Abstract
Immunity is usually classified into two categories: innate immunity and adaptive immunity, distinguished by the process and characteristics of the immunological impact. It was widely assumed that only adaptive immunity possessed memory features; however, current research has revealed that innate immunity, like adaptive immunity, possesses memory properties as well. “Trained immunity”, also known as “innate immune memory”, is a phenomenon that occurs when the immune system’s innate cells are stimulated and then undergo epigenetic reprogramming and metabolic alterations. When it comes to innate immunity, macrophages are essential since they have immunological memory capabilities and play a significant role in the body’s immunity. The concept of innate immune memory expands the definition of immunological memory and offers a broader view of immune response research. This article reviews the properties, mechanism, and significance of macrophage innate immune memory in disease.
Keywords
Macrophage, innate immune memory, epigenetic reprogramming, trained immunityIntroduction
There are two types of host immunity: innate and adaptive, mediated by monocytes, macrophages, neutrophils, other phagocytes, and natural killer (NK) cells and have the characteristics of rapid response and non-specificity. The latter is mediated by lymphocytes and has the characteristics of slow reaction, strong specificity, and classical immune memory. Recent research shows that immunological memory exists in both adaptive and innate immunity [1]. For example, plants and vertebrates without adaptive immunity can resist re-infection in the event of secondary infection; even if some different pathogen causes the two infections, cross-protection remains [1]. It is worth noting that there is a similar phenomenon in mammals [2, 3]; that is, innate immune cells can show some characteristics of adaptive immune response after receiving a specific stimulus and make an enhanced or weakened response to the secondary stimulus, and this response is not specific. This phenomenon is known as “trained immunity” or “innate immune memory (IIM)” [2, 4]. Since Netea et al. [2] proposed a new concept of “trained immunity” or “IIM” in 2011, more and more studies have confirmed the existence of IIM. Different natures of primary stimulation affect the reactivity of the cell’s secondary stimulation response [5]. Training immunity in the broad sense refers to IIM, including tolerance and training phenotype. Additionally, macrophages possess immunological memory functions and are involved in a wide range of key pharmacological and pathological processes like conventional innate immune cells. Research on innate macrophage immunity and its function in disease is the subject of this review.
Characteristic of IIM
The introduction of IIM is a great compliment and perfection to immunology, although both IIM and classical immune memory belong to immune memory have definite differences. Firstly, the cells involved in two different types of memory, myeloid cells, NK cells, and innate lymphoid cells (ILC), are IIM. Secondly, IIM is usually non-specific for reactivity to secondary stimuli. Classical immune memory relies on antigen-specific gene rearrangement. IIM relies on transcription factors and superficial genetic reprogramming signals [2], and the continuous changes of these transcription programs do not involve permanent genetic changes such as mutations and recombination. The duration of immune memory varies, such as IIM relies on changes in the functional status of innate immune cells that persist for weeks to months after the elimination of the initial stimulus, but usually not for years (Table 1).
Inherent immune memory and the characteristics of the adaptive immune memory
Characteristics | IIM | Adaptive immune memory |
---|---|---|
Organism | Plants, invertebrates, vertebrates | Higher vertebrates |
Cells | Monocytes/macrophages, NK cells, granulocytes, ILC | T and B lymphocytes |
Mechanism | Epigenetic reprogramming, cell metabolic change | Antigen-specific gene rearrangement |
Lasting time | Weeks to months | Years |
Specificity | No | Yes |
Discovery of IIM in macrophages
Macrophage IIM also includes two forms of tolerance and training. In 1988, scholars found that macrophages were closely related to endotoxin tolerance. After the first high-dose lipopolysaccharide (LPS) stimulation, macrophages would show low reactivity, called tolerance, to avoid excessive inflammation when the second stimulation occurs [6]. In 1986, Bistoni et al. [7] observed that in mice lacking T and B cells, Candida albicans infection could avoid the secondary infection of other pathogenic bacteria, and macrophages played a role in speculating that macrophages might have memory function. After Netea et al. [2] first proposed the concept of IIM in 2011, the macrophage’s training has been further recognized. In 2012, researchers identified β-glucan of Candida albicans cell wall as the main component inducing the training of macrophages mentioned above, and macrophages trained by β-glucan significantly enhanced their response to secondary infection of Candida albicans and other pathogenic bacteria [8, 9]. With the deepening of research, scholars found that micro bacteria, fungi, parasites, and viruses can train monocytes and macrophages to secrete more pro-inflammatory cytokines and enhance their phagocytosis during secondary stimulation, confirming the uniqueness of the IIM of macrophages [9–12]. In addition to animal models, IIM has also been found in humans. For example, injection of Bacillus Calmette-Guerin (BCG) can induce the production of IIM, and this process is related to the activation of histone tri-methylation of lysine 4 on histone H3 (H3K4me3) [13]. Moreover, there is evidence that macrophages’ IIM plays a vital role in diseases. For example, inflammatory stimulation can cause the epigenetic reprogramming of brain microglia, aggravate the degeneration of beta-amyloid, enhance the inflammatory response of the central system, and play a pathological role in Alzheimer’s disease [14].
It is commonly known that macrophages, as their title indicates, may phagocytize pathogens and antibodies. They do, however, have sentinel and innate immune cell-calling functions, which allow them to summon and drive adaptive and innate immune cells to the damage site (Figure 1a). Some of the strategies employed by macrophages as effectors to restrict the progression of intracellular infections include antimicrobial peptides and cell death triggered by macrophage activation. Macrophages and sentinels, which create and present antigens to adaptive immune cells, begin and coordinate immune activation.
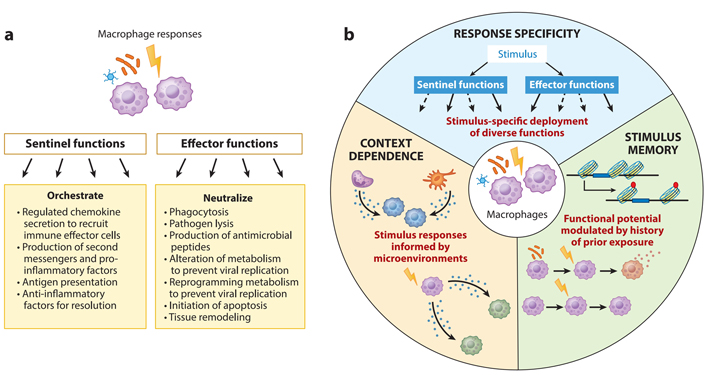
Functional characteristics of macrophages. (A) As a response, macrophages engage in a variety of functions. They might act as immune system guards or immunological effector cells, depending on their function; (B) macrophage responses have three characteristics of immunological activity: response specificity, context reliance, and stimulus memory. Solid and dashed arrows show the deployment of various actions to vary degrees of speed
As first responders, macrophages are strategically placed throughout the body. Nearly every organ and tissue in the body have a remarkable density of 5,000–10,000 macrophages per cubic milliliter [5–7]. Macrophages in the peritoneum and liver Kupffer cells detect infections in the air or the digestive system, and macrophages in the peritoneum detect infections in the air or the digestive tract. When bone marrow-derived monocytes identify chemoattractants generated by the first responding macrophages, they flow into the bloodstream and rapidly extravagate into tissues. Monocytes rapidly develop into macrophages after recognizing a damage-associated molecular pattern (DAMP), a pathogen-associated molecular pattern (PAMP), or an infected cell coated with antibodies. They offer effector and sentinel functions only at the site of inflammation or injury. Macrophages share certain functional similarities despite their distinct ontogenies and physiological activities. More than embryonic origin or molecular approaches (as identified in epigenomic or transcriptome profiles [8]), these characteristics are characterized by macrophages’ functional capabilities while reacting to immunological challenges. At a steady state, the molecular hallmarks of macrophages, characterized by the expression of cell-type or ontological markers, can be seen. On the other hand, functional hallmarks are only apparent after being activated by PAMPs, DAMPs, cytokines, or antibodies. Our hypothesized functional markers for a healthy macrophage response are shown in Figure 1b: (a) response specificity, or the ability to mount threat-specific immune function, (b) context dependence, or the ability to adjust the threat-specific response to the microenvironmental cytokine milieu, and (c) stimulus memory, or the capacity to recognize earlier stimulus and correctly adapt later threat-specific responses.
Mechanism of macrophage IIM
After discovering the IIM of macrophages, studies on related sub-mechanisms have been carried out successively, and progress has been made in the following significant aspects.
Macrophage surface receptors involved in IIM
Unlike lymphocytes, monocytes and macrophages do not express recombinant antigen-receptor genes; they express pattern recognition receptors (PRRs). PRRs can recognize the structure of foreign antigens-PAMPs. Furthermore, DAMPs, and endogenous hazard signals, generate corresponding responses [15, 16]. PRRs may be involved in immune memory response. After BCG injection, intracellular PRR nucleotide-binding oligomerization domain 2 (PRRNOD2) participates in the protective effect of monocytes against secondary infection [17]. Prestimulation of monocytes with multiple PRRs ligands, such as dectin-1 ligand β-glucan, NOD2 ligand cytomyodyl dipeptide, and toll-like receptor 5 (TLR5) ligand flagellin, resulted in the production of large amounts of tumor necrosis factor-alpha (TNF-α) and interleukin-6 (IL-6) on secondary stimulation. However, TLR4 ligand LPS and flagellin stimulation (10 μg/mL) can induce long-term tolerance of monocytes and reduce the production of pro-inflammatory factors [18]. The IIM involved in forming cell surface receptors will not be lost with cell differentiation. Macrophages differentiated from hematopoietic stem cells, and progenitor cells that are tolerant to TLR2 ligands produce less inflammatory factors and reactive oxygen species in subsequent inflammatory stimulation [19]. This evidence suggests that PRRs may be widely involved in forming IIM. Further research is needed to clarify which PRRs and how they are involved in the process of IIM of specific macrophages.
Macrophage epigenetic reprogramming
Epigenetic inheritance of histone modified innate immune cells
Reprogramming is one of the essential mechanisms of IIM. The epigenetic reprogramming of delicate cells involves a variety of mechanisms, among which protein modification performs an essential task in the formation of IIM. For example, BCG can reprogram the functions of mature neutrophile cells by H3K4me3 modification. Reprogrammed cells showed increased expression of activation markers and enhanced antibacterial function [20]. Monocytes/macrophages exposed to pathogens can undergo changes in histone acetylation and methylation levels, which affect their gene expression patterns upon re-exposure to pathogens [21]. Mononuclear cells stimulated by β-glucan could be divided into macrophages with different phenotypes according to the expression of pro-inflammatory factors TNF-α and IL-6. After training, changes in macrophage function were accompanied by epigenetic changes such as H3K4me1 and H3K27ac. H3K27ac, as a promoter and enhancer activation marker, is in dynamic change, and the H3K27ac marker is gradually eliminated after the stimulus subsides, while the promoter marker H3K4me1 can persist [21]. In addition, a class of enhancers that are usually inactive, unlabeled, and unlinked transcription factors were selectively activated in low LPS stimulation. The H3K4me1 marker was retained after the stimulus was eliminated. Upon secondary stimulation, macrophages labeled with H3K4me1 can quickly activate transcription factors, produce more pro-inflammatory factors, and respond more strongly [22].
In conclusion, H3K4me1 may serve as a marker for macrophages to maintain IIM. BCG inoculation resulted in continued enrichment of H3K4me3 of inflammation-related genes in monocytes of healthy volunteers. It increased the production of inflammatory mediators TNF-α and IL-1β in monocytes after subsequent infection with Mycobacterium tuberculosis, Staphylococcus aureus, and Candida albicans. The protective effect of BCG inoculation on non-specific infection [17]. LPS-induced endogenous immune memory generation in macrophages can increase the phosphorylation level of the transcription factor cyclic associated molecular pattern (AMP)-dependent transcription factor 7 (ATF7). Thus, the methylation level of histone H3K9me2 was decreased, and the resistance of macrophages to Staphylococcus aureus was enhanced [23].
There are also changes in the level of histone modification during tolerance generation. Induced by LPS, H3K9me2 and H3K27me2 changes occurred in proteins of the monocyte/macrophage group, resulting in down-regulation of the expression of inflammatory factor TNF-α [21, 24, 25]. These results suggest that histone modification plays a vital role in forming immune tolerance. Histone modifications differed significantly in training and tolerance phenotypes. Among the trained phenotypes of β-glucan-induced macrophages, 500 gene promoters were changed in H3K27ac and H3K4me3, while there were fewer changes in H3K27ac in LPS-induced tolerance [21]. Thus, β-glucan can induce changes in many gene promoters and enhancers, whereas LPS can induce only small epigenetic changes.
MicroRNAs regulation
MicroRNAs (miRNAs) participate in numerous physiological and pathological processes and play an essential role in generating IIM [26]. Long half-lives characterize these miRNAs changes that occur in myeloid cells and may persist for a long time after the initial stimulation. For example, miR-155 is up-regulated in myeloid cells in response to inflammatory stimuli, which is related to excessive activation of myeloid cells [27]. The expression of miR-221 and miR-222 has increased in bone marrow-derived macrophages during long-term stimulation with LPS in mice. These miRNAs lead to the silencing of inflammatory genes through switch/sucrose non-fermentable (SWI/SNF) and signal transducer and activator of transcription (STAT)-mediated chromatin remodeling, which further promotes the LPS tolerance of macrophages [28].
Metabolic changes of macrophages
During the generation of innate immunity, cellular metabolism in innate immune cells changes, which is associated with the above epigenetic changes [29] and is also an essential mechanism of IIM. Metabolic pathways change when immune cells are activated. Taking macrophage polarization as an example, the metabolic pathways of macrophages with classically activated M1 phenotype and those with alternative activated M2 phenotype are different: M1-type macrophages produce energy by glycolysis [30, 31]. M2 macrophages generate energy mainly through oxidative phosphorylation and have a complete tricarboxylic acid cycle [32]. In response to β-glucan and BCG, a critical link in cellular metabolism is the transition from oxidative phosphorylation to aerobic glycolysis dependent on the Akt/mechanistic target of rapamycin (mTOR)/hypoxia-inducible factor-1α (HIF-1α) pathway [21, 33, 34]. However, macrophages will undergo an intense and transient glycolysis process during the acute response phase [30]. After the stimulus fades, its metabolic mode rapidly changes to oxidative phosphorylation, and deacetylase-1 and deacetylase-6 deacetylate histone deacetylases form an immune tolerance phenotype [35].
In the process of IIM, there is a relationship between histone modification and intermediate products of cellular metabolism. Histone acetylation requires a critical metabolic intermediate, acetyl-coenzyme A (acetyl-CoA), whose elevated levels are in trained monocytes [21]. Tricarboxylic acid cycle intermediates such as fumarate, succinic acid, and α-ketoglutaric acid may regulate the production of IIM. The accumulation of Tamara inhibits the activity of demethylase in the protein of the group [36], induces cholesterol synthesis, leads to the accumulation of mehydroxyprotanic acid, and promotes epigenetic reprogramming during the generation of IIM of monocytes/macrophages by activating the mTOR pathway [37]. The breakdown of glutamine leads to the accumulation of succinic acid and α-ketoglutaric acid, cofactors of an important epigenetic enzyme family. In the process of macrophages producing solid immune memory, succinic acid and α-ketoglutaric acid inhibit lytic acid-specific demethylase 6 (KDM6, also known as JMJD3), resulting in the enhancement of M2-related gene H3K27me3, which inhibits the expression of these genes and makes memory macrophages in an anti-inflammatory phenotype [38, 39]. In classical LPS-induced endotoxin tolerance, α-ketoglutaric acid promotes macrophage M1 activation [39]. These results suggest that cellular metabolism is involved in solid immune memory production. However, the direct evidence that cellular metabolism intermediates affect histone modification enzymes is still needed to find further.
The role of macrophage IIM in disease
Innate immune cell immune memory enables the body to make an enhanced or weakened response to secondary stimuli, which plays a role in some diseases. Preorder studies have found that macrophage IIM plays a role in infectious diseases, transplant rejection, atherosclerosis, autoinflammatory diseases, and tumors.
Infectious diseases
The innate immune system is critical to microbial and antiviral defense. As part of the innate immune system, monocytes/macrophages help fight off infections from bacteria and viruses. Memory macrophages play a protective role in both primary and secondary infection of the same or different pathogens. Staphylococcus aureus is a significant reason of skin and skin structure infection (SSSI). Macrophages with IIM function are protective in the recurrent mouse SSSI model. It reduces the severity of skin damage. Stimulated macrophages can still provide in vivo protection after adoptive transfer to the original skin [40]. In the secondary infection of mice previously infected with Staphylococcus aureus, the stimulated macrophages recruited monocytes faster, increased bacterial killing rate, accelerated healing speed and enhanced resistance to the second infection [41]. Alveolar macrophages (AMs) can be induced by primary infection (e.g., respiratory tract virus infection) with two different pathogens. Memory AMs are reprogrammed with high expression of major histocompatibility complex (MHC)-II molecules and increased glycolysis metabolism. In the case of secondary infection with Streptococcus pneumoniae and Escherichia coli, memorizing AMs produces more chemokines and chemotactic neutrophils to reach the lungs to enhance the body’s resistance to bacterial infection [42]. LPS stimulated macrophages can show more vigorous killing activity when encountering Staphylococcus aureus [23]. In conclusion, macrophage IIM plays an essential role in resistance to re-infection.
Transplant rejection
Chronic allograft rejection has long been linked to the role of monocytes/macrophages in generating inflammation and modulating adaptive immunological responses [43–45]. In recent years, researchers have found that macrophages’ IIM is involved in transplant rejection [46]. After the adoptive transfer of macrophages prestimulated by alloantigen into MHC matched immune-deficient mice, the latter acquired a strong specific ability to reject skin grafts for at least 120 days. Acquiring this ability requires the assistance of CD4+ T cells [47, 48]. Macrophages can also recognize specific molecules and generate memory. For example, mouse macrophages can obtain the memory of specific recognition of MHC-I molecules. These memory responses involve MHC-I receptors [type A paired immunoglobulin-like receptors (PIR-A)]. In mouse models of kidney and heart transplantation, deletion of recipient PIR-A or blocking the binding of PIR-A to donor MHC-I molecules can block the memory response and alleviate the rejection reaction [49]. The memory of innate immunity is also present in human transplant cases. In liver transplantation, mononuclear phagocytes (MNPs) from recipient blood are partially reprogrammed to obtain strong expression of CD68/CD206 in human leukocyte antigen (HLA)-mismatch allograft [50]. It is suggested that these cells may influence the recipient’s tolerance to the graft. Macrophages can acquire IIM for recognizing alloantigens or specific molecules, and blocking this memory may be a potential means to improve the effect of transplantation.
Atherosclerosis
Monocytes and macrophages play critical roles in all stages of atherosclerosis [51], and their IIM also plays a vital role in disease progression. In addition to classical innate immune inducers β-glucan, BCG, and LPS, endogenous non-microbial atherogenic stimulus such as oxidized low-density lipoprotein (oxLDL) and lipoprotein(a) [Lp(a)] also induce IIM in monocytes/macrophages [52]. Transient stimulation of oxLDL and Lp(a) in vitro induced human monocytes to become macrophage phenotypes. When stimulated by TLR2 and TLR4 ligands again, the production of pro-atherogenic factors such as TNF-α and IL-6 in monocytes/macrophages increased significantly [52, 53]. oxLDL-stimulated macrophages can produce more collagenases such as matrix metalloproteinases 2 (MMP-2) and MMP-9, which play an essential role in the instability of atherosclerosis plaque [52]. High cholesterol can also induce immune memory production. Monocytes in patients with familial hypercholesterolemia are characterized by increased TNF-α production. Their promoters are rich in H3K4me3 markers, which cannot be eliminated after 3 months of cholesterol-lowering statins [54], suggesting that these patients are at increased risk of atherosclerosis. Therefore, the IIM of monocytes/macrophages plays a role in atherosclerosis, and its specific mechanism needs to be further explored.
Autoinflammatory diseases
In autoinflammatory diseases, pathogenic inflammation is mainly caused by the overactivation of antigen-independent immune pathways [55], in which the IIM of macrophages plays an important role (Figure 2). A significant feature of autoinflammatory diseases is the excessive production of IL-1β, so anti-IL-1 therapy has become a broad-spectrum approach for treating autoinflammatory diseases [56]. IL-1β is a critical disease factor and an essential inducer of IIM production. Studies have shown that the mortality of mice reinfected with bacteria after injection of IL-1β is significantly reduced [57]. Human monocytes prestimulated by IL-1β in vitro produced more IL-6 and TNF-α under LPS stimulation, and further studies showed elevated H3K4me3 levels in the promoter regions of IL-6 and TNF-α [58]. These findings suggest that IL-1β can induce the production of IIM and may lead to excessive inflammation, suggesting that IL-1β may play an essential role in autoimmune diseases. In addition to IL-1β, β-glucan is also a classical inducer of IIM. β-glucan can reprogram macrophages in patients with inflammatory disease, reducing the production of IL-1β mediated by nucleotide-binding oligomerization domain-like receptor family pyrin domain-containing-3 (NLRP3) inflammasome and inhibiting inflammation [59], suggesting that β-glucan can reprogram macrophages to obtain immune memory and reduce pathogenic inflammation. The function of macrophage IIM in autoimmune diseases needs to be further explored.
The tumor
Innate immune cells play different roles in tumor progression and finally show two opposite results: inhibiting and promoting tumors. When the innate immune system is activated effectively, it can play an anti-tumor role, and IIM can also play an important role in anti-tumor immunity. β-glucan, for example, induces an IIM of granulocytes that lasts for at least 28 days. Neutrophils can be reprogrammed into anti-tumor phenotype through transcription and epigenetic changes during the process of neutrophils producing IIM to exert anti-tumor activity and inhibit tumor growth. This process requires to type I interferon signaling and is independent of host adaptive immunity [60]. There are significant differences between tumor-associated macrophages (TAMs) and tumor-associated neutrophils in the mode of action, mechanism of action, duration of action, and effect on tumor progression. The innate memory of immunity may also be different between the two. Macrophage IIM can also play an anti-tumor role. Existing studies speculated that BCG-induced macrophage IIM might play a role in the tumor microenvironment. The anti-tumor effect of BCG has long been known. BCG can be used to treat bladder cancer [61], melanoma [62], and other malignant tumors and reduce the risk of leukemia [63] and lymphoma [64]. Further studies have revealed that this effect is achieved by providing non-specific protection through innate immune-dependent mechanisms involving histone H3K4me3, which is related to the generation of IIM of monocytes/macrophages [13, 17]. Long-term IIM of macrophages induced by BCG therapy may also play an essential role in preventing tumor recurrence.
In many cases, innate immune cells contribute to tumor progression. Innate immune cells invade the area around the tumor and become part of the tumor microenvironment. Inflammatory microcells in the tumor microenvironment usually promote tumor progression, and they can promote tissue remodeling, angiogenesis, and fibrosis, creating a microenvironment conducive to tumor growth [65]. The tumor microenvironment can functionally reprogram disease-free cells to counteract anti-tumor effects and promote the production of inflammatory states that are often associated with disease progression [66]. Epigenetic reprogramming of TAM in the tumor microenvironment is a core feature of TAM differentiation due to long-term histone modification. For example, changes in H3K4me3 and H3K9me3 in the promoter regions of IL-6 and TNF-α lead to increased TAM pro-inflammatory factors, expressed as tumor-promoting gene expression profiles [67]. The role of macrophage IIM in the tumor microenvironment remains further explored.
Conclusions
It has been ten years since IIM was proposed, which has attracted more and more attention, and the related fields have made significant progress. However, the research on IIM is still in its infancy, and there are still many new problems that deserve further exploration. Macrophages’ IIM is an integral part of it (Figure 1). Since macrophages play a crucial role in host immune response, an in-depth understanding of the mechanism of macrophages’ IIM and its role in diseases can not only promote the study of IIM. It can also provide valuable clues for elucidating the pathological mechanism of disease occurrence and development and developing new therapeutic methods. There are still many scientific issues in macrophage IIM that deserve further research. Firstly, the mechanism of macrophage IIM generation and which signaling pathways mediate cell metabolism and epigenetic changes are elucidated from the molecular level. Secondly, we elucidate the decay mechanism of macrophage IIM from the molecular level, which factors affect the intensity and duration of macrophage IIM. Thirdly, the characteristics and action mechanism of macrophage subpopulations involved in immune memory and the interaction mechanism between macrophages of each subpopulation and other innate and adaptive immune cells.
The rapid development of single-cell transcriptomics and epigenomics has provided a necessary means to identify potential new memory subpopulations of macrophages and the mechanisms by which they produce IIM. There have been in-depth studies on the IIM of macrophages in infection, transplantation, atherosclerosis, and autoimmune diseases. Although the critical role of TAM in the tumor microenvironment has been widely recognized [68, 69], the role and mechanism of TAM in tumor proliferation, migration, invasion, and metastasis have attracted much attention [70]. However, the research on the role of macrophage IIM in the tumor is relatively lagging, and there is a broad space for research. Leukemia is a hematological malignancy. The author’s team has carried out a series of studies on the leukemia immune microenvironment, primarily focusing on the leukemia macrophage-associated macrophage (LAM) in the leukemia microenvironment [71–75]. There were significant differences between LAM and TAM.
LAM population has substantial heterogeneity: there are significant differences in gene expression, miRNA expression, phenotype, and function of LAM in different tissues. M1-like and M2-like LAM subsets can exist in the same tissues, and the subsets change with the progression of the disease. There are significant differences in phenotype and function of LAM from different sources. Mononuclear cell-derived LAM has more significant M1-type macrophage characteristics and plays a pathological role in extramedullary infiltration of acute T lymphoblastic leukemia (T-ALL) cells. LAM expresses both M1 and M2-macrophage-related genes, and intervention of LAM with more M1 macrophage properties can delay the progression of leukemia [74, 76–81]. In addition, macrophages can enhance the resistance to leukemia cell therapy [82] and reduce graft-versus-host reaction after bone marrow transplantation [83, 84]. However, does LAM have immune memory function? What is the mechanism? Does macrophage immune memory affect the progression and treatment of leukemia, and can it be used to play a role in the immunotherapy of leukemia? All these problems remain to be solved, and further research may provide new perspectives and potential solutions for elucidating the pathogenesis of leukemia and solving the recurrence after treatment and rejection after bone marrow transplantation.
Abbreviations
AMs: | alveolar macrophages |
BCG: | Bacillus Calmette-Guerin |
DAMP: | damage-associated molecular pattern |
H3K4me3: | tri-methylation of lysine 4 on histone H3 |
IIM: | innate immune memory |
IL-6: | interleukin-6 |
LAM: | leukemia macrophage-associated macrophage |
LPS: | lipopolysaccharide |
MHC: | major histocompatibility complex |
miRNAs: | microRNAs |
NK: | natural killer |
oxLDL: | oxidized low-density lipoprotein |
PAMP: | pathogen-associated molecular pattern |
PIR-A: | type A paired immunoglobulin-like receptors |
PRRs: | pattern recognition receptors |
TAMs: | tumor-associated macrophages |
TLR5: | toll-like receptor 5 |
TNF-α: | tumor necrosis factor-alpha |
Declarations
Author contributions
SUK contributed the conception, drafting, literature search and artwork of the study; MUK did the drafting, artwork and literature search. All authors contributed to manuscript revision, read and approved the submitted version.
Conflicts of interest
The authors declare that there are no conflicts of interest.
Ethical approval
Not applicable.
Consent to participate
Not applicable.
Consent to publication
Not applicable.
Availability of data and materials
Not applicable.
Funding
Not applicable.
Copyright
© The Author(s) 2022.