Abstract
Over the past few decades, it has become clear that an excessive activity of matrix metalloproteinases (MMPs) can accelerate the progression and fatal outcomes of several serious age-related diseases, including atherosclerotic coronary heart disorders and various types of malignancies. These proteolytic enzymes mediate the degradation and remodeling of the extracellular matrix through cleaving its various components, thereby affecting many critical functions of surrounding cells and intercellular communication. Consequently, the low expression levels of MMPs can be important in the prevention and treatment of such chronic life-threatening pathologies, contributing to the better quality of life and longer life expectancy. In this review article, the pathogenic proteolytic roles of MMPs are examined in more detail, especially in the cases of heart attack and stroke as well as cancer invasion and metastasis, showing that these enzymes can be considered not only as diagnostic and prognostic biomarkers but also as important therapeutic targets in the fight against many age- and lifestyle-related serious disorders. The identification and development of suppressing agents with a selective activity towards specific MMPs have, however, still remained a complex and complicated challenge, in which natural plant-derived compounds are increasingly recognized as promising leads for the new-generation inhibitors.
Keywords
Matrix metalloproteinases, proteolytic enzymes, atherosclerosis, heart attack, cancer, angiogenesis, metastasis, longevityIntroduction
Matrix metalloproteinases (MMPs), also known as matrixins, belong to a family of multidomain zinc-dependent endopeptidases which are capable of degrading extracellular matrix (ECM) through cleaving various connective tissue components [1–4]. MMPs were discovered in 1962 by Jerome Gross and Charles M. Lapiere while exploring the degradation of triple-helical collagen during the resorption of the tail of metamorphosing tadpole. The collagen was cleaved by an enzyme known as interstitial collagenase, and this enzyme was first isolated from the human skin in 1968 [2–5]. Over the next few decades, MMPs were isolated and purified from both vertebrates and invertebrates as well as plants, whereas their phylogenetic origin is considered to be lower organisms, namely Bacteroides fragilis [2, 4].
MMPs play a pivotal role in remodeling ECM via controlling the formation and degradation of its major components [6]. The ECM presents an extracellular element of tissues that provides structural and biochemical support for cells and storage for growth factors, besides regulating cell migration, intercellular interactions and communication [2, 5, 6]. The ECM has many important components, including fibers (e.g., collagen, elastin, laminin, and fibronectin), proteoglycans, glycoproteins and polysaccharides, with collagen being the most abundant protein in the ECM [2, 6]. MMPs act specifically on different segments of the ECM, targeting a variety of proteins, such as collagen, gelatin, elastin and casein [3, 4, 6]. Collectively, these enzymes can break down most of the components of the ECM [1, 4]. In doing so, MMPs may not only degrade the ECM components, but indirectly act also as activators for biologically important molecules and processes [4].
To date, 28 different types of MMPs have been described in vertebrates, among which at least 23 are expressed in human tissues [2, 5]. On the basis of their substrate specificity, sequential similarity and structural organization, these enzymes can be classified into several subgroups, i.e., collagenases (MMP-1, MMP-8, MMP-13), gelatinases (MMP-2, MMP-9), stromelysins (MMP-3, MMP-10, MMP-11), matrilysins (MMP-7, MMP-26), membrane-type MMPs (MT-MMPs; MMP-14, MMP-15, MMP-16, MMP-24), and other MMPs [2, 4, 6]. The most important role of collagenases is the cleavage of triple-helical fibrillar collagens in their native state at neutral pH [2, 4]. Gelatinases can primarily degrade denatured collagen (gelatin) and certain types of intact collagens, besides cleaving also laminin, elastin, fibronectin and proteoglycan core proteins, being thereby importantly involved in the breakdown of basal lamina [2–4]. Stromelysins are able to degrade mainly the non-collagenous ECM proteins, such as proteoglycans, glycoproteins, fibronectin and laminin, and can cleave even the other MMPs [4]. The main difference of MT-MMPs from other matrixins is their association with the cell membrane [3, 4]. The activation of MMPs is triggered by the proteolytic removal of the cysteine-containing propeptide domain that interacts with zinc ion in the catalytic site to keep the enzyme in an inactive state. This mechanism is known as the cysteine switch [1, 2, 4].
The proteolytic action of MMPs is controlled at multiple levels, including the gene expression and protein secretion as well as the regulation of enzymatic activities [1, 2, 4]. The production of certain MMPs can be induced by different exogenous signals, including cytokines, inflammatory mediators, growth factors, hormones and changes in intercellular interactions [2, 4]. Once activated, the tissue inhibitors of metalloproteinases (TIMPs) cause the inhibition of MMPs, indicating that the degradation of basement membranes is determined not only by the amounts of proteolytic enzymes expressed in tissues, but by the balance between activated proteases and their naturally occurring inhibitors [1, 4, 7]. There are four TIMPs currently identified (TIMP-1, TIMP-2, TIMP-3, TIMP-4), which are responsible for the regulation of the activities of different MMPs and the prevention of an excessive matrix degradation [1, 4]. Moreover, the activation of MMPs can be provoked also by some physiochemical factors, such as heat, low pH, chaotropic agents and thiol-modifying substances, inducing the disruption of cysteine-zinc coordination in enzyme molecules [2, 4].
The role of MMPs in normal physiological processes
Followed by their discovery, the MMPs research has been focused on the function of these proteolytic enzymes in the ECM degradation and remodeling within homeostatic balance and imbalance [4, 5]. These studies have convincingly demonstrated a crucial role of different MMPs in multiple physiological processes, including reproductive events (e.g., menstrual cycle, ovulation, endometrial proliferation, involution of uterine, breast and prostate), embryonic growth and development, tissue morphogenesis, osteogenesis, angiogenesis and tissue repair; affecting the cellular differentiation, migration and phenotype, and altering the intercellular communication [2, 4, 5]. MMPs are indeed produced in various tissues and cells, being secreted by connective tissue and diverse proinflammatory and uteroplacental cells, such as fibroblasts, osteoblasts, endothelial cells, vascular smooth muscle cells, macrophages, lymphocytes and neutrophils [2, 4].
For example, during the wound healing process, different types of MMPs are involved in many cellular, molecular and biochemical events. On the one hand, the neoepithelium formation is significantly impaired by blocking the activity of certain MMPs. The specific matrixins responsible for this re-epithelialization process are, however, still largely unknown [5]. On the other hand, without the protective epithelium, the susceptibility to infections is increased and the selective suppression of the pathogenic MMPs may present a therapeutic option for the treatment of non-healing chronic cutaneous wounds [5]. Therefore, the role of redundancy in complex and interconnected network of MMPs definitely needs further in-depth investigations.
The role of MMPs overexpression in pathological processes
Over the recent years, a great number of studies have demonstrated the involvement of MMPs overexpression in the pathogenesis of several serious diseases [2]. In fact, the elevation of MMPs activity with an excessive degradation of the ECM can lead to the development and progression of diverse metabolic and immune diseases, neurodegenerative illnesses, cardiovascular disorders and cancers [2, 4, 6]. Consequently, specific MMPs have been recently recognized as diagnostic, monitoring and prognostic biomarkers for several diseases involving the ECM disruption [2, 4, 6]. These are the pathologies associated with the tissue destruction (e.g., inflammatory conditions, arthritis, ulcers, brain degenerative disorders, and cancer metastasis), fibrosis (e.g., fibrotic lung and liver diseases, multiple sclerosis, and atherosclerosis) and matrix weakening (e.g., varicose veins, dilated cardiomyopathy, and aortic aneurysm) [2, 4–6]. For example, overproduction of different MMPs in synovial membranes, cartilage, tendon and bone of synovial joints is often correlated with the tissue destruction in both rheumatoid arthritis and osteoarthritis [4]. Elevated levels of certain MMPs and their dysregulated activities are associated with several diabetic comorbidities, such as diabetic retinopathy, nephropathy and chronic non-healing ulcers. The chronic inability of wounds to heal due to an excessive degradation of the ECM by overexpressed MMPs is a serious consequence of diabetes, besides several cardiovascular events [2, 6]. Sepsis is also a potentially life-threatening condition related to the tissue remodeling mediated by MMPs following the pathogen invasion. A positive correlation between the levels of certain MMPs (e.g., MMP-1, MMP-2, MMP-8, MMP-9, and MMP-10) and poor prognosis has been indeed described in septic patients [6]. In addition, the ECM remodeling by different MMPs has been demonstrated to be involved also in a variety of central nervous system disorders. The ECM degradation due to the MMPs activity is critical for blood brain barrier leakage resulting in edema and eventually cerebral ischemia and stroke, and can promote also the formation of multiple sclerosis lesions [6]. Specifically, the levels of MMP-9 have been found to be higher in acute ischemic stroke patients, being correlated with ongoing brain ischemia and infarct size, severity of stroke and poor functional outcome [6]. Moreover, different MMPs can present also as biomarkers for the pathogenesis and progression of neurodegenerative disorders, such as amyotrophic lateral sclerosis, Alzheimer’s disease and Parkinson’s disease [3, 6]. The role of these proteolytic enzymes in diverse neurological conditions is, however, still rather under-investigated and needs further extensive studies [3].
In the following subsections, the role of MMPs overexpression on the pathogenesis of two most prevalent and dreadful chronic degenerative disorders, i.e., atherosclerotic coronary heart diseases and cancers are described in more detail.
Upregulation of MMPs as a promoting factor for heart attack and stroke
Atherosclerotic coronary heart diseases are currently one of the major causes of death around the world, especially in industrialized societies [8–10]. These are the disorders that develop silently during many years until sudden and potentially life-threatening clinical manifestations [11]. Atherosclerosis is a complex multifactorial pathology characterized by degenerative changes in the wall of large arteries, involving endothelial dysfunction, reactive oxygen species (ROS)-dependent oxidation of low-density lipoproteins (LDL), vascular inflammation, accumulation of foam cells, and the migration and proliferation of vascular smooth muscle cells [9, 12, 13]. The formation of atherosclerotic plaque from cholesteryl esters-containing foam cells in the subendothelial space is accompanied by thickening, narrowing and hardening of blood vessels, decreasing or even impeding the blood flow into various organs and tissues, especially to the vital organs such as the heart and lungs, ultimately leading to serious cardiovascular events [8, 12]. Being a chronic inflammatory disease, the onset and progression of atherosclerotic changes are closely related to several factors such as increasing age, smoking, obesity, abnormal lipid metabolism, hypertension and diabetes mellitus, whereas the excessive accumulation of lipids in the arterial wall represents a key step of atherosclerotic processes [8, 9, 14].
It is well accepted that atherosclerosis predisposes patients to acute myocardial infarction (heart attack), stroke or sudden death [8, 12]. In fact, atherosclerotic lesions are characterized by a dense fibrous cap being constituted by the proliferative vascular smooth muscle cells and their produced ECM proteins (collagen, elastin, fibronectin) to cover the lipids-containing core [9, 12, 14, 15]. If such a fibrous cap becomes unstable in response to diverse surrounding stimuli such as inflammatory mediators, the upregulation and secretion of different MMPs (MMP-1, MMP-2, MMP-9, MMP-13, MMP-14) in the involved cells (i.e., smooth muscle cells, endothelial cells, and macrophages) render the atherosclerotic plaque susceptible to the spontaneous rupture [12, 14, 16] (Figure 1). This proceeds from the ability of MMPs as proteolytic enzymes to degrade the interstitial collagen of the protective plaque fibrous cap, thereby weakening its biomechanical strength and structural integrity [16–18]. The plaque rupture further leads to the activation, adhesion and aggregation of platelets, ultimately resulting in the formation of thrombus that can occlude the vessels and produce tissue ischemia [11, 14, 18]. Therefore, the inherently low levels of MMPs may be protective against the plaque rupture through the maintenance of atherosclerotic plaques in a stable state, being important for the prevention of atherothrombosis. Also, the therapeutic strategies that downregulate the expression and activity of MMPs might be useful in avoiding severe acute cardiovascular complications, thereby providing a new target for the blockage or treatment of atherosclerosis. Such compounds include, for example, some natural agents, in particular specific flavonoids [12, 19, 20]. In this context, it is interesting to mention that although estrogens are generally considered to be protectors against atherosclerosis, these natural steroid hormones can still act as potential disruptors of established plaques. This is in opposite to phytoestrogenic isoflavones which seem to be not deleterious for the advanced stages of the disease [17].
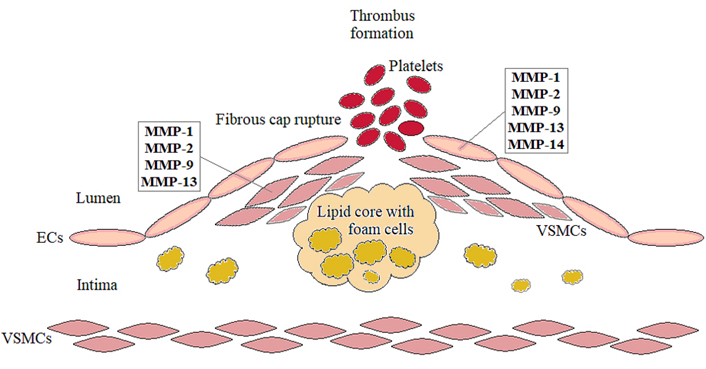
The role of MMPs in the fibrous cap rupture during the progression of atherosclerosis leading to potentially life-threatening sequelae. ECs: endothelial cells; VSMCs: vascular smooth muscle cells
Upregulation of MMPs as a promoting factor for tumor angiogenesis and metastasis
Malignant tumors have remained one of the most dreadful diagnoses worldwide being still often incurable. These tumors generally develop through a multistep process starting from the accumulation of transformed cells due to diverse endogenous and exogenous causes, accompanied by gradually acquiring the ability to invade into the adjacent tissues and form distant metastases throughout the body [21]. There are several mechanisms allowing cancer cells to grow and spread, including evasion of cell cycle arrest and apoptosis, induction of angiogenesis, epithelial-mesenchymal transition (EMT) and degradation of the ECM [21, 22]. These events facilitate the migration and invasion of malignant cells through the basement membrane and ECM, intravasation, survival in the bloodstream, and extravasation and the formation of secondary tumors at distant sites [21, 22].
Tumor angiogenesis is a complex process under which new blood vessels are formed from the pre-existing ones to provide nutritional support to the neoplastic tissue [23, 24]. Actually, without angiogenesis, tumors cannot grow to a size more than about 1–2 mm3, due to the diffusion limit of oxygen and nutrients [25]. After receiving excessive proangiogenic stimuli from tumoral cells, such as vascular endothelial growth factor (VEGF) or fibroblast growth factor (FGF), an angiogenic switch occurs and resting endothelial cells activate the gene expression to provoke new vessel development [24–26]. This involves the production of proteolytic MMPs, mostly MMP-2 and MMP-9, which promote a local degradation of the vascular basement membrane and ECM, followed by the migration and proliferation of endothelial cells into the surrounding stroma and ultimately, the maturation and stabilization of the new blood vessels [22, 25, 26] (Figure 2). The major ECM components found in endothelial cell basement membrane and interstitial spaces include fibronectin, laminins, elastin and collagens, all degraded by MMPs during angiogenesis [26]. However, differently from normal vasculature, the resultant vascular network in tumors is often disorganized, tortuous and anarchic, inducing an uneven distribution of drugs throughout the malignant tissue [22]. Accordingly, the suppression of angiogenesis in its different steps has been highlighted as a promising new strategy against cancerous neoplasms, termed as antiangiogenic therapy [25, 27]. Besides, downregulating the levels of proangiogenic factors such as VEGF, its receptors and downstream signaling molecules, MMPs represent an important target to impede angiogenesis and restrict tumor progression [24–26]. However, no MMPs-directed angiogenic inhibitors are still clinically available to date. Nevertheless, several natural compounds, especially some plant-derived flavonoids and chalcones (e.g., quercetin, silibinin, and isoliquiritigenin), have been demonstrated to behave as potential antiangiogenic agents in preclinical settings, through suppressing the expression and activity of MMPs in tumor-associated endothelial cells [24–26].
Furthermore, angiogenesis plays an important role also in cancer metastasis as the new blood vessels present the way through which cancer cells can leave their original site [22, 28]. Specifically, to reach the blood circulation, tumor cells must first destroy matrix barriers and invade through the basement membrane and ECM, by upregulating the expression and release of MMPs, mostly MMP-2 and MMP-9 [21]. The production of these enzymes is indeed shown to be increased in various types of malignant cells, correlating with their invasive/metastatic potential [1]. In addition, MMPs are crucial also in modulating the vascular permeability and forming secondary lesions after extravasation, degrading ECM in the metastatic microenvironments [21] (Figure 3). These proteolytic enzymes may therefore be considered as potential targets for antimetastatic therapies [1]. After implantation into the distant organ, tumor cells resume all the process of enhanced proliferation and angiogenesis, beginning to form a new metastatic tumor [22]. Consequently, metastasis represents the most serious complication of carcinogenesis, being the primary cause of death of cancer patients [21]. Reducing the levels of MMPs secreted by cancerous cells as well as various cells in tumor microenvironment, including tumor-associated endothelial cells, may thus be important for impeding the tumor aggressiveness and retarding malignant progression, keeping primary tumors as well as metastatic lesions in a dormant state as long as possible. Despite intensive efforts, no inhibitors of MMPs have been approved as anticancer therapeutics for the clinical use so far [1]. Nevertheless, in preclinical studies, several natural compounds, especially structurally different plant polyphenols, have been demonstrated to be potent blocking agents for these proteolytic enzymes through regulating multiple intracellular signaling pathways, thereby impairing the invasive potential of diverse types of malignancies [1, 21, 22, 24, 27].
Current strategies to downregulate MMPs
Due to a crucial role of MMPs in the ECM degradation and remodeling, and the association of MMPs overexpression with the pathogenesis and progression of several serious diseases, these proteolytic enzymes must be tightly controlled to prevent their detrimental activities [4–6]. Accordingly, over the past few decades, MMPs have been intensively explored as important therapeutic targets for the prevention and treatment of various pathologies involving the ECM disruption, especially different cancer types and coronary heart disorders, using a wide range of synthetic MMP inhibitors (MMPIs) [5, 6]. Several pharmacological approaches have been examined to suppress the MMPs activity, such as TIMPs, small-molecule MMPIs and inhibitory antibodies, applying also modern biotechnological tools [4, 6]. Unfortunately, despite some success in preclinical models, the randomized controlled trials with small-molecule MMPIs have revealed many disappointing results related to their poor oral bioavailability, lack of efficacy, dose-limiting toxicities and adverse side effects [5, 6]. The first-generation synthetic MMPIs were designed to mimic the natural substrates of MMPs; however, these inhibitors prevented the functions of various types of MMPs and inactivated also other proteinases unrelated to the disease but necessary for normal physiological events [5, 6]. Such setbacks from the clinical studies with broad-spectrum MMPIs clearly emphasized the extreme complexity of MMPs activities in diverse biological processes and showed a high need for the identification and development of MMPIs with a narrower specificity [5, 6]. Currently, no approved MMPIs are still available, with the only exception of a low-dose oral doxycycline (Periostat®) that is used as an adjunct for the treatment of adult periodontitis, in virtue of its ability to inhibit MMP-8 collagenase [5].
In the recent years, structurally different natural compounds, especially plant-derived flavonoids, have been demonstrated to act as potent blocking agents for MMPs in numerous preclinical models, opening a new avenue for the design of more selective and safer new-generation inhibitors for these proteolytic enzymes [29, 30]. Further randomized controlled trials with such lead compounds are required to determine their real clinical potential. Nevertheless, the current promising results clearly suggest that the regular dietary intake of flavonoid-rich products, i.e., fruits, vegetables, legumes, grains, nuts, spices and medicinal herbs, could be essential in suppressing the expression and activity of different MMPs, thereby preventing the pathogenesis and progression of a variety of severe diseases involving the disruption of ECM.
Conclusions
On the whole, as the overexpression of MMPs in diverse human tissues is closely related to the development of different chronic degenerative diseases, including various types of malignancies, heart attack and stroke, maintaining the levels of these proteolytic enzymes under control is essential for a good health. Therefore, low expression of MMPs might be considered to be a key for longevity through preventing the pathogenesis and progression of age- and lifestyle-related serious diseases. Although the results of the current synthetic inhibitors have been disappointing due to their broad specificity and undesired side effects, one possible strategy to keep the levels of MMPs steadily low is the regular consumption of food products rich in natural MMP inhibitors, such as plant polyphenolic flavonoids. This approach may suppress the ECM degradation and thereby reduce the risk of atherosclerotic plaque disruption and tumoral spread, improving the quality of life and extending the life expectancy. Further studies are definitely needed to unravel the precise molecular mechanisms under MMPs activities and improve the knowledge about diverse biological roles of these proteolytic enzymes in the development of diverse diseases, allowing to design more selective and safe inhibitors for the clinical use, preferably proceeding from the promising results with naturally occurring lead compounds.
Abbreviations
EC: | endothelial cell |
ECM: | extracellular matrix |
MMP: | matrix metalloproteinase |
MMPIs: | matrix metalloproteinase inhibitors |
TIMPs: | tissue inhibitors of metalloproteinases |
Declarations
Author contributions
KS: Conceptualization, Methodology, Writing—original draft, Writing—review & editing.
Conflicts of interest
The author declares that she has no conflicts of interest.
Ethical approval
Not applicable.
Consent to participate
Not applicable.
Consent to publication
Not applicable.
Availability of data and materials
Not applicable.
Funding
Not applicable.
Copyright
© The Author(s) 2024.