Abstract
The dynamic of the virus-host interaction is subject to constant evolution, which makes it difficult to predict when the SARS-CoV-2 pandemic will become endemic. Vaccines in conjunction with efforts around masking and social distancing have reduced SARS-CoV-2 infection rates, however, there are still significant challenges to contend with before the pandemic shifts to endemic, such as the coronavirus acquiring mutations that allow the virus to dodge the immunity acquired by hosts. SARS-CoV-2 variants deploy convergent evolutionary mechanisms to sharpen their ability to impede the host’s innate immune response. The continued emergence of variants and sub-variants poses a significant hurdle to reaching endemicity. This underscores the importance of continued public health measures to control SARS-CoV-2 transmission and the need to develop better second-generation vaccines and effective treatments that would tackle current and future variants. We hypothesize that the hosts’ immunity to the virus is also evolving, which is likely to abet the process of reaching endemicity.
Keywords
Covid-19, pandemic, RNA virus, mutation, vaccines, immunity, endemic diseasesIntroduction
As the fourth year of the pandemic approaches, SARS-CoV-2 infections continue to spread, though the levels are variable and erratic in different parts of the world. More and more individuals are developing milder symptoms following infection with the virus, which has reduced the rates of hospitalization and fatalities [1]. The US and most European countries did not see a significant surge last winter (2023) as was initially predicted. Moreover, global infection rates have remained relatively stable in the last five months [2]. This has raised hopes that the Covid-19 virus can soon become endemic. This paper discusses how the host’s immunity is evolving alongside the virus and how this may facilitate a stable co-existence with the Covid-19 virus.
The dynamic of the virus-host interaction is subject to constant evolution. Since SARS-CoV-2 is an RNA virus, the virus will mutate, and the emergence of new variants will occur as we have already seen over the last three years [3, 4]. Suppose the virus mutates into a variant or subvariant that is more contagious and can evade the host’s immunity; in that case, the transmission rate can increase, which may lead to higher hospitalization and death rates. Furthermore, increased transmission means that the virus is replicating more often, which increases the chances that this RNA virus will mutate. Of note, RNA viruses are characterized by their high mutation rate [5], which increases the risk that a new variant may emerge. While media coverage of Covid-19 has dwindled, the infection continues to significantly impact human health in parts of the world. For example, since the start of the pandemic in India, there have been over 45 million confirmed cases of Covid-19 with 533,295 deaths [2]. Moreover, this disease continues to do marked damage to at risk populations, such as those with weakened immunity or comorbidities [6], which means that the fight is still far from over.
Having considered the important advances in our knowledge regarding viral mutation and the host’s immune response to emerging variants, this paper addresses whether SARS-CoV-2 could be here to stay, despite the presumed evolution of a stronger immune response in hosts over time. In this paper, we discuss different components of immunity impacting the Covid-19 virus including innate immunity, T and B-cell mediated immunity, cross reactive immunity, the role of the host’s genetics, and then hypothesize that the hosts’ immunity to the virus is evolving, which is likely to support the process of reaching endemicity.
The emergence of SARS-CoV-2 variants
The continuous evolution of SARS-CoV-2 has resulted in the emergence of numerous subvariants that often exhibit growth advantages over previous variants. Scientists have recently reported that the most infectious SARS-CoV-2 variants exploit convergent evolutionary mechanisms to hone their ability to impede host immune responses [7]. They discovered that earlier variants (such as Alpa, Beta, Gamma, and Delta) converged to suppress interferon (IFN)-stimulated genes through several viral proteins, including open reading frame 6 (Orf6) and Orf9b, which serve as innate immune antagonist proteins [7]. A second study by the same group further elucidates the role of Orf6 in weakening important pathways in the hosts’ antiviral responses [8]. A recent study demonstrates that viral structural M proteins are abundant in Omicron variants and were shown to impact innate immune system pathways [9]. Additionally, the Orf proteins were shown to impact genes in hosts making an antagonistic effect on innate immune pathways [9]. Viral mutations that enable them to limit the host’s innate immunity mechanism may at least partially explain why the SARS-CoV-2 virus has continued to spread.
Since the emergence of Omicron in December 2021, several hundred sub-lineages (BA.1, BA.2, BA.4, BA.5, and BA.2.12.1) have evolved [10], some of which demonstrate the ability to evade immunity from vaccination or previous infections. BA.4 and BA.5 exhibit the qualities of immune evasion; studies indicate that the variants can elude some of the antibodies produced after coronavirus vaccinations and infections, including infections caused by some earlier versions of Omicron (Figure 1). The BA.5 sub-lineage can evade antibodies from both vaccination and prior infection [11].
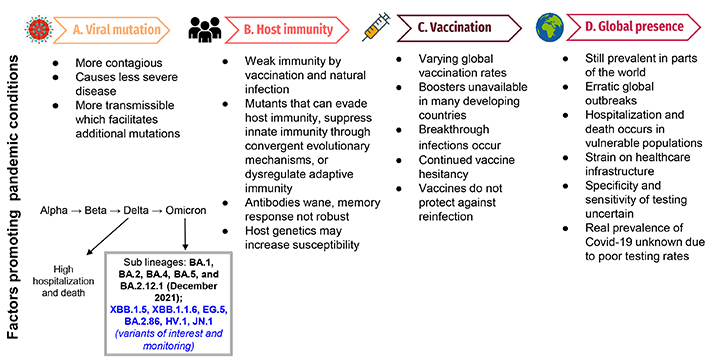
The changing landscape of the Covid-19 pandemic. This figure demonstrates the factors that will propagate a pandemic situation: Panel A lists viral mutations and characteristics that will promote a pandemic and demonstrates the characteristics of recent variants; panel B demonstrates factors affecting host immunity and variations in susceptibility that would propagate the current pandemic; panel C illustrates factors related to vaccination that would facilitate a continued pandemic state; panel D demonstrates factors contributing to a pattern of continued, erratic global outbreaks attributed to a pandemic
In September 2022, scientists identified subvariant XBB, which is believed to be a recombinant of Omicron subvariants BA.2.10.1 and BA.2.75 [12]. A new group of Omicron sub lineages (BQ.1, BQ.1.1, and XBB) was reported to be gaining ground across the US and other countries in late 2022 [12]. The BQ.1.1 variant has three spike mutations (N460K, K444T, and R346T), which makes it more contagious than other closely related variants. Both the BQ.1 and BQ.1.1 sub lineages are descended from BA.5, which had been dominant for months, while XBB comes from two different BA.2 lineages recombined into one [12].
The BQ.1.1 variant appears to have an advantage due to three major mutations on its spike protein that make this variant more contagious than its predecessors. The researchers found that BQ.1.1 can evade antibodies from past BA.5 infections, which suggests it may also be able to dodge protection from vaccines [13]. The study also found that some monoclonal antibody drugs are less effective against BQ.1.1, compared to earlier strains of the virus. This is particularly concerning since antibody therapies have proved popular and effective against other variants and subvariants of SARS-CoV-2. Public health officials fear that the ability for substantial neutralization escape can render antibody treatments like Evusheld, a Covid-19 treatment for severely immunocompromised individuals, and other monoclonal antibody treatments to be largely ineffective against these new variants. Of note, there is a long-standing controversy between the efficacy of therapeutic monoclonal antibodies and polyclonal preparations from convalescent plasma [14, 15].
In March of 2023, a new variant known as XBB.1.6 (also known as Arcturus) was classified by the WHO as a variant under monitoring [16]. This variant has been identified in at least 31 countries, including the US. It is very similar to its predecessor XBB.1.5 but has one additional mutation in the spike protein. The variant XBB.1.1.6 has a higher transmissibility rate than previous strains but doesn’t appear to be more dangerous, however, symptoms of conjunctivitis in young patients have been reported in association with this particular variant.
BA.2.86, a descendant of Omicron strain BA.2, emerged in August of 2023 [17]. With more than 30 mutations seen in its spike protein [18] this strain was considered to be more transmissible (Figure 1), however, BA.2.86 was not shown to be more dangerous than its predecessors [19].
On August 9, the WHO classified EG.5, also known as Eris as a “variant of interest”, indicating that Eris will be monitored for mutations that may make it more severe. Since then, EG.5, accounted for 24% of all cases while Fornax (FL.1.5.1) accounted for 12% of all cases in the week ending September 30, 2023, in the US [20]. A newer variant, called HV.1, surpassed Eris as of the week ending October 23, 2023. Of note, both Eris and HV.1 are descendants of the XBB variant [21, 22].
The latest variant to attract the attention of scientists and public health agencies is called JN.1, and so far, it appears to be highly transmissible (Figure 1). The rapid ascent of the JN.1 variant in December 2023 has led the WHO to also label this as a “variant of interest”. According to the WHO, JN.1 is a “descendent lineage” from BA.2.86, which itself is a descendant of the BA.2 Omicron subvariant. The difference between BA.2.86 and JN.1 is that the latter possesses one additional spike mutation, L455S that has resulted in increased receptor binding to angiotensin-converting enzyme 2 (ACE2). The preliminary research indicates that this single mutation may provide extra immune evasion capacity to this strain [23]. Further studies are needed to better understand the rapid accumulation of immune-evasive mutations that have been discovered in recently emerged variants. It is yet not clear what potential JN.1 has to cause breakthrough infections and whether these infections result in severe disease. Infections with JN.1 have also been reported in several European countries including France, the UK, India, Singapore, and China [24]. The rapid and continued growth of this variant suggests that it is better at immune evasion and more transmissible than its predecessors. In the US, JN.1 was first detected in September 2023 and now accounts for 44% of cases in the week ending December 23, 2023, up from just 7.5% the month prior [25].
Interestingly, the impacts of sub variants may vary from place to place which can complicate global monitoring. For example, BA.2.75 caused a major spike in illness in India, while barely registering in other countries [26]. This could be related to limited testing and reporting. It is not clearly understood how the host’s immune system adapts to the mixture of subvariants cocirculating at the same time in the hosts and how these processes are impacted by the varying types and rates of vaccination by country.
How host immunity is evolving with SARS-CoV-2
As we enter the fourth year of the Covid-19 pandemic, the immunity landscape is as complicated as ever due to the variability in infection rates, exposure to different viral variants, and disparate vaccination rates. The general trend seems to be that infections are getting milder as immune systems adapt to protect against serious disease, despite the continuous mutation across the SARS-CoV-2 genome [27]. The CDC estimates that approximately 97% of the US population has antibodies to SARS-CoV-2 from vaccination, prior infection, or both [28]. The fact that the clinical presentation of emerging variants continues to be mostly mild, demonstrates the dynamic nature of the hosts’ immune system.
It seems that Covid-19 has become a more manageable disease as “community immunity” builds up from vaccination and prior infection, in conjunction with the availability of Covid-19 tests to detect circulating variants and antiviral treatments against emerging variants has led to the development of milder infections. Still, we cannot say how long the Omicron phase of SARS-CoV-2 evolution will persist. However, if a subvariant continues to spread and replicate, evolutionary pressure will ultimately cause the lineage to change further. The chance for the emergence of a new dominant variant, which causes more severe disease or has developed immune evasion mechanisms, is still there, so continued monitoring is of paramount importance. Covid-19 still causes hospitalization and deaths in the US and elsewhere, though it is seen more so in elderly and immunocompromised populations [29]. Recent reports from the CDC show roughly 1,300 deaths per week compared to nearly 4,000 deaths per day from Covid-19 in 2021 [25].
Moreover, the currently available vaccines do not protect against reinfection, and the immunity induced by vaccinations or natural infection tends to fade within eight months [30]. While the current vaccines may be sufficient to provide individual protection against severe disease, they have little effect on protection against infection and reduction in transmission and therefore do not adequately generate community immunity (Figure 1) [31]. Without a reduction in transmission, the risk for a more contagious subvariant to emerge remains; the more the virus spreads and replicates, the more chances for a mutation to occur [32]. The issue of “herd immunity” is often raised in Covid-19 research. We estimate that the goal of reaching “herd immunity,” remains elusive as the virus develops immune evasive characteristics and adapts to undermine the vaccines’ protection [33, 34]. SARS-CoV-2 immune evasion strategies include mutating key protein structures that are recognized by the body’s immune system or, camouflaging its viral mRNA to mimic the host’s mRNA to avoid immune detection. A recent study has shown that the SARS-CoV-2 RNA’s genome contains a code for a viral five prime (5’) cap, which facilitates immune evasion [35].
Not only have SARS-CoV-2 acquired mutations to evade the hosts’ immunity, but also those that inhibit the innate immune response [36]. Innate immunity is the first to respond to intruding pathogens and may exert a strong selection pressure on the Covid-19 virus, which could explain the rapid spread of the two most recent and dominant variants. One of the ways innate immunity protects the host against infection is to recruit antiviral proteins to combat invading viruses; studies have shown that SARS-CoV-2 can dampen the activation of these critical antiviral proteins [36]. This allows SARS-CoV-2 to evade the body’s initial lines of defense and could explain the virus’ ability to infect vaccinated or previously infected people.
Several studies have shown that neutralizing antibodies can wane over time, however, the long-lasting B and T memory cells can persist in recovered individuals [37, 38]. The initial spike in immunoglobulin A (IgA) antibodies drops significantly and the levels of IgG antibodies remain elevated for at least 3 months [39]. In a recent study, IgG antibodies against spike proteins were found to be stably produced for over 6 months post-infection. SARS-CoV-2 CD4+ T cells and CD8+ T cells showed a half-life of 3–5 months in primary response [37, 39]. However, in the secondary response, T-cell responses can persist in the blood long after the antibody responses wane [40]. One study demonstrated that T-cell responses were detected up to 15 months after initial Covid-19 infection with 86% sensitivity [41, 42]. New research conducted in mice demonstrates that memory CD8+ T cells are crucial in mediating SARS-CoV-2 viral control in the lungs, independently of antibodies [43]. The memory T cell response has better breadth and magnitude (Figure 2) [44]. Recent studies report that memory T cells targeted the virus in the respiratory tract, such as the airways, lung vasculature, and associated lymph nodes [43].
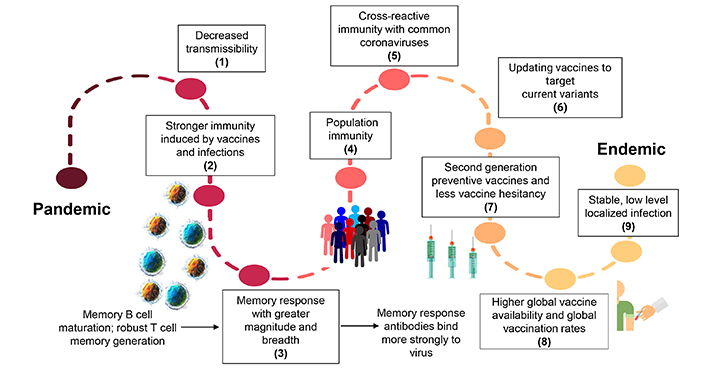
The journey of Covid-19, from pandemic to endemic. This figure demonstrates the factors that will eventually shift the current pandemic to an endemic situation: (1) decreased transmissibility of circulating virus, (2) increased immunity induced by vaccines and infections, (3) induction of a stronger memory immune response by stimulating memory B cell maturation and robust memory T cell generation, (4) more people in the community acquiring an immune response, (5) stronger cross-reactive immunity stimulated by the common coronavirus, (6) updating vaccines targeting the currently circulating virus, (7) the development of better second-generation vaccines preventing infection and wide vaccine acceptance, (8) increased global vaccine availability leading to local control and to impeding the spread of SARS-CoV-2 virus, (9) shifting to low stable localized infections facilitating the transition to endemicity
A recent study compared the antibody mediated response between patients who had severe or mild disease and found that patients with severe disease demonstrated a more robust humoral immune response due to increased levels of B cell receptor activation and clonal expansion [45]. Interestingly, another study using inhibition assay found contrasting results which indicated that convalescent Covid-19 patients did not always generate spike specific and receptor binding domain (RBD) specific antibodies that inhibit RBD binding to ACE2 [46]. Clearly, SARS-CoV-2 infections can generate varying levels of antibody response [46]; however, it is not robust enough to induce protection against reinfection, which has implications for studies seeking to measure vaccine-induced immune memory (Figure 2).
Stefano and Kream [47] proposed that convalescent memory T cell immunity in individuals with mild or asymptomatic SARS-CoV-2 infection may result from an evolutionarily adapted immune response to coronavirus and the ‘common cold’. Furthermore, there is increasing evidence of the existence of SARS-CoV-2-reactive T memory cells in unexposed healthy individuals originating from previous immune responses to endemic coronaviruses that cause the ‘common cold’ in humans [48]. The patterns of SARS-CoV-2-specific CD4+ and CD8+ T cells responsive to human leukocyte antigens (HLA) class I and class II epitopes found in multiple species of viral proteins were observed in a significant number of unexposed healthy donors [48, 49]. These findings suggest a more extensive and persistent expression of SARS-CoV-2-reactive T memory cells in unexposed healthy individuals than previously believed, which could be attributed to multiple cross-reactive viral protein targets following previous exposure to circulating human endemic ‘common cold’ coronaviruses (Figure 2) [49, 50].
Assessment of global virus-specific T cell responses could be challenging. Compared to humoral immunity, the investigation of cellular responses against peptides presented through multiple HLA alleles in human populations is more complex [51]. However, some important progress has recently been made. A recent study has shown that spike mutations can lead to loss of T cell recognition within epitopes restricted by common HLA alleles [52]. This is potentially important, given that population immunity may not yet be sufficient to drive strong T cell selection [53]. The large number of mutations in the Omicron variant within the spike is likely to inactivate the presentation or recognition of some epitopes. At the current time, potential mechanisms by which viral proteins or RNA may act to directly suppress antigen presentation are unclear, although ORF8 was shown to downregulate the expression of HLA class I proteins [54]. Of note, ORF8 is a rapidly evolving accessory protein of SARS-CoV-2 that has been proposed to interfere with immune responses to the virus [55].
Interestingly, recent data showed the presence of non-spike cross-reactive memory T cells protecting SARS-CoV-2-naïve contacts from infection [56]; these findings suggest that vaccine manufacturers should include non-spike antigens that can target spike-antibody immune escaping variants when designing better second-generation vaccines (Figure 2). In fact, recent scientific endeavors have been focused on the development of vaccines that could stimulate long-lasting T-cell responses and researchers have started comparing the T-cell responses between the currently available vaccines. It has been reported that the mRNA vaccines from Pfizer and Moderna (as well as two other vaccines that work by different mechanisms) produced relatively consistent levels of a key T cell in the six months after vaccination [57]. Over the same period, antibodies generated by the Pfizer and Moderna shots faded. Researchers at the University of Tübingen in Germany have a trial going to investigate the safety of a vaccine made of SARS-CoV-2 proteins that are known to stimulate T-cell immunity [58]. A similar study at the Massachusetts Institute of Technology demonstrated that their mRNA vaccine, which targets highly conserved parts of the coronavirus, generates a robust T cell response that confers protection against morbidity and mortality in mice [59].
Recently, Hendrix et al. [60] investigated if prior infections with SARS-CoV-2 impact the severity of subsequent infections. The authors found a mild protective effect during the early stages of the pandemic, however, this faded after the emergence of the Omicron variants. The study concluded that a prior infection is not enough to prevent harm from subsequent infections. It is important to note that there is differing immunity to different variants as each variant has a different number of mutations or combinations thereof and those mutations could occur in varying locations in the virus. These findings emphasize the need to continue to bolster prevention strategies through vaccination (i.e., developing more efficacious vaccines) and appropriate public health measures, rather than relying on immunity acquired through previous infection.
While most antibodies showed minimal neutralizing activity against earlier variants, one antibody called S309 shows promise as it targets a region on the virus’ spike protein that has remained relatively unchanged between variants, presumably due to its essential role in cell entry [61]. The authors of this study suggest that due to immune imprinting, the immune system tends to produce antibodies specific to older variants, rather than produce new antibodies specific to newer variants [61]. The authors suggest that updated vaccines should not contain components from previous variants as this may induce a less effective immune response and affirm our earlier assertion that updated vaccines should target conserved structures across SARS-CoV-2 variants [4].
Host genetics and susceptibility to SARS-CoV-2
It has recently been shown that people with allergic conditions such as hay fever, rhinitis, and atopic eczema, may have a lower risk of Covid-19 infection, especially if they also have asthma [62]. This may be related to the decreased expression of ACE2, the gene encoding the SARS-CoV-2 receptor, which has been reported in people with asthma [62]. SARS-CoV-2, and human coronavirus NL63 (HCoV-NL63), utilize the human protein ACE2 as a cellular receptor to gain entry into human cells [63, 64]. Genetic ACE2 variants are likely to correlate with the increase or decrease in their affinity. However, it is unknown whether these natural ACE2 variants decrease or increase their affinity for coronavirus spike protein and affect the susceptibility of individuals to infection. Several variations have been observed in the ACE2 gene, some of which have been significantly associated with arterial hypertension, diabetes mellitus, and coronary artery disease [65]. A recent study that assessed the impact of ACE2 single nucleotide polymorphisms (SNPs) on interactions with coronavirus S proteins and SARS-CoV-2 entry in vitro and in vivo has identified an SNP that potentially protects individuals against SARS-CoV-2 infection [66]. ACE2 polymorphism may alter human susceptibility to SARS-CoV-2 infection and contribute to ethnic and geographical differences in SARS-CoV-2 spread. Intact viruses can target their receptors on multiple cell types through conformational matching; this is followed by viral replication by an evolutionary modification of the ACE2 receptor required for the virus binding and host cell entry [67]. The ability to successfully escape immunity while maintaining its ability to bind to ACE2 may have potentially contributed to the rapid global spread of Omicron subvariant BA.5 [68].
Although both genetic and environmental factors are certainly expected to impact the susceptibility of individuals, the HLA alleles can affect both the susceptibility and the severity of SARS-CoV-2 infection. The crucial role played by HLA molecules in the immune response, especially through pathogen-derived peptide presentation, and the huge molecular variability of HLA alleles in the human populations could be responsible for the varying rates of infection in different patients following Covid-19 infection [69].
A recent study conducted an enrichment analysis leveraging the Reactome database and suggested that the immune system is a major area involved in Covid-19-related genetic variation [70]. This includes the influence of signaling by interleukins (ILs) and IFN signaling domains, implicating protein-protein interactions [70]. While common variants’ risk profiles can help predict the severity of the disease, they do not significantly improve the prediction of disease severity when compared to demographic/clinical factors alone [71, 72]. Clearly, there is a need to establish a higher number of genetic predisposing factors to better predict disease severity.
A recent genome-wide association study reported genetic risk factors, such as six genes in a region of chromosome three, are associated with Covid-19 severity [73]. Another study found that patients with severe Covid-19 disease had genetic mutations that resulted in a deficient type I IFN response or autoimmune antibodies affecting type I IFN [74]. In fact, recent studies highlighted that many of the genes associated with severe disease were associated with inflammation or immunoregulatory pathways [75], particularly those associated with the IFN signaling pathway [76]. These studies support the idea that human genetic variation can impact the susceptibility and pathogenicity of the Covid-19 virus in different geographic territories and ethnicities. Of note, most genome wide association studies conducted early on were skewed due to small sample sizes and the selection of severely ill patients. Clearly, further studies are required to better identify the genetic factors that influence Covid-19 disease pathology to define biomarkers for at-risk individuals and inform potential therapeutic targets.
Vaccines and the role of boosters
In most infectious diseases, with a few exceptions, the human immune system needs repeated exposure to natural infections to develop immunity, even in the case where the infectious agent does not mutate significantly. Notable exceptions include measles and cutaneous leishmaniasis in which one primary natural infection may lead to life-long immunity [77, 78]. Many individuals in Africa can develop a degree of natural immunity against malarial parasites after repeated exposure [79]. Immunity without repeated exposure wanes and exposure with smaller doses reduces the risk of overburdening the immune system. Ideally, the purpose of the boosters is to provide this repeated exposure to trigger the immune system’s memory cells to produce a response of protective antibodies and T cells that can target a wide range of related viruses.
Currently, scientists are debating how frequently bivalent vaccines need to be used across the population. A new study from Yale University and the University of North Carolina at Charlotte suggests healthy people should get annual Covid-19 boosters to prevent widespread outbreaks [80]. On the other hand, a recent paper in the New England Journal of Medicine challenges the use of mRNA boosters in healthy individuals who often develop mild infections. The author opined that protecting against Covid-19 infection with the current mRNA technology is unrealistic, especially as new coronavirus strains emerge every few months [81]. Of note, the bivalent booster was hurriedly introduced with limited data from pre-clinical animal studies. Furthermore, relatively few people in the US have had updated boosters. As of February 6, 2023, only around 15% of people over age 5 had gotten them, according to the CDC [25].
Recently, there has also been an increased interest in the development of intranasal (IN) vaccinations for Covid-19. Recent studies have demonstrated that intramuscular vaccines are not effective at hampering viral replication and shedding in the upper respiratory tract, so while symptoms are milder, the virus can still be transmitted [82]. The appeal for IN vaccinations lies in the fact that they have shown promise to induce sterilizing immunity against mucosal pathogens [83], which could prevent virus infection, replication, shedding, and disease development, while also limiting viral transmission. Most recently, researchers have developed an IN immunization that is being tested in animal models and which has demonstrated promising results by significantly reducing viral replication in the nasal mucosa, lungs, and brains in mice and significantly reducing viral airborne transmission in hamsters [84]. This shows much promise over intramuscular routes of administration as it is less invasive and stops the viral replication in the airways before the virus enters the bloodstream.
Vaccines-induced immune responses naturally wane over time. This in conjunction with the immune evasiveness of newly emerged variants, might explain why those particular subvariants were able to spread quickly regardless of vaccination status or prior infection [85]. These findings have lent urgency to policymakers to develop Omicron subvariant-targeted boosters (Figure 1). In 2022, the CDC recommended using bivalent mRNA vaccines as boosters for human beings [86]. This vaccine targets both the original strain of SARS-CoV-2 and Omicron subvariants BA.4 and BA.5. The effect of this booster vaccine has been tested in mice and was found to generate a significant immune response against the new Omicron subvariant. Newly released data from Pfizer and BioNTech’s ongoing clinical trial indicate that the booster stimulated an immune response against Omicron’s BA.4 and BA.5 subvariants; participants demonstrated elevated antibody levels against BA.4 and BA.5 just seven days after injection [87]. Although this booster is a bivalent vaccine made from the BA.4 and BA.5 Omicron subvariants, it is expected to provide broad protection against newer variants as well. It is not yet clear if this new booster will stimulate protection against reinfection for a longer period, however, the most important point here is how well the vaccines can prevent severe disease with the currently circulating variants. The most recent (May 2023) recommendation from the WHO suggests that vaccine makers should drop ancestral strains and switch to a monovalent vaccine that only targets current variants [88].
In addition, about 3% of the US population (7 million people) are severely immunocompromised, according to the National Institutes of Health [89]. Until recently, this cohort was prescribed Evusheld, however recent data suggest that the most dominant variants have evolved so much that Evusheld is unlikely to provide protection and therefore is no longer authorized for use by the FDA [90]. Since this announcement in January of 2023, individuals with compromised immune systems have been limited in options to protect themselves from infection or severe disease. The US government announced this week that it is funding the development of a new preventive antibody through the drug company Regeneron. Trials of that drug are expected to start this fall, according to the US Department of Health and Human Services.
Another recent study suggests that instituting yearly, population-wide booster vaccinations updated to predominant variants is beneficial, however, the study only focused on people with healthy immune systems [80]. A recent study suggests instituting regular, population-wide boosters, which target the currently circulating variants, could be an important strategy to forestall or eliminate Covid-19 [80]. However, this study did not consider data from immunocompromised populations. The authors of this study acknowledged that immunocompromised populations may require more frequent immune boosting and are conducting additional studies to evaluate the optimal vaccine interval for people with weakened immunity. It is not clear when individuals with weakened immune systems should be given the bivalent booster and how prolonged and robust the antibody response is generated. Many immunocompromised individuals have a lower number of B-cells [91], which play an essential role in antibody and cytokine production to protect against infection. Further studies are needed regarding the dose, frequency, and type of vaccine (e.g., mRNA, protein-based, or inactivated virus) in individuals with different demographics and health conditions.
When developing vaccine and booster schedules, public health leaders must consider the risk of immune exhaustion, which is characterized by the inability of immune cells to function properly due to repeated stimulation. Of note, a recent study demonstrated that T-cell responses to various vaccines remain stable without showing any signs of T cell levels declining over time, and most notably, that boosters do not significantly affect the T cell responses [92]. If this is the case, then Covid-19 immunity could be maintained with only occasional boosting based on T cell levels. However, quantitatively measuring T cells is more difficult than antibody detection. There is a need to improve this technology and relate T cell monitoring to the development of future vaccines, boosters, and effective immunization schedules.
A recently published systematic review concluded that individuals who were vaccinated and infected (hybrid immunity) had the highest magnitude and durability of protection, and as a result, might be able to extend the period before booster vaccinations are needed [93]. Another study reported that T and B cells responses are enhanced in vaccinated individuals. It was shown that activated memory T cells appeared early and could be an important component of the early immune response to breakthrough infections to prevent severe disease [94]. Assessing the magnitude and durability of existing protection has become a challenge because of the varying rates and timings of past infections and vaccinations, availability of multiple types of vaccinations and varied dosing schedules, and variants of concern that can escape pre-existing immunity. These variables make it difficult to establish a standardized booster schedule as we have seen with other vaccinations.
According to the CDC, while 69 percent of Americans completed their initial two-dose vaccination series, only 17 percent are up to date with last year’s booster dose [25]. Most recent data indicate that only 7% of adults and 2% of children have received the updated 2023–2024 vaccine, though it was rolled out on September 14, 2023 [95]. Furthermore, Covid-19 is now one of the three leading causes of death among persons aged 65 and older [96], however, only 40% of that group has received updated booster shots [25]. Public health officials attribute the low uptake in boosters to the lower hospitalization and death rates as reported on social media. Yet another reason for the low booster rate could be a lack of trust in the shots, which has posed a significant obstacle since the introduction of these novel vaccines [4]. Stronger public health messaging by physicians, officials, and advocates that pointedly address the various factors contributing to vaccine hesitancy would be beneficial (Figure 1).
The original mRNA vaccines developed by Pfizer and Moderna targeted the original SARS-CoV-2 virus. In September of 2022, the vaccines were updated to be bivalent and targeted the original strain as well as Omicron variants BA.4 and BA.5. The most recent vaccine updated in September 2023 has replaced the bivalent version and targets the XBB lineage of the Omicron variant. Vaccine manufacturers have said that the most recently approved vaccinations will still work against BA.2.86, along with EG.5, FL.1.5.1, based on preliminary data from preclinical trials [97]. Since HV.1 is also a descendant of XBB [22], officials believe that the updated booster will also confer some protection against this most recent strain. Like its predecessors, the updated vaccines will likely be effective at dampening disease severity and preventing hospitalization and death from Covid-19. It remains to be seen how long the immunity induced by these updated vaccines will last and how effective they will be against primary infection or reinfection. Anyone above the age of 6 months is eligible to get vaccinated, however, individuals that recently had Covid-19 are advised to delay vaccination by three months. It is important to note that all of these mRNA vaccines must be stored at ultra cold temperatures, which makes global vaccine distribution a challenge in rural areas without the infrastructure to support proper storage. Furthermore, the cost prohibitive nature of these vaccines can limit access and distribution in developing countries unless they are substantially subsidized.
On October 3, 2023, the FDA issued an emergency use authorization for the Novavax vaccine, which is the fourth Covid-19 vaccine to be administered in the US (after Johnson & Johnson, which is no longer available). Like the Pfizer and Moderna vaccines, the Novavax vaccine targets the XBB.1.5 strain. This vaccine contains a recombinant form of the SARS-CoV-2 spike protein produced from baculovirus infected Sf9 insect cells and Matrix-MTM adjuvant containing saponins [98]. The duration of protection against Covid-19 is currently unknown, however, the Novavax Covid-19 vaccine, demonstrated 90% efficacy in its clinical trial, performing almost as well as the other mRNA vaccines in their early trials [99]. Of note, this particular vaccine is simpler to make and can be stored in a refrigerator which makes it easier to distribute globally.
The efficacy of a vaccine booster to contain SARS-CoV-2 will depend upon whether the virus mutates again and whether antibodies generated against earlier strains will still be effective. Vaccines that induce antibodies against current and future SARS-CoV-2 variants in conjunction with therapeutics, including monoclonal antibodies and antiviral drugs, will be critical in the fight against Covid-19. Another challenge is that updating vaccines requires clinical testing; however, finding adults who have not been infected or vaccinated remains a challenge for conducting valid tests.
Experts do not expect the recent vaccination of children ages 6 months to 5 years old to alter the trajectory of the pandemic due to sustained vaccine hesitancy in parents; seven out of ten parents in the US remain hesitant about vaccinating their children mainly due to the lack of transparency in the trial process and dosing determination [100, 101]. Furthermore, many children may not be eligible for updated boosters as many of them have not yet received a primary dose of vaccine. Interestingly, studies report that infants and young children infected with SARS-CoV-2 demonstrate a stronger and longer lasting antibody response when compared to adults [102]. Remarkably, the children’s noses had high levels of inflammatory cytokines, IFN-α, Th17, and neutrophil markers IL-17, IL-8, and CXCL1 in nasal samples but not in the blood. This finding raises the possibility of devising vaccine adjuvants that mirror young children’s immune responses without stimulating potentially dangerous excess inflammation in the blood. Of note, vaccines developed for adults typically require in-depth research to calculate adequate dosages and investigate the short-term and long-term side effects before they are approved for use in children, which adds to the challenge of developing vaccines for this group. Transparency and adequate messaging by physicians and public health workers targeted at parents might be helpful in the application of mass-scale vaccination, particularly with vaccines developed using new technologies (Figure 1).
It was reported that most children develop SARS-CoV-2 infection with a milder clinical course than adults [103]. In children, the higher number of naive and regulatory cells may help in avoiding the cytokine storm often associated with severe Covid-19 disease. Furthermore, recent studies report a considerable increase in the prevalence of respiratory viruses from 2022 to 2023 within the pediatric population [104], which suggests a significant change in viral activity. Respiratory syncytial virus (RSV) saw the greatest surge, which can be attributed to the lack of immune stimulation during the high pandemic period. Clearly, preventative efforts to contain SARS-CoV-2 were successful in decreasing the transmission of respiratory infections.
The shifts in viral prevalence and infection patterns highlight the dynamic nature of viral circulation and the evolution of hosts’ immunity. Continued monitoring the consequences of these viral interactions, such as co-infections through the use of tools such as prospective epidemiological studies and mathematical modeling have been suggested [105].
SARS-CoV-2 may eventually spread like endemic coronaviruses, where exposure to young children under the age of 5 can help bolster the immune system to prevent serious disease. Reinfection throughout adulthood is common for many viruses that were initially encountered in childhood. In the context of SARS-CoV-2 variants, this has raised hopes that repeated exposure throughout a person’s lifetime can strengthen immune responses to new variants and accelerate the drifting of the virus toward lower virulency.
The prospect of Covid-19 shifting to endemic
The transition from pandemic to endemic could take time and depends on how long humans retain immunity to the Covid-19 virus and how quickly the virus evolves (Figure 2). Generation of robust host immunity to recent variants may accelerate the transition to endemic, which could lead to the SARS-CoV-2 virus becoming like other commonly circulating coronaviruses. However, we do not know yet what degree of immunity is enough to contain Covid-19 nor to reduce transmission rates (Figure 2). The dynamic nature of the host immune system is evolving and impacting the outcomes of the host-viral interaction. Despite continuous mutations across the SARS-CoV-2 genome as well as in their spike protein, the clinical presentation by emerging variants is mostly mild. In short, Covid-19 has changed and so has our immunity.
We may continue to see disparate outcomes in severe disease and deaths between vaccinated and unvaccinated people for some period in different parts of the world. Development of “herd immunity” in enough people in a population who become immune to the virus could be helpful in the transition to endemicity. However, achieving herd immunity to the Covid-19 virus seems to be elusive because of the variable global vaccination rate and the ever-changing nature of SARS-CoV-2 in terms of infectivity [106, 107]. The frequent surge of SARS-CoV-2 is a further sign that a herd immunity threshold against Covid-19 has not yet been reached.
As long as viral transmission remains widespread, the virus will continue to mutate, and more variants and subvariants will emerge (Figure 2). Natural selection will favor mutations that give the virus the greatest survival advantage, so the selection of mutations that promote the evasion of the host’s immunity is not surprising [108] and could delay the transition to an endemic state. Additionally, some of the new variants could be so different that the immunity induced by the currently available vaccines will fail to recognize them, and the virus will continue to replicate and transmit effectively in vaccinated and/or nonvaccinated individuals. The development of better second-generation vaccines capable of stimulating a robust immune response involving a full arsenal of both cellular and humoral immunity, which could be more effective in containing existing and future SARS-CoV-2 variants, is critical. Many scientists have underlined the importance of developing universal Covid vaccines [109]. An effective universal vaccine could activate all the components of the immune system to work together to induce a high level of long-term immunity against SARS-CoV-2.
The average case is getting much milder over time, however, endemic doesn’t mean harmless. Tuberculosis and HIV, which are two well-known endemic diseases, cause millions of deaths each year around the world. Another endemic disease, influenza is an issue that public health workers must contend with seasonally. In most viruses, the use of treatments and vaccines causes them to evolve ways of escaping these protective measures so they can continue to spread [108, 110]. It is not yet known what selective pressures exist for viruses to acquire the genetic material that facilitates the quick emergence of new variants. One theory is that while these viruses can hide from the immune system, they will survive longer to replicate, and may pick up new genetic material in replication. The selective pressures on the genome of a pandemic virus are likely to be variable in different parts of the world.
Conclusions
The transition for the Covid-19 virus to become endemic is still not certain at this stage, and it is hard to predict the timeline as Covid-19 has changed alongside our immunity. Prospects of reaching endemicity are promising, as the majority of people’s immune systems can recognize key parts of the virus now, we have updated vaccines and good antivirals to lean on when cases start to rise, masks still work, rapid tests are in stores, we now know to filter the air and to ventilate our spaces. Yet there are still reasons not to treat the virus too casually just yet as there are still many unknowns, even four years in. The Omicron variants and subvariants are still driving infections in the US and elsewhere. Currently, poor testing and low reporting may mean that Covid-19 levels are likely underestimated in many parts of the world. In addition, many places are no longer regularly sequencing the virus genome to determine the circulating variant, which also hinders our understanding of the trajectory of Covid-19 globally.
Our best hope will be to increase the number of people vaccinated with better second-generation vaccines, which match the currently circulating virus, and simultaneously reduce the transmission rates. In the meantime, efforts should continue to develop more effective vaccines that reduce the reinfection level and treatments that can cure infected individuals, particularly for vulnerable populations. There are signs of optimism, and we speculate that SARS-CoV-2 will soon follow in a similar evolutionary trajectory to the four endemic coronaviruses that cause the ‘common cold’. Eradication will be a different campaign, which requires the development of more effective vaccines that can protect against reinfection at a local and global level.
Abbreviations
ACE2: | angiotensin-converting enzyme 2 |
HLA: | human leukocyte antigens |
IFN: | interferon |
IgA: | immunoglobulin A |
ILs: | interleukins |
IN: | intranasal |
Orf6: | open reading frame 6 |
Declarations
Author contributions
AH: Conceptualization, Writing—original draft, Writing—review & editing. ABP: Writing—review & editing, Visualization. Both authors concur with the submission of the manuscript.
Conflicts of interest
The authors have no conflicts of interests to declare.
Ethical approval
Not applicable.
Consent to participate
Not applicable.
Consent to publication
Not applicable.
Availability of data and materials
Not applicable.
Funding
Not applicable.
Copyright
© The Author(s) 2024.