Abstract
As the average lifespan has increased, memory disorders have become a more pressing public health concern. However, dementia in the elderly population is often neglected in light of other health priorities. Therefore, expanding the knowledge surrounding the pathology of dementia will allow more informed decision-making regarding treatment within elderly and older adult populations. An important emerging avenue in dementia research is understanding the vascular contributors to dementia. This review summarizes potential causes of vascular cognitive impairment like stroke, microinfarction, hypertension, atherosclerosis, blood-brain barrier dysfunction, and cerebral amyloid angiopathy. Also, this review address treatments that target these vascular impairments that also show promising results in reducing patient’s risk for and experience of dementia.
Keywords
Dementia, vascular contributors, microinfarction, amyloidIntroduction
Amongst the world’s aging population, the prevalence of dementia has rapidly increased in recent decades [1]. While each of the four types of dementia is defined by unique degenerative pathologies, all these conditions also have some cerebrovascular difficulties associated with their progression [1]. It is crucial that the cerebrovascular system is functional in order to maintain cerebral blood flow and cerebral autoregulation [2]. The vascular system is the brain’s primary supply of oxygen and energy substrates. Therefore, this review aims to understand how the degeneration of the cerebrovascular system contributes to dementia pathology. The cerebrovascular system is comprised of all the veins and arteries that transport blood towards the brain and back to the heart [3]. The blood-brain barrier (BBB) tightly regulates the movement of substances in and out of the microvasculature of the central nervous system. The endothelial cells that make up the BBB prevent toxins, pathogens, and other harmful substances from entering the delicate central nervous system [4].
Research has shown that systemic and cerebral cardiovascular dysfunctions like stroke, microinfarction, venous damage, BBB dysfunction, and age-related vascular changes are contributing mechanisms to all forms of dementia [1]. Additionally, acute events like traumatic brain injury (TBI) can also present as a risk factor for dementia as these events often initiate cerebrovascular pathology. Preventing the exacerbation of vascular risk factors now associated with dementia and related memory disorders requires greater attention and investigation [5]. Both preventive treatments traditionally reserved for cardiovascular patients and emerging pharmaceutical treatments are being investigated to help patients at risk of cardiovascular-originating cognitive dysfunction that would lead to dementia. In the following sections, we provide an overview of the four identified types of dementia: Alzheimer’s disease (AD), vascular dementia (VaD), dementia with Lewy bodies (DLB), and frontotemporal dementia (FTD). Next, we offer discussion of vascular mechanisms, including stroke, microinfarction, hypertension, and other age-related cardiovascular dysfunctions, as well as the way they contribute to the development of dementia (Figure 1). Finally, we highlight emerging preventative and treatment approaches related to the denoted cardiovascular contributors to dementia.
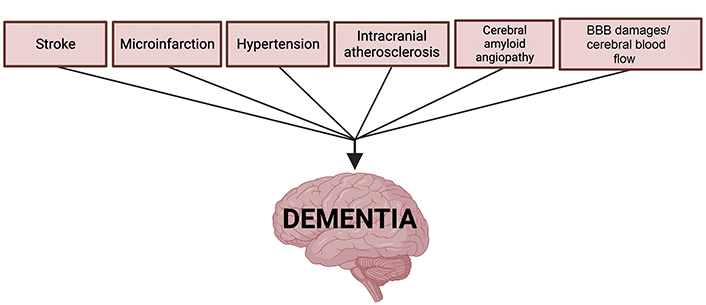
Overview of dementia
AD
AD is a chronic neurodegenerative disorder that is characterized by a loss of cognitive function and ability. It is the most common type of dementia, accounting for 60–80% of dementia cases with > 95% of those cases being sporadic or late-onset AD [6]. There are two clinical hallmarks of AD: amyloid plaques—aggregates of amyloid-β (Aβ) peptides outside neurons, and neurofibrillary tangles—clumps of hyperphosphorylated tau proteins within neurons [7]. However, recent studies have raised awareness of the vascular contributors to cognitive impairment and dementia, especially in AD, with the likes of intracranial atherosclerosis, small vessel disease (SVD), and BBB breakdown [8]. Apolipoproteins (APOs), proteins that transport lipids in the bloodstream, are the strongest genetic factor for AD, with the APOE ε4 allele being associated with an estimated 3- to 4-fold increased risk of AD as opposed to other, less prominent risk alleles [9, 10]. APOE ε4 has been linked to BBB dysfunction—in transgenic mice expressing APOE ε4, pericyte degeneration has been observed as well as BBB leakage [11]. Pericytes are cells found along the capillary wall and maintain the BBB by maintaining endothelial junctions [12]. Aβ peptide deposition is also associated with BBB alteration and vascular degeneration by increasing the activity of the semicarbazide-sensitive amine oxidase or vascular adhesion protein-1 (SSAO/VAP-1) enzymes, producing reactive oxygen species, and increasing inflammation [13]. With these biomarkers in mind, we can better understand how vascular contributors are implicated in the pathogenesis of AD.
VaD
VaD refers to dementia associated with cerebrovascular disease which causes an abrupt onset of varying cognitive impairment (depending on which region of the brain is affected) [6]. VaD follows a stepwise course of decline as opposed to a progressive decline in the case of AD [14]. VaD, the second most common cause of dementia, accounting for 5–10% of dementia cases, is more commonly found as a mixed pathology, co-occurring with AD or other types of dementia [6, 14]. The term vascular cognitive impairment (VCI) is used to denote the spectrum of cognitive impairment associated with vascular pathologies, with VaD being the farthest development of VCI [15]. Skrobot et al. [16] identified seven key pathologies as predictors of cognitive impairment: CAA, large infarcts, microinfarcts, lacunar infarcts, myelin loss, arteriolosclerosis, and perivascular space dilation. Vessel diseases such as arteriolosclerosis, atherosclerosis, and CAA are the most common factors associated with VaD [17]. Ischemic lesions because of microinfarctions and large infarctions are frequently associated with cases of VaD. Microinfarcts are present throughout the brain in large numbers, up to thousands [18] and are present in 30% of autopsies of brains with cerebrovascular diseases like VaD [19]. When patients experience cognitive decline before an ischemic stroke, the risk of developing dementia is further increased, with about 30% of patients developing dementia—one-third being AD and two-thirds VaD [5]. Although there is no treatment for VaD yet, managing the comorbidities (atherosclerosis, arteriolosclerosis, etc.) and lifestyle modifications can control the presence of large and SVDs [20, 21].
DLB
DLB is characterized as neurodegeneration caused by the buildup of α-synuclein protein in neural cells. DLB is a subtype of Lewy body dementia, which also includes Parkinson’s disease (PD) and PD dementia (PDD). DLB is the second most prevalent neurodegenerative disease after AD, and it has a greater progression rate [22]. Pope et al. [22] found that the rapid rate of decline could be caused by multiple pathologies, the presence of neuropsychiatric symptoms, or worse neurotransmitter deficit. There are limited DLB biomarkers, making it an identified need and an active area of research [23]. Concomitant cerebrovascular pathology, pathological alteration observed in addition to the hallmark lesions of a brain with a neurodegenerative disease, is found in 50% of autopsy-confirmed DLB cases [24]. It has been suggested that the pathology shows reduced cerebral blood flow and microvessel density associated with a decreased vascular endothelial growth factor [24].
FTD
FTD is a term used to describe a group of neurodegenerative diseases with symptoms related to impairments in behavior, executive function, or language. Based on clinical features, FTD is further split into behavioral variant FTD (bv-FTD) and primary progressive aphasia (PPA). As one of the most prevalent types of dementia in people over the age of 65, FTD is also the most underdiagnosed dementia since its symptoms frequently resemble those of psychiatric manifestations [25]. FTD is characterized by gliosis, neuronal loss, and microvacuolar changes, which occur in the anterior temporal lobe, frontal lobe, insular cortex, and anterior cingulate cortex [25]. Nearly all cases of FTD are caused by either transactive response DNA-binding protein 43 (TDP-43), microtubule-associated protein tau (MAPT), or fused in sarcoma (FUS) protein. For the diagnosis of dementias, biomarkers such as neuroimaging biomarkers, fluid biomarkers, and gene-specific biomarkers are helpful. One neuroimaging biomarker used for FTD called arterial spin labeling (ASL) noninvasively measures brain perfusion [25]. Blood perfusion measured by ASL can identify a decrease in cerebral blood flow in pre-symptomatic individuals who carry the granulin (GRN) precursor or MAPT mutation [25].
Vascular mechanisms
Stroke
Strokes occur when blood supply to the brain tissue is blocked, leading to decreased nutrient and oxygen supply [26]. Stroke is also a well-established risk factor for dementia and is linked to the onset of cognitive impairment [27]. Of the many conditions leading to dementia, stroke is amongst the most common [26]. The incidence of dementia is approximately doubled following a stroke [27]. Several components influence the onset of dementia following stroke including the site of the lesion, severity of the stroke, and white matter changes [5]. The location of the stroke also impacts the onset of dementia, with left-hemisphere strokes having the greatest increase in risk [28]. Important pathophysiological components underlying the onset of dementia include the dysfunction of the neurovascular unit and the regulation of blood flow to the white matter [5]. Strokes result in a complex cascade of events that overall decrease energy due to interrupted blood flow, oxidative stress, microvascular injury, and death of the cells in the affected region [5]. As a result, the tissues undergo repair mechanisms, such as angiogenesis, to reduce damage [5]. However, in aging brains, the implementation of repair mechanisms is reduced, resulting in parenchyma that cannot be repaired and ultimately contributes to cognitive impairment [5]. The impacted area can also become necrotic and due to the decreased energy, launching the apoptotic signaling pathway poses a challenge and increases the risk for dementia [5]. Following stroke, damaged astrocytes and microglia express an attenuated cytokine response. This neuroinflammation and immunodepression are factors worsening dementia onset as they have devastating consequences on cognitive functions [5].
Microinfarction
Microinfarcts are ischemic infarctions resulting in lesions typically less than 1–2 mm in diameter, occurring in both the cortical and subcortical regions of the brain [18]. Microinfarcts are an emerging contributor to deficits in semantic memory, episodic memory, and perceptual speed [29, 30]. In a study investigating the presence of microinfarcts, researchers found that 80% of microinfarcts were found in the gray matter, with 11% occurring in the white matter [30]. Additionally, there is a strong relationship demonstrated between the location of lesions and dementia likelihood. Microinfarcts occurring in the middle frontal, superior temporal, and inferior parietal regions were the ones associated with the onset of dementia [30]. A study has found that the effect of microinfarcts extends beyond the lesion core and potentially impairs the neuronal function of regions approximately 12 times greater than the lesion core [31]. Microinfarcts in both the gray and white matter result in demyelination and damage to the axonal structure by disrupting neighboring fibers [31]. Structural damages, as a result of microinfarcts, include the thinning of dendritic processes, reduced dendritic spine density, morphological changes to astrocytes, enlargement of soma and processes, and increased expression of glial fibrillary acidic protein [31, 32]. This ultimately results in lowered perivascular clearance of Aβ, increasing dementia risk [33].
Hypertension
Hypertension has been shown to play a significant role in the development of dementia as it promotes changes in the brain structure and function [34]. Hypertension is a risk factor for cerebral SVD and stroke, which are risk factors for dementia [35]. Exposure of the small brain vessels to a higher blood pressure leads to microvascular damage, white matter damage, cortical disconnection, and silent lacunae [36]. Hypertension results in inadequate cerebral blood flow, as it alters the cerebral artery structure and function, which is linked to cognitive decline [37]. Hypertension leads to arteriole and capillary loss, which reduces blood flow to the brain [37]. Reduced blood flow leads to chronic hypoperfusion [37]. Damage to the small cerebral arteries can also lead to brain matter atrophy and white matter lesions [36]. Additionally, hypertension plays a role in cognitive impairment and the development of dementia as it impacts white matter hyperintensities (WMH), WMH progression, and cerebral microbleeds [35]. Microhemorrhages, microinfarcts, and white matter disease can result from hypertension and are strongly linked to dementia [38]. These damages to the cerebral microvasculature impair the clearance of pathological Aβ deposition in AD [39]. Breakdown of the BBB is mediated by hypertension through oxidative stress, inflammation, and vasoactive circulating molecules, ultimately resulting in exposure of neurons to cytotoxic molecules that lead to neuronal loss, cognitive decline, and dementia [37]. Hypertension can lead to cerebral autoregulation being disrupted, decreased cerebral perfusion, and can hinder the brain’s capacity to remove Aβ [35]. The small cerebral vessels play a vital role in connecting hypertension to dementia as damage to them from hypertension results in alterations of their vascular wall, disrupts the BBB’s permeability, and decreases cerebral perfusion, all of which increases the risk of dementia [40].
Intracranial atherosclerosis
Presence of intracranial atherosclerosis significantly increased the odds of dementia, a leading cause of age-related cognitive impairment [41, 42]. Intracranial atherosclerosis is the buildup of plaque in the arteries that supply the brain with blood, causing narrowing and blockage of cerebral blood vessels [43]. These vessels contribute to brain health by delivering nutrients and oxygen and playing a role in trophic signaling that links neuronal and glial well-being to cerebrovascular cells [41]. The narrowing and blockage of these vessels puts the health of the brain and neuronal tissue at risk by increasing the probability of developing vessel tears and hemorrhage [44]. Blockage of vessels, narrowing of vessels, and hemorrhage reduce vessel blood flow to brain tissue, potentially leading to cerebral hypoxia [45]. Chronic hypoxia activates microglia, increases reactive oxygen species, and pro-inflammatory cytokines which induces neuroinflammation, features common to various degenerative central nervous system disorders, including dementia [46].
BBB damages/cerebral blood flow
BBB is responsible for maintaining the central nervous system homeostasis by protecting the brain from unwanted compounds and foreign compounds; its impairment has been linked to various neurodegenerative conditions. Following conditions like stroke, hypoxia damages of the BBB can lead to cytotoxic edema and increased permeability which are exacerbated by neuroinflammatory responses to injury [47]. Dysfunction and damage to the BBB triggers neuroinflammation and oxidative stress which enhances the activity of beta-secretase and gamma-secretase, resulting in the promotion of Aβ proteins [48]. BBB dysfunction also impairs normal transport of Aβ proteins which results in abnormal Aβ accumulation. Normal Aβ transport through BBB utilizes transporters like low-density lipoprotein receptor-related protein-1 (LRP1), receptor for advanced glycation end (RAGE) products, and P-glycoprotein (P-gp). During the development of dementia, these transporters exhibit abnormal expression and function, resulting in abnormal Aβ transport and deposition which leads to further degradation of BBB [49].
CAA
CAA is the deposition of Aβ peptide within leptomeningial and cortical vessels, likely reflecting an imbalance between Aβ production and clearance. Amyloid buildup stimulates a sequence of changes in the cerebral vascular architecture, leading to a wide variety of neurological events including lobar intracerebral hemorrhage, brain ischemia, and cognitive decline [50]. Perivascular drainage impairment potentially triggers a positive feedback pathway by worsening vascular Aβ accumulation, leading to activation of vascular injury pathways, impaired vascular physiology, and further increased parenchymal and vascular amyloid accumulation [51]. Aβ accumulation is common with aging and is toxic to smooth muscle cells, which can cause damage to blood vessel walls resulting in vascular rupture and intracerebral hemorrhage [8, 52]. CAA might be formed by the leakage of hemolysates into the vessel wall as these components are enriched with Aβ peptide. Blood cells themselves, particularly the platelets, are rich sources of amyloid precursor protein [53]. It has been demonstrated that AD patients show a significantly higher ratio of platelet amyloid protein precursor than healthy adults [53]. The platelet-derived amyloid precursor protein may serve as a diagnostic marker for Alzheimer’s and other dementias in the future as their presence may indicate developing pathologies like CAA [54].
TBI
One of the most established environmental risk factors for late-life dementia is TBI. Epidemiologic studies indicate that TBI in early life is associated with a significant increased risk of late-life dementia [55]. In one prospective study on World War II veterans, those who were hospitalized for severe TBI were 4 times more likely to have dementia than their peers; those with a history of a moderate TBI were twice as likely to have dementia [56]. It’s been demonstrated in rodent models that TBI results in memory deficits [57] and biologically, the immediate upregulation of amyloid precursor protein [58]. In humans, amyloid accumulation is rapid and can be detected in tissue of TBI patients in mere hours after their injury [59]. There are many potential mechanisms behind this amyloid deposition phenomenon seen after TBI including ischemic stress [60], blood flow reduction, metabolic acidosis [61], and others [62]. As discussed throughout this review, amyloid plaques contribute to various cerebrovascular pathologies that are known risk factors for dementia. The neurovascular stress events induced by amyloid deposits could contribute to the dementia seen after TBI. Also, like AD, the cerebral atrophy that follows a TBI incident often disproportionately affects the hippocampus and other structures associated with memory [63].
Other abnormalities
Abnormal glucose, lipids, and homocysteine levels in the blood can lead to notable cerebrovascular abnormalities and contribute to dementia. Glucose dysregulation in later life has already been associated with dementia pathology. Diabetes, especially type 2 diabetes, is a known risk factor for AD [64]. However, higher glucose levels may contribute to microvascular disease of the central nervous system [64, 65]. The products of glucose metabolism known as advanced glycation end (AGE) products are detected in the vascular walls of AD patients. Deposits of these molecules in the cerebrovascular system contribute to macroangiopathy, microangiopathy, and amyloid genesis [66]. This is evidence that cerebral glucose metabolism and the cerebrovascular effects of hyperglycemia are likely connected to dementia pathology. There is evidence that high levels of cholesterol are associated with a higher risk of developing dementia in later life [67, 68]. The main APO in the brain is APOE which is incorporated into particles that supply cholesterol to neurons [69]. Both dementia and CAA are associated with high levels of the APOE4 isoform which form complexes with amyloid plaque. The clearance of these complexes is not as efficient as other APOE isoforms. Therefore, the decreased trans-endothelial clearance may explain the connection between the lipoproteins, cerebrovascular disease, and dementia [69]. The interest in homocysteine as a potential risk factor for dementia began because it has been known to be neurotoxic and associated with cerebrovascular diseases like stroke [70]. Some studies indicate that a high plasma homocysteine level can be an independent risk factor strongly associated with AD and other dementias [71, 72].
Emerging treatment approaches
Factors implicated in the development of cardiovascular disease, such as hypercholesterolemia, atherosclerosis, hyperglycemia, hypertension, decreased physical activity, and increased body weight, have been more frequently witnessed in populations with cognitive impairment deficits, such as dementia, as opposed to their counterparts with an absence of neurodegenerative symptoms [73, 74]. Despite these previously founded observations, current treatments for patients with cognitive impairment-related diseases have primarily focused on disease-modifying techniques, often disregarding the underlying, crucial role that cardiovascular health may have on this affected population.
Exercise
A reemergence of novel data linking individuals with cardiovascular disease as being more prone to developing cognitive impairment-related disorders has reinvigorated the neuroscientific field [75]. Emerging research with an approach to ameliorating the implicated factors of cardiovascular disease and preventing stroke often highlights the positive results obtained from a regimen that incorporates exercise and dietary modifications [76]. The steps outlined by the Institute of Medicine to reduce the likelihood of manifesting cognitive impairment deficits emphasize the importance of physical activity. Generally, exercise is hypothesized to increase neurovascular performance, cerebral perfusion, and neuroplasticity, all while ameliorating stress-induced neurodegeneration [76]. The American Heart Association recommends approximately 30 min of moderate physical activity, incorporating a mixture of aerobic or anaerobic exercises [73]. Current studies are already showing promising results: high-intensity exercises show approximately a 64% reduction in dementia progression amongst participants [77]. Similarly, some analyses draw attention to the importance of behavioral therapy, both in the context of exercise and cognitive training as treatments for dementia [78, 79]. In other words, exercise in the context of neurological training has also been identified as being crucial to the production of neurotrophins and insulin-like growth factor 1, which further promote cerebral blood flow [78, 79]. Comparably, the Institute of Medicine further spotlights the importance of remaining socially and intellectually engaged, especially for patients with dementia [75, 80]. Together, however, cognitive and physical exercise appears to exhibit an even larger effect than the sole performance of either individual task (Figure 2) [79].
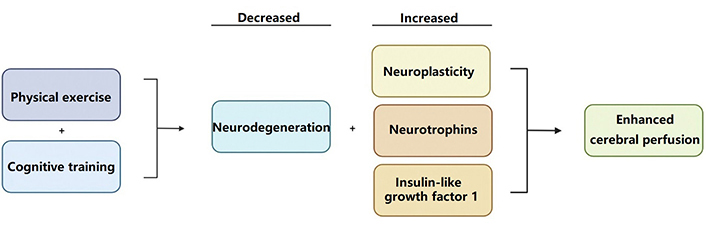
Physical exercise is hypothesized to increase cerebral perfusion; all while decreasing stress-induced neurodegeneration and increasing the production of protective neurotrophins and insulin-like growth factors [76]
Diet modification
As part of a united effort to reduce the risk of dementia, the Alzheimer’s Association further believes sufficient strong evidence has been obtained to conclude that a healthy diet may also reduce the risk of cognitive deficits, such as dementia [80]. Researchers note the overlap between obesity and dementia as significantly considerable [81]. An ineffective diet, such as one that predisposes individuals to higher body mass, is seen to probe the human body into an inflammatory state that can lead to insulin resistance and overall decreased blood flow [81]. On the opposing side, a diet rich in antioxidants, such as vitamin E specifically, has been demonstrated to lower the risk of cognitive impairment deficits when taken orally or through dietary supplements [73]. Furthermore, the incorporation of polyunsaturated fatty acids as part of one’s diet due to their anti-inflammatory and antioxidant capabilities, as well as the observation that they constitute a substantial portion of the brain’s phospholipid membrane and are essential for neuronal activity [73]. An essential part of an individual’s nutritional regimen takes into consideration the inclusion of dietary metabolism, such as one that is influenced by the presence of ketone bodies as an effect of intermittent fasting [82]. The phenomenon that patients with mild dementia have reaped the benefits of fasting increased blood flow was observed after exposure to caprylidene, a ketogenic precursor [82, 83]. Overall, however, it is important to note that the targeting of dietary modifications can prove to be a challenging task as dietary patterns often vary with lifestyle factors, such as socioeconomic status and cultural influences [81].
Pharmaceutical interventions
Sufficient evidence has linked vascular health to cognitive health, brain health, and dementia [84]. Amongst vascular diseases, hypertension has been linked to dementia [85, 86]. An initiative to repurpose antihypertensive drugs to specifically treat dementia yielded inconclusive results [85]. However, a subsequent study of patients with systolic hypertension displayed a reduced risk of developing dementia by 55% after taking antihypertensives for a long-term duration [86]. Contrary to these results, a study posited that removing antihypertensive medication could decrease dementia due to increased brain perfusion. In addition to increased patient health risks, their results were inconclusive [87].
Patients with VCI originating from cerebrovascular disease are known to develop symptoms ranging from mild cognitive impairment to dementia [88]. Because patients with dementia and less severe VCI share reduced cholinergic neurotransmission, cholinesterase inhibitors (donepezil, galantamine, and rivastigmine) were used as treatment for patients experiencing forms of VCI [88, 89]. Although the patient results were not definitive, cholinesterase inhibitor donepezil displayed the most beneficial cognitive effects in VCI and dementia patients [88, 89].
Aggregation of Aβ at the BBB can lead to the cognitive impairment seen in AD [48]. Due to the highly selective semi-permeability of BBB, magnetic resonance-guided focused ultrasound has been hypothesized to briefly separate BBB and remove Aβ [90]. SVD is responsible for a myriad of diseases; however, it is the most common cause of VaD (Figure 3). Although there are no established treatments for SVD, lowering a patient’s blood pressure is a promising preventative measure [91]. Lastly, in conjunction with vascular health, statins have been implicated as a prophylactic treatment. However, additional research is necessary to better develop the use of statins in dementia [92].
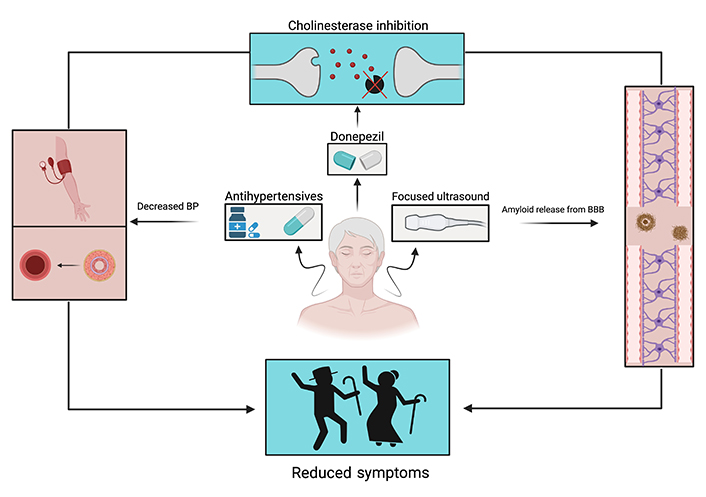
Conclusions
There is much evidence to suggest a strong connection between cardiovascular dysfunction and dementia pathology. AD, the most common form of dementia, is associated with SVD and BBB breakdown. VaD associated with cerebrovascular disease and patients with DLB often show decreased micro-vessel density and blood flow. Finally, individuals with genetic predisposition to FTD also often show a decrease in cerebral blood flow. The mechanisms that result in an inadequate relationship between cerebral functioning and blood supply include events like stroke, microinfarctions, hypertension, atherosclerosis, and CAA. Since this relationship between the neural and cardiovascular circuits is somewhat circular, it can be difficult to distinguish cause and effect, this is still an important point of exploration in the field of dementia research. Through an increase in our understanding of brain pathophysiology and emerging holistic treatments, one step at a time can be taken towards addressing the vascular profile of dementia.
Abbreviations
AD: |
Alzheimer’s disease |
APOs: |
apolipoproteins |
Aβ: |
amyloid-β |
BBB: |
blood-brain barrier |
CAA: |
cerebral amyloid angiopathy |
DLB: |
dementia with Lewy bodies |
FTD: |
frontotemporal dementia |
SVD: |
small vessel disease |
TBI: |
traumatic brain injury |
VaD: |
vascular dementia |
VCI: |
vascular cognitive impairment |
Declarations
Author contributions
CGD: Writing—original draft, Writing—review & editing, Supervision, Conceptualization, Visualization. SJW, SM, and DBV: Investigation, Writing—original draft, Visualization. DF, IK, and AM: Investigation, Writing—original draft. BLW: Supervision, Conceptualization.
Conflicts of interest
The authors declare that they have no conflicts of interest.
Ethical approval
Not applicable.
Consent to participate
Not applicable.
Consent to publication
Not applicable.
Availability of data and materials
Not applicable.
Funding
Not applicable.
Copyright
© The Author(s) 2023.