Abstract
The study investigated the role of probiotics and prebiotics in treating COVID-19. It focuses on their mechanisms of action in modulating the immune system and mitigating the disease’s impact. The research focused on the significance of the gut-lung axis and the potential benefits of probiotics and prebiotics against COVID-19. The research identified several findings: probiotics have shown potential in reducing the severity of COVID-19 symptoms and improving patient outcomes. The gut microbiota plays a critical role in the body’s immune response to COVID-19, with probiotics aiding in restoring its balance. Both can modulate the immune system, reducing the inflammatory response associated with COVID-19. While there is evidence supporting the benefits of probiotics and prebiotics, limitations exist due to the lack of studies on their use to improve COVID-19 outcomes. The originality of the study is focused on the gut-lung axis and the review of probiotics and prebiotics as potential therapies for COVID-19. By analyzing recent literature, the research contributes to understanding the interactions between diet, gut health, and respiratory infections. It also shows the need for further studies to determine the efficacy of COVID-19, toward new directions for research in the emerging field.
Keywords
Probiotics, prebiotics, mechanisms of action, COVID-19, immunological systemIntroduction
Coronavirus disease (COVID-19) is an infection caused by the SARS-CoV-2 virus. Coronaviruses are spherical-shaped and moderately pleomorphic microorganisms, although this virus appears as a crown [1]. The new coronavirus (SARS-CoV-2) is a strain not previously identified in humans [2].
Thus, in December 2019, SARS-CoV-2 emerged and spread to virtually every city in the world, causing a global health problem because it is an unprecedented and unknown zoonosis. Among those who developed symptoms, currently, 80% have recovered from the disease without the need for hospital treatment, about 15% develop severe disease and require oxygen, while 5% reach a critical state and require intensive care [2]. As a result, a global vaccination campaign was initiated. Currently, the technology achieved by humankind allowed Russia to register the first vaccine in August 2020. With the registration of new vaccines by several developed countries, representatives from different sectors around the world created various vaccines: Russia (Sputnik V), USA (Moderna), Germany (BioNTech-Pfizer), China (Sinopharm and Sinovac), Sweden and Great Britain (Oxford-AstraZeneca), and India (Covaxin) [3].
However, there is currently no specific drug for the treatment of COVID-19, as the development of new antivirals requires drug validation. Therefore, it has been necessary to implement alternative therapies or to consume supplements or foods containing probiotics and prebiotics, as they demonstrate some effectiveness against this disease.
Likewise, people with an altered microbiota are more susceptible to COVID-19. Therefore, probiotic supplementation has been reported to improve the ability of the gastrointestinal microbiota to modulate immune activity. Patients who consume foods high in prebiotic content have been shown to have a lower risk of COVID-19 complications and better post-disease recovery [3].
Among the alternatives, scientists suggest that probiotics serve as a preventive treatment for COVID-19, as these functional foods possess anti-inflammatory properties during viral infections and contribute to preventing bacterial superinfections. In this investigation, a narrative review of the literature was conducted to achieve the objective of this article: to identify probiotic strains and the intake of prebiotics, as well as the mechanisms of action of probiotics in the management and treatment of COVID-19.
During the COVID-19 pandemic, the disease has exhibited influenza-like infection behaviors. This is reported in a randomized controlled trial (RCT), where the effect of the probiotic Lactobacillus plantarum DR7 on upper respiratory tract infections was investigated. This study was conducted in Malaysia with a total of 124 subjects (ages 18–60), who were divided into two groups: 62 subjects received L. plantarum DR7 for 12 weeks, while the rest received a placebo (maltodextrin 100%). The results showed that the administration of L. plantarum could lead to a suppression of plasma pro-inflammatory cytokines (IFN-γ, TNF-α) in middle-aged adults (ages 45–60), and an enhancement of antiinflammatory cytokines (IL-4, IL-10) in young adults (ages 20–45), along with reduced levels of plasma peroxidation and oxidative stress. These findings suggest that oral probiotic consumption may enhance the immune response to combat this disease and future pandemics, as the digestive tract is a focal point of the body’s defenses and contributes to preventing viral infections [4].
This study is of great relevance as it contributes to the understanding of the interactions between gut health and respiratory infections, specifically in the context of COVID-19. The findings of this research could influence the development of alternative and complementary therapies using probiotics and prebiotics to improve outcomes in patients affected by COVID-19. In addition, it highlights the need to continue to investigate the efficacy and safety of these supplements in different populations, which could open new directions for research in this emerging field.
The present study has as its main objective to investigate the role of probiotics and prebiotics in the treatment of COVID-19, with a particular focus on their mechanisms of action to modulate the immune system and mitigate the impact of the disease. It focuses on the relevance of the gut-lung axis and the potential benefits that these substances could offer against COVID-19.
Results and discussion
The research focused on identifying relevant documents, with particular emphasis on the quality and relevance of publications related to COVID-19. Although the search was limited to 2010–2023, no such restriction was imposed when addressing the background and contextualization of the problem. Priority was given to including randomized controlled clinical trials and systematic reviews to ensure a rigorous and informed analysis.
The selection of publications was initially carried out by evaluating titles and abstracts, highlighting those that significantly addressed critical aspects related to the study problem. Subsequently, the full texts of the selected documents were obtained, allowing for a more detailed and exhaustive examination of the available information. This methodological approach was adopted to ensure the incorporation of solid, up-to-date research into the overall topic analysis.
Intestinal microbiota
Microbiota refers to the community of living microorganisms resident in each ecological niche. They are found in different body parts, mainly in the intestine, skin, mouth, and genital areas. Such microorganisms influence the human body’s development, physiology, immunity, and nutrition [5].
The intestinal microbiota is considered a “metabolic organ” with functions related to nutrition, immunity regulation, and systemic inflammation [6]. The composition and effects of the human microbiota, particularly the amount of bacteria present in the gastrointestinal tract, are influenced by several factors from birth to adulthood. For example, the route of birth (vaginal delivery or cesarean section) and the type offending at birth (breast milk or formula) have been shown to produce differences in gut microbiota [7]. Meanwhile, during infancy and throughout life, the composition of the microbiota changes according to age and diet.
Functions of the intestinal microbiota
The intestinal microbiota regulates the immune system’s modulatory function by recognizing harmful elements through intestinal components, producing mediators, and generating responses to maintain intestinal immune homeostasis [7, 8]. In addition to its protective function, the microbiota plays a fundamental role in nutrition, as it:
Regulates intestinal transit
Synthesizes essential amino acids such as lysine and threonine
Synthesizes vitamins K and B12
Synthesizes polyunsaturated fatty acids such as conjugated linoleic acid
Promotes the digestion of lactose
Enables the utilization of soluble dietary fiber
Facilitates the absorption of minerals such as magnesium, calcium, and iron
The critical role of gut microbiota in modulating the innate and adaptive immune systems has led to multiple recent studies on the effect of probiotics during the COVID-19 pandemic, with several projects underway. Currently, there are several clinical studies in the United States, the first of which is evaluating the effect of Lactobacillus rhamnosus GG on the microbiome of people exposed at home who have had contact with COVID-19-positive patients. Research on Lactobacillus coryneform K8 for the prevention of healthcare workers is in progress, along with another study on oxygen-ozone as an adjuvant in the early treatment of COVID-19, focusing on the progression and modulation of the microbiota (Probiozovid), and bacteriotherapy in the treatment of COVID-19 (BACT-COVID) [9, 10].
SARS-CoV-2 infections frequently cause gastrointestinal inflammation, with clinical presentations ranging from intestinal cramps and diarrhea to intestinal perforations (Figure 1) [11, 12]. Furthermore, gastrointestinal symptoms were more common in critically ill patients requiring intensive care than in those who did not require it, with 10% of patients experiencing diarrhea and nausea 1 to 2 days before the development of fever and respiratory symptoms.
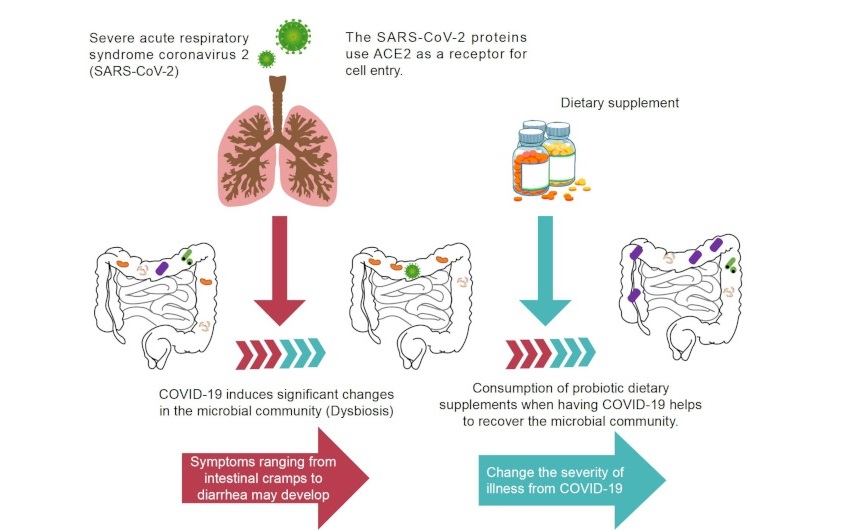
Gastrointestinal involvement and altered gut microbiota during COVID-19 and recovery by probiotic dietary supplementation. ACE2: angiotensin-converting enzyme 2
Note. Adapted from “Gut Dysbiosis during COVID-19 and Potential Effect of Probiotics” by Hung YP, Lee CC, Lee JC, Tsai PJ, Ko WC. Microorganisms. 2021;9:1605 (https://www.mdpi.com/2076-2607/9/8/1605). CC BY.
However, evidence detailing successful therapy for gastrointestinal involvement in patients with COVID-19 is currently limited [13].
Probiotics
Probiotics refer to products that contain viable strains of microorganisms in quantities suitable for altering the host microflora (either by colonization or implantation) and producing beneficial effects in that host. This definition includes products containing microorganisms (such as fermented milk, yogurt, or sauerkraut) as well as tablets or powders that serve as dietary supplements [14].
Recent scientific research on the properties and function of live microorganisms in foods indicates that probiotics play an essential role in immune, digestive, and respiratory functions. They are thought to positively alleviate infectious diseases in children and other high-risk groups, including patients who have developed COVID-19 [15].
According to [16], probiotics are live microorganisms that benefit the host’s health when administered in sufficient quantities. They contain enough live organisms to positively alter the intestinal microbiota by colonizing the intestine. For a microorganism to be classified as a probiotic, it must meet specific characteristics: it should be a typical inhabitant of the human intestine, neither pathogenic nor toxigenic, able to survive the acidic environment of the stomach and the effects of bile in the duodenum, capable of adhering to epithelial cells, able to adapt to the intestinal microbiota without displacing the existing normal microbiota, capable of producing antimicrobial substances, and able to enhance immune functions and metabolic activities.
Examples of probiotic microorganisms commonly used for human consumption include lactic acid bacteria (LAB), which comprise Lactobacilli and Bifidobacteria, as well as non-pathogenic bacterial strains such as Streptococcus, Enterococcus, and yeasts like Saccharomyces boulardii, which is non-pathogenic.
The Lactobacillus genus (from the Latin lactis, meaning milk, and bacillus, meaning rod) consists of gram-positive, catalase-negative, non-spore-forming, and microaerophilic bacteria. They use carbohydrate fermentation to produce lactic acid [14]. These bacteria can be found on the skin and mucous membranes, improving immune system function. Therefore, their primary functions include:
Epithelial cell adherence
Lactic acid production
Hydrogen peroxide production
Bacitracin production
Interleukin production
Nutrient competition
They can act against pathogens (including SARS-CoV-2) by competing for nutrients through cell adhesion, thereby preventing pathogens from initiating infection, or by producing bacitracin, which inhibits pathogen growth. Consequently, they are essential elements of the defense system, as they stimulate interleukin production by endothelial and lymphocyte cells, acting as chemical mediators [17].
Bacteriocins
Bacteriocins are bacterial peptides (formed by positively charged amino acids) that form pores and inhibit the growth of various bacteria. They are also used as preservatives added to minimally processed food [18, 19].
These compounds are produced by LAB, which are considered generally recognized as safe (GRAS) and are used as bio-preservatives because they act against several microorganisms across a wide pH range and demonstrate thermostability. So far, the FDA has approved nisin, pediocin, and Micocin® for use in food [20].
Bacitracin production depends on the growth and physiological activity of the producing strain, reflecting the relationship between biomass and the amount of bacitracin produced [21]. Its primary action mechanism involves the formation of pores or ionic channels through destabilization and permeation of the membrane, causing the exit of nutrients such as phosphate, potassium, amino acids, and ATP, ultimately leading to decreased macromolecule synthesis and cell death.
Cintas et al. (2001) [22] proposed using mixed bacteriocins to reduce the ability of other microorganisms, such as COVID-19, to develop resistance.
Nystatin is an antifungal compound produced by Streptomyces noursei. Due to its chemical structure, it is categorized as a polyene antifungal that can exist in three forms: type A, B, and C. The most common A and B forms can interconvert under certain environmental conditions.
Additionally, [22] established that nystatin is an antimicrobial that binds with steroids, inhibiting caveolae-dependent endocytosis and eliminating membrane cholesterol, which is necessary for caveolae maintenance and membrane separation. It was demonstrated in vitro that nystatin inhibits endocytosis induced by respiratory virus glycoproteins (such as SARS-CoV-2), with results showing between 49% and 93% inhibition of syncytial formation induced by Newcastle disease in COS-7 and HeLa cells.
Some advantages of nystatin for oral consumption include its safety for all ages, from neonates to adults, including immuno-compromised individuals. Once nystatin is administered orally, it rapidly penetrates the pharyngeal, esophageal, and gastrointestinal mucosa, protecting these areas against the COVID-19 virus, with minimal systemic absorption, resulting in few significant side effects [23].
Nisin is a polypeptide produced by various strains of Lactococcus lactis and Streptococcus lactis during fermentation in a modified dairy medium. It is approved and used by the WHO as a preservative in the food industry [24] and is classified as a GRAS substance by the FDA due to its antibacterial properties, minimal impact on food sensory qualities, and easy degradation in the human digestive system [25].
According to [26], this bacteriocin is classified as a class I antibiotic because it is a small, polycyclic, heat-stable peptide, active at the membrane level, containing amino acids such as lanthionine, β-methyl-lanthionine, and dehydroalanine, which are formed post-translationally through the dehydration of serine and threonine.
Due to its low molecular weight, nisin’s high bioavailability (especially for nisin H and Z) allows it to bind with angiotensin-converting enzyme 2 (ACE2), potentially preventing the COVID-19 virus from attaching to the same site.
Finally, since nisin is a heat-stable natural food-grade peptide, it can be produced cost-effectively, even in large quantities, through microbial fermentation [27].
Mechanisms of action
Among the probiotic effects attributed to LAB, some mechanisms of action, such as the reduction of lactose intolerance, are well-researched. In contrast, others—such as antitumor properties or effects on hypocholesterolemia—require further in vitro studies and natural and experimental models to confirm their mechanisms of action.
Several mechanisms have been proposed for the effectiveness of probiotics in enhancing host resistance against pathogenic organisms. According to Vizcaíno-Carruyo et al. [28]:
Probiotics like L. rhamnosus GG improve lung barrier function and homeostasis by increasing regulatory T cells, enhancing antiviral defenses, and decreasing pro-inflammatory cytokines, which can help reduce respiratory infections. These immunomodulatory benefits are significant for individuals infected with or previously infected by COVID-19.
Probiotics produce antimicrobial substances such as lactic acid, short-chain fatty acids, hydrogen peroxide, diacetyl, and bacteriocins. These compounds reduce the number of viable pathogenic cells and can affect bacterial metabolism or toxin production. In the food industry, LAB are used as biological preservatives to produce bacteriocins that exert antibacterial action and help prevent food spoilage.
Probiotics lower intestinal pH, promoting the growth of beneficial organisms.
They increase resistance to colonization by competing with pathogens for adhesion sites on the intestinal epithelium. Certain strains, such as Lactobacillus, are selected for their ability to adhere to epithelial cells.
Probiotics compete for nutrients, utilizing there’s ounces consumed by pathogenic organisms.
The beneficial properties of probiotic strains in the intestine justify their inclusion in fermented dairy products, particularly those that support the immune system. LAB in fermented products should not only induce immunostimulation at the mucosal level but also ensure the absence of side effects, such as microbial translocation (the passage of microbes through the intestinal mucosa to extraintestinal organs) and alterations in intestinal permeability due to exacerbated inflammatory responses. Therefore, it is crucial to evaluate the ability of probiotic strains to interact with the intestine and its associated immune cells [29] (see Figure 2).
Prebiotics
Prebiotics are foods, typically high in fiber, that serve as nutrients for the human microbiota, enhancing the balance of these microorganisms. They are undigested food ingredients, such as inulin and fructooligosaccharides, that beneficially affect the host by stimulating the growth or activity of beneficial intestinal bacteria like Bifidobacterium in the colon.
Symbiotics are products that contain both prebiotics and probiotics, creating a synergistic effect that enhances the benefits to the host. This term refers to products where the prebiotic components selectively favor the probiotic components. When probiotics and prebiotics are incorporated into foods during manufacturing or added as supplements, functional foods are created—foods that provide benefits beyond their nutritional value, such as fermented milk, yogurt, cheeses, or juices. Unlike probiotics, most prebiotics are used as food ingredients in products like cookies, cereals, chocolates, spreads, and dairy products [30]. Some well-known prebiotics include: oligofructose, inulin, galacto-oligosaccharides, lactulose, and oligosaccharides from breast milk [30].
Prebiotics must possess key properties: they should be non-hydrolyzed natural products, resistant to hydrochloric acid, non-absorbable by digestive enzymes, and capable of modifying gut microbiota through bacterial fermentation [19].
Other prebiotics known for their health benefits include kimchi, sourdough bread, and processed starches from wheat and barley, as well as isomalt-oligosaccharides (IMO) [31] and xylooligosaccharides (XOS) derived from corn cob hydrolysis, transgalactooligosaccharides (TOS) from milk, and soy oligosaccharides (SBOS) [32].
Prebiotic consumption primarily increases Bifidobacterium levels, leading to the production of acetic, propionic, and butyric acids, which help inhibit pathogens alongside probiotics [33] (see Figure 3).
SARS-CoV-2 and the immune system
According to [34], coronaviruses are pathogens that primarily attack the human respiratory system. Thus, reinforcing this system is essential to enhance immune responses against any pathogen. Actions such as maintaining a balanced diet supplemented with immune-stimulating elements can lead to a reduced propensity to become ill, experience severe symptoms, or recover more quickly [35, 36].
When nisin is added, its bacteriostatic mode of action halts colony growth without causing cell death. At a pH of 6.5, a decrease in optical density is observed, indicating that the number of microorganisms present falls below 103 CFU/mL. This demonstrates that nisin acts bacteriolytically on Lactobacillus fructivorans at nearly neutral pH levels. The three steps in the dual mechanism of action of nisin from Lactococcus lactis are as follows:
1. Nisin, being positively charged, enhances its interaction with the negatively charged components of the cell wall.
2. Nisin binding to lipid II (the primary transporter of peptidoglycan subunits from the cytoplasm to the cell wall) interferes with peptidoglycan synthesis, leading to bacterial cell death.
3. The insertion of multiple nisin molecules into the cell membrane via lipid II results in pore formation, contributing to cell death.
Recent studies indicate that COVID-19 can cause respiratory and gastrointestinal disorders, heart damage, and even death within 6 to 41 days, depending on the patient’s age, clinical conditions, and immune system status [34]. Within our body, soluble substances called cytokines are produced by cells; these act as projectiles that locate and selectively destroy infected cells [17, 19]. Cytokines are crucial in the immunopathology of viral infections. As noted in studies on COVID-19 pathogenesis, a cytokine storm can lead to heightened inflammation and cell damage [37].
Experiments show that the addition of nisin leads to consistent readings throughout the duration of the experiment. The plate count indicates that nisin acts bactericidally on L. fructivorans at pH 3.5, as the number of microorganisms decreases over time.
Due to nisin’s cationic and hydrophilic characteristics, its mechanism of action on cell membrane permeability to involves either (a) acting as a “pore” complex in which is in monomers bind, insert, and oligomerize in the cytoplasmic membrane to form a pore—this is the most widely accepted model—or (b) destabilizing the membrane similarly to a detergent.
Clinical cases and empirical data
This research consulted various clinical cases and empirical data from recent studies to illustrate the benefits of probiotics and prebiotics, as well as their mechanisms of action, in patients infected with COVID-19 and their responses to treatment.
The findings from different clinical cases of patients with COVID-19 are as follows.
Probiotics, recognized for restoring equilibrium to the intestinal microbiota, played a significant role in reducing the duration of various symptoms, including general malaise, respiratory distress, and digestive issues. This was observed in a study involving a cohort of 300 adult patients who tested positive for SARS-CoV-2. These findings underscored the impact of COVID-19 on the microbiota and highlighted the tangible benefits of incorporating probiotics into the therapeutic regimen. This multifaceted improvement contributed to an overall reduction in disease severity.
Moreover, the utility of probiotics extends beyond COVID-19, proving beneficial in addressing common respiratory infections such as influenza, syncytial virus, and other coronaviruses. In research conducted by [38], all patients received standard treatments, including medications like hydroxychloroquine, azithromycin, and tocilizumab. Notably, a subset of patients was randomized to receive a probiotic mixture comprising eight strains: Lactobacillus brevis, Lactobacillus acidophilus, Lactobacillus helveticus, Lactobacillus paracasei, L. plantarum, Streptococcus thermophilus, and Bifidobacterium lactis, administered over two weeks. This integrative approach shows promise for enhancing overall management and outcomes in COVID-19 and related respiratory infections.
The study's outcomes highlighted the rapid resolution of diarrhea in patients who incorporated probiotics, with a remarkable 93% recovery rate by the third day, compared to only 7% in those who did not participate in the probiotic regimen [39]. Additionally, the research indicated a significant increase in the risk of respiratory failure among patients who omitted the probiotic mixture from their treatment.
In a parallel investigation, researchers compared infection patterns between COVID-19 and influenza. These observations were meticulously documented in an RCT exploring the effects of the probiotic L. plantarum DR7 on upper respiratory tract infections. The results showed that administering L. plantarum led to a suppression of plasma pro-inflammatory cytokines (IFN-γ, TNF-α) in middle-aged adults (ages 45–60) and an increase in antiinflammatory cytokines (IL-4, IL-10) in younger adults (ages 20–45). This compelling evidence suggests that oral probiotic consumption enhances immune response, bolstering the body’s ability to combat he effects of the disease [4].
Additionally, in-depth research by [12] examined the occurrence of diarrhea among critically ill COVID-19 patients and the effectiveness of integrating probiotics into their treatment. The study established that probiotics reduce diarrhea symptoms, regardless of origin, while also improving nutritional quality and mitigating the effects of the “cytokine storm”, ultimately leading to a more favorable prognosis.
Table 1 presents the characteristics, key studies, and findings regarding the benefits of probiotics from various case studies obtained from diverse databases [40–48].
Key studies, characteristics, and finding detailed of results in this review
Study design | Key studies, characteristics, study population, intervention, effect of microbiota | Findings | Reference |
---|---|---|---|
Meta-analysis | Increased beneficial bacteria, reduced harmful bacteriaType 2 diabetes patientsVarious probiotic strains | Probiotics improve glycemic control and modulate gut microbiota | Tao et al. (2020) [41] |
Randomized controlled trial | Increased production of SCFAsHealthy adults Lactobacillus and Bifidobacterium strains | Probiotics enhance the production of beneficial SCFAs in the gut | Markowiak-Kopeć et al. (2020) [42] |
Consensus statement | General improvement in gut microbiota balanceN/AVarious probiotic strains | Standardized definition and guidelines for probiotic use | Hill et al. (2014) [43] |
Randomized controlled trial | Reduced diarrhea incidence, increased beneficial bacteria Malnourished childrenLactobacillus rhamnosus GG | Probiotics reduce diarrhea incidence in malnourished children | Amadi et al. (2020) [44] |
Animal study | Improved gut microbiota composition, enhanced growthInfant miceLactobacillus rhamnosus GG | Early-life probiotics improve gut health and growth in mice | Sindhu et al. (2020) [45] |
Systematic review & Meta-analysis | Improved gut microbiota diversity, reduced depressive symptomsDepression patientsLactobacillus and Bifidobacterium strains | Probiotics may alleviate depressive symptoms by modulating gut microbiota | Xiao et al. (2021) [46] |
Animal study | Modulated gut microbiota composition, improved metabolic health Mice on high-fat dietLactobacillus and Bifidobacterium strains | Probiotics counteract high-fat diet-induced gut microbiota changes | Tomas et al. (2020) [47] |
Randomized controlled trial | Improved gut microbiota diversity, enhanced immune responseRheumatoid arthritis patientsLactobacillus casei | Probiotics improve immune response and gut health in rheumatoid arthritis | Ferro et al. (2021) [48] |
Self-elaboration with adapted data from [40]. SCFAs: short-chain fatty acids; N/A: not available
A noteworthy study uncovered that hospitalized patients with confirmed COVID-19 diagnoses exhibited dysbiosis, indicating an imbalance in their gut microbiota. This dysbiosis was accompanied by a significant reduction in anti-inflammatory bacteria, such as Faecalibacterium prausnitzii. An inverse correlation was observed between ACE2 levels and the SARS-CoV-2 viral load [46]. This correlation suggests a potential link between gut microbiota composition and these varieties of viral infections, providing valuable insights into the intricate interactions between the microbiome and COVID-19 outcomes.
However, an examination of various controlled trials by [37] challenged some anticipated outcomes. Despite this, a significant reduction in symptoms related to COVID-19 was observed. In a separate study involving 300 adult patients with COVID-19 symptoms, although no discernible change in fecal microbiota was found among those administered probiotics, there was a substantial increase in the levels of both IgM and IgG compared to individuals in the placebo group. This suggests that while the impact on inflammation markers may be limited, probiotic intervention could enhance the immune response, as evidenced by the increased levels of the secrucial immunoglobulins.
Dose-effect relationship
Probiotic doses of 108 to 1010 CFU must be consumed daily to produce health benefits in humans [38]. Patients most susceptible to SARS-CoV-2 are those with altered microbiota, including older adults and immunosuppressed individuals. Thus, probiotic supplementation is recommended for these groups, as it could improve the gastrointestinal microbiota’s ability to modulate immune activity and help prevent viral infections, including COVID-19 [39]. Studies have reported that Lactobacilli possess immunomodulatory effects that can protect against viral infections by enhancing antiviral cytokine responses in the respiratory tract, immune cells, and intestinal mucosa [4].
Limitations
There is insufficient concrete evidence to assert that probiotics can combat or eliminate the coronavirus, as highlighted by the National Academies in 2022 [12], which emphasizes the need for extensive research to establish the effects of probiotics on viral infections across diverse demographic segments, considering factors such as age, gender, and individual habits. These studies should focus on individuals with severe illnesses that compromise their immune systems, as probiotic consumption may lead to adverse health effects in such cases.
Potential side effects must also be considered. Some individuals may experience discomforts such as gas, bloating, or upset stomach when incorporating probiotics into their regimen. Caution is warranted regarding possible drug interactions, necessitating consultation with a healthcare professional before starting any supplement regimen. For instance, antibiotics can indiscriminately eliminate both harmful and beneficial bacteria, thereby diminishing the positive effects of probiotics.
Other limitations include the growing body of evidence suggesting that probiotics and prebiotics can positively influence immune response and modulate inflammation. However, much of this evidence stems from preclinical studies or small clinical trials with methodological limitations. Most existing studies are not large-scale RCTs, which are essential for establishing causality and generalizing results. The lack of large-scale RCTs causes a shortage of high-quality studies. Although there is a growing body of evidence suggesting that probiotics and prebiotics can positively influence immune response and modulation of inflammation, much of this evidence comes from preclinical studies or small clinical trials with methodological limitations. Most of the existing studies are not large-scale RCTs, which are essential for establishing causality and generalizing results. On the other hand, to date, have limited sample sizes, which reduces statistical power and the ability to detect significant effects. This also makes it difficult to extrapolate results to larger populations.
The available trials show great variability in terms of dosage, probiotic strains used, duration of treatment, and inclusion criteria, which complicates direct comparison between studies and the formulation of standardized clinical recommendations.
Social implications
The societal implications of using probiotics and prebiotics in treating COVID-19 include maintaining a healthy gut microbiome, which strengthens the immune system and improves overall health. Robust scientific evidence is still required to determine the precise benefits regarding COVID-19 treatment. Moreover, the consumption of probiotics and prebiotics may contribute to good intestinal health by preventing cardiovascular diseases, cancer, and obesity, fostering a healthier society. This may have implications for health disparities and access to care, but further studies are needed to understand this relationship better.
Recommendations
Research on the effects of probiotics for treating or aiding recovery from COVID-19 in adults is currently limited, with small study populations likely due to the recent emergence of the infection. Nevertheless, this research has generated valuable insights and sparked interest in future investigations. Notably, identified knowledge gaps arise from the heterogeneity among studies and the diverse probiotic strains examined. To establish the efficacy and safety of probiotics, there is an urgent need for more well-designed, randomized, controlled clinical trials. Some studies, such as [40], have proposed that probiotics may help modulate the immune system, potentially enhancing the response to viruses by maintaining a balanced inflammatory response.
It is crucial that large-scale RCTs with rigorous methodological designs be conducted to definitively evaluate the efficacy and safety of probiotics and prebiotics in the treatment of COVID-19. These trials should include a diverse population, have adequate sample size, and follow international standards for the evaluation of medical treatments. Future studies should implement strategies to minimize selection, confounding, and publication bias. This includes pre-registration of studies, use of intention-to-treat analysis, and publication of all results, regardless of their nature. In addition to RCTs, more mechanistic research is needed to understand how and why probiotics and prebiotics might influence the progression of COVID-19. This could involve in vitro and in vivo studies, as well as omics analyses to explore the interactions between microbiota, derived metabolites, and the immune response in COVID-19.
Conclusions
In conclusion, the research highlights the potential benefits of probiotics in both treating and preventing COVID-19 and related respiratory infections. Probiotics and prebiotics may reduce symptom severity by restoring gut microbiota balance, modulating the immune response, and improving overall patient outcomes. These findings suggest that probiotics could serve as a valuable adjunctive therapy for COVID-19, particularly in mitigating the inflammatory response and enhancing immune function. However, this area remains under further investigation.
Furthermore, the potential benefits of probiotics in preventing respiratory infections, including COVID-19, provide a rationale for their use in public health interventions. Implementing probiotics as part of preventive strategies, such as supplementation in high-risk populations or integration into dietary guidelines, shows promise in reducing the incidence and alleviating symptoms of respiratory infections.
On the economic front, incorporating probiotics into COVID-19 management and prevention strategies warrants consideration. While the initial investment in probiotic supplements may be significant, their use in immunosuppressed patients remains a key consideration. Enhancing immune function and reducing infection risk through probiotics could yield economic benefits, such as increased labor productivity.
Despite supporting evidence for probiotics in COVID-19 management, these findings should be approached with caution due to variability in probiotic strains, dosages, patient populations, and study designs. Safety is also a concern, as adverse events have been reported. The efficacy and benefits of probiotics must be weighed against the potential for side effects.
Finally, the impact of probiotics on gut microbiota may be transient, with some trials failing to demonstrate significant alterations in the gut microbiome. A longer duration of treatment may be necessary for therapeutic effects. Additionally, there is a lack of systematic assessment of adverse events in probiotic trials, complicating safety evaluations. Despite the abundance of publications, the current literature is not yet adequately equipped to address safety concerns with certainty.
Abbreviations
ACE2: | angiotensin-converting enzyme 2 |
COVID-19: | coronavirus disease |
GRAS: | generally recognized as safe |
LAB: | lactic acid bacteria |
RCT: | randomized controlled trial |
Declarations
Acknowledgments
The authors would like to thank the student Patricia Boziquian for her support in carrying out this research through Primary Information Search.
Author contributions
NASL: Conceptualization, Investigation, Writing—original draft, Writing—review & editing, Methodology. YRMP: Validation, Writing—review & editing, Supervision. TGCC: Investigation, Writing—review & editing. JCM: Writing—review & editing. All authors read and approved the submitted version.
Conflicts of interest
The authors declare that they have no conflicts of interest.
Ethical approval
Not applicable.
Consent to participate
Not applicable.
Consent to publication
Not applicable.
Availability of data and materials
Not applicable.
Funding
Not applicable.
Copyright
© The Author(s) 2025.
Publisher’s note
Open Exploration maintains a neutral stance on jurisdictional claims in published institutional affiliations and maps. All opinions expressed in this article are the personal views of the author(s) and do not represent the stance of the editorial team or the publisher.