarations
Author contributions
ELA wrote the first draft of the manuscript. All authors contributed to manuscript revision, read and approved the submitted version.
Conflicts of interest
The authors declare that they have no conflicts of interest.
Ethical approval
Not applicable.
Consent to participate
Not applicable.
Consent to publication
Not applicable.
Availability of data and materials
Not applicable.
Funding
Not applicable.
Copyright
© The Author(s) 2020.