Abstract
Acquired immunodeficiency syndrome (AIDS) is a global disease caused by human immunodeficiency virus (HIV). About 50 million people have died worldwide due to HIV-1 infection alone. HIV is a primary sexually transmitted infection but can also spread via breastfeeding, blood transfer, organ transfer, etc. Early detection with the maintenance of the disease is the only way to reduce the spread and severity of the disease. There are many conventional techniques for the detection of the virus. Still, recently nano-based diagnostic method remains a little ahead of these techniques due to advancements in nanotechnology. Nanomaterial-based biosensors constitute a significant part of the discussion because of their high sensitivity and accuracy. Nanobiosensors like electronic nano biosensors, quantum dot (QD)-based biosensors, optical biosensors, electronic biosensors, electrochemiluminescence nanosensors, field-effect transistor (FET) biosensors, surface acoustic wave (SAW) biosensors, graphene-based biosensors, etc. have been widely used for detecting HIV in human blood samples. All these biosensors offer promising results in the detection of the virus. In this article, different types of nanobiosensors and their application in the field of diagnosis and maintenance of HIV was reviewed.
Keywords
Biosensors, nanobiosensors, types of biosensors, applications of biosensors, pathogen detection by biosensors, human immunodeficiency virusIntroduction
Human immunodeficiency virus (HIV) is a global threat causing microorganisms, and a vast population of people gets infected by this virus. HIV type 1 alone has caused over 50 million deaths worldwide [1]. HIV causes acquired immune deficiency syndrome (AIDS), which is a burden primarily to low and middle-income countries. In 2016 alone, 1 million people died due to AIDS, and about 1.8 million people got infected with new AIDS-related infections [2]. Lack of awareness about the disease is a significant factor in spreading HIV disorders, and many such studies have been done to counsel the patients and explain the relationship of HIV with peripheral nerve lymphoma [3, 4]. According to studies, about 60–80% of HIV infections were transferred from infected individuals who were not aware of their conditions [5]. In 2018, only 79% of the infected population was aware of their disease status, and more than 5 million people were having no idea about their conditions, and hence they remained undiagnosed [5].
HIV is a retrovirus, and it infects CD4+ lymphocytes. AIDS will develop when CD4 lymphocytes undergo apoptosis, and apoptosis of CD4 lymphocytes occurs when HIV starts replicating. HIV spreads via many modes like sexual intercourse, organ transplantation, breastfeeding, contaminated syringes, and blood transfusion. Inside the host, HIV uses the cell-to-cell mode of transmission or cell-free mode of transmission [6]. The schematic diagram in Figure 1 shows the different ways the HIV virus can enter the host cell, replicate and come out to invade the surrounding cells.
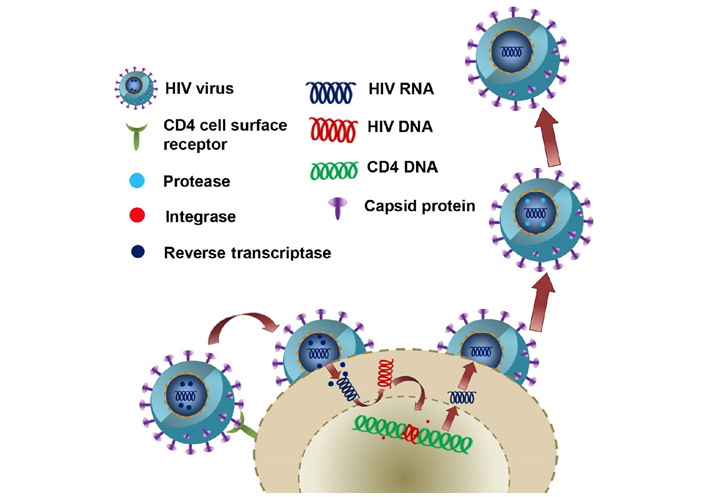
The pathway of HIV entry into the cell, reverse transcription to make HIV DNA from HIV RNA, replication, and packing into the capsid with the help of integrase and release into the surrounding
In medicine, nanotechnology mainly aims at finding solutions for disease diagnosis, treatments, and ways to prevent many diseases [7]. Nanotechnology has profound applications in biosensing, theranostics, targeted drug delivery, nanoformulation of neutraceuticals, medical imaging, etc. [8–19]. Nanobiosensors are sensing devices used to detect various biomolecules in a test sample. The analyte (test component) in a sample will be directly measured using a tool known as a sensor. Nanosensor is an arrangement in which components like gases, electric fields, heat, light, biological components, etc. will be measured using a nanostructure. A biosensor detects explicitly the biological elements like whole cells, DNA, enzymes, peptides, cytokines, proteins, etc. by exploiting different properties of materials like magnetic properties, electrical properties, optical properties, and chemical properties [20, 21].
Nanobiosensors have a receptor, transducer, and detector. The digital output is generated by the detector, and the signal analyzed by the detector will be converted by the transducer (Figure 2). For the amplification of signals, gold nanoparticles (AuNPs), nanofilms, etc., can be used. This will increase the efficiency, sensitivity, and high specificity of the nano-based biosensors [8, 10, 22]. Nanoparticles like platinum, silver, and gold are being used to construct nanomaterial-based biosensors. It also includes the usage of nanowires, nanofilms, and nanorods. These particles are having unique chemical, electrical, mechanical, and optical properties. Because of these properties, they are widely used in nano biosensors [23].
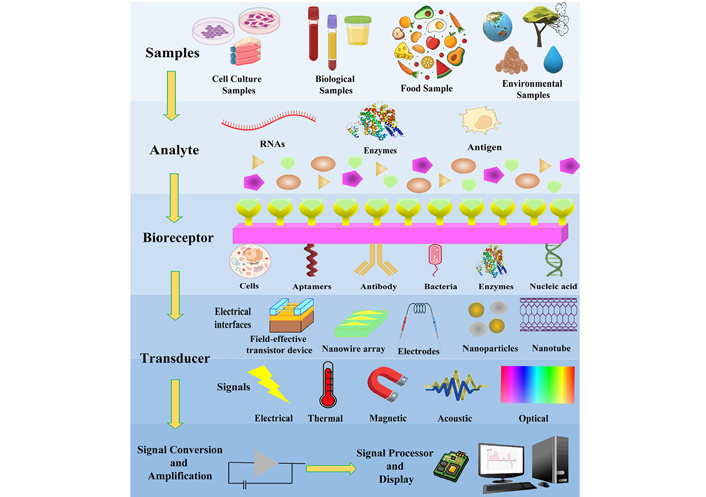
Schematic diagram showing a biosensor. The analytes are taken from the sample which binds specifically to the recognition element. The binding of the analyte with the biorecognition element produces a signal which is converted by a suitable transducer to a measurable parameter and further amplified a readout
Working principle of different types of biosensors
There are different types of nano biosensors classified according to the transducer used.
Electrochemical biosensors
Electrochemical biosensors utilize bioelectrochemistry phenomena to produce a measurable current (amperometric), a quantifiable potential (potentiometric), or a measurable change in the conductivity of the medium (conductometric). There are two other electrochemical methods of detection: impedimetric, which measures resistance and conductance, and field-effect, which measures current using the principle of transistors generated by potentiometric effects. An electrochemical sensor typically requires three electrodes: a reference electrode, an auxiliary or counter electrode, and a working electrode. Silver/silver chloride (Ag/AgCl) is the most commonly used reference electrode, which should be kept a little away from the reaction site. In contrast, the auxiliary or counter electrode created a connection with the electrolyte solution to facilitate the flow of current to the working electrode, while the sensing or working electrode acts as a transducer of the biochemical reactions [8].
Optical biosensors
By interacting with the biorecognition element, optical biosensors detect analytes. Label-free detection and label-based detection are the two modes of optical biosensing. The signal to be detected in label-free detection is produced directly by the analyte interacting with the transducer, while in label-based detection, a label is used, and an optical signal is generated further by fluorescence, colorimetry, or luminescence. There are several levels of optical biosensors for biorecognition, including surface plasmon resonance (SPR), interferometers, localized SPR, gratings, resonators, and refractometers. Any optical biosensor must generate a signal proportional to the concentration of the analyte [8].
Piezoelectric biosensors
When mechanical stress is applied to a material, it produces voltage, which is known as the piezoelectric effect or piezoelectricity. The same phenomenon can be applied in the opposite situation, i.e., alternating voltages can cause mechanical stress or crystal oscillation when they are applied to a piezoelectric material’s surface. Piezoelectricity is exerted by crystals that are anisotropic, that is, do not possess a center of symmetry. There are a number of common materials that serve as piezoelectric materials, including zinc oxide, aluminum phosphate (berlinite), aluminum nitride, crystalized topaz [Al2SiO4(F,OH)2], barium, lead titanate, gallium orthophosphate, quartz (SiO2), tartrate tetrahydrate (Rochelle salt), polyvinylidene fluoride, and polylactic acids. Piezoelectric biosensors are excited by alternating voltage exerted on their surfaces through two electrodes. When incorporated into the oscillation circuit, the alternating voltage induces mechanical oscillations in the crystal, which can be recorded. As a result of analytes or masses bound to crystal surfaces or to electrode surfaces placed on crystals, the frequency of oscillation changes [8].
Thermometric biosensors
In all biological reactions, heat is absorbed or evolved, making it the basis of thermometric biosensors. As a result of a biochemical reaction, heat evolves or absorbs proportionally to molar enthalpy and, ultimately to the number of molecules. Thus, all the changes in enthalpy of the reaction mixture are multiplied to determine the amount of heat generated or absorbed. This heat evolved can be measured using calorimetry [8].
Magnetic biosensors
Magnetic biosensors detect biological interactions using paramagnetic and superparamagnetic nanoparticles. Magnetic effects in biological systems can be measured by changes in coil, magneto-optical properties, and resistance in biological systems. Surface functionalization of magnetic particles is achieved by coating them with antibodies or nucleic acids. The physical properties of the detected particles can be altered when these biorecognition elements interact with the target. Various optical instruments and imaging techniques can be used to detect the particles, including induction coils, Hall effect devices, and giant magnetoresistive (GMR) devices [8].
Nanobiosensors for detecting HIV
The spread of HIV can be prevented by a timely diagnosis of the infection. Conventional methods for detecting HIV in the body includes enzyme-linked immunosorbent assay (ELISA) and polymerized chain reaction (PCR). A gold standard-based method used is usually nucleic acid amplification tests (NAATs) to detect the minute concentration of the virus in the blood. But all these methods come across various challenges. For overcoming these challenges, nano biosensors were developed [24], and some of the major nano biosensors used for HIV detection are discussed below.
Quantum dot-based biosensors
Quantum dot (QD) is a semiconducting nanocrystal and is also called a zero-dimensional nanomaterial. These crystals have been widely used in the field of biosensing and bioimaging. This is due to QDs’ unique properties like intriguing luminescence because of their smaller size and quantum effect, and size-dependent emission property acquired by the quantum confinement effect. They have excellent photostability against photobleaching and blinking. Luminescent QDs facilitate rapid and selective detection of viruses. So, these are used in the early detection of many viruses including HIV [22].
Deng et al. [25] developed fluorescent QDs with testing strips in combination with strand displacement amplification (SDA) for the early diagnosis of AIDS. This assay had a low detection limit of 0.76 pmol/L, and the range of detection was between 1 pmol/L and 10 nmol/L. Signal intensity in the test zone was a major factor in the working of this system. QD has the potential to assemble more biomolecules and thus can increase the accuracy and sensitivity of strips. HIV-DNA quantification was done with the aid of SDA. HIV-DNA was recycled many times, which resulted in the formation of many QD double-stranded DNA (dsDNA). These nanostructures were later captured by test zones, which increased the signal intensity of the zone. In this assay, when target HIV-DNA was detected, both the test and control zones will exhibit a signal which indicated a positive test. In the negative test, only the control zone showed fluorescence signals [25]. Wang et al. [26] in their study designed a QD-based photoelectrochemical biosensor for detecting the HIV-1 gene. In this platform, they cascaded photoactive interphase, in which cadmium telluride (CdTe) QDs and zinc oxide nanorods (ZnO NRs) were used to achieve amplification of the signals. This biosensor’s detection limit was as low as 0.65 fmol/L and from the serum sample, from which about 97.6–101.8% of HIV-1 was recovered. It also showed a detection range between 1fmol/L–1nmol/L [26].
Electronic nanobiosensors
Electronic nano biosensors can be used for the early diagnosis of an HIV gene, and it is having a relatively low cost. These biosensors have a transducer that is developed from a modified commercially available electrode structure and have the potential to detect HIV-DNA using a resistive measurement technique. Recently in nano biosensing devices, an electrochemical transducer also was used. These transducers worked based on an electrochemical reaction taking place inside the electrochemical cell. It consisted of three types of electrodes namely—working, reference, and counter electrodes. This system identified and detected the target DNA bound to the biorecognition element. Another transducer, known as, an integrated electrode-based interdigitated electrode (IDE) transducer, was developed and was believed to be a future tool for the early diagnosis of HIV in a resource-limited environment. This setup included a tunable nanoparticle size and in-between distribution among the IDE. This combination is believed to improve the overall sensitivity of the system [27].
For the electronic detection of HIV antibodies, Morin et al. [28] developed a disposable nanopore test strip and a portable reader device, using which clinically relevant HIV antibody detection in the concentration range of 0.4–20 mg/L was possible within 60 s, from saliva. Macchia et al. [29] constructed a large field single-molecule transistor (SiMoT) sensor for detecting HIV-1 P24 capsid protein, which can detect concentrations as low as 2 ± 1 proteins per mL, which is equal to 3 × 10–11 nmol/L.
Electrochemiluminescence sensors
Highly specific and sensitive HIV-1 genes can be detected using an electrochemiluminescence sensor. For this purpose, Babamiri et al. [30] designed a novel molecular imprinted polymer-electrochemiluminescence (MIP-ECL) sensor. In this system, Europium sulfide nanocrystals (EsNCs) were used as the signal-producing compounds. The template used was HIV aptamer, and o-phenylenedyamine was the functional monomer. Both template and monomer were electropolymerized directly on to indium tin oxide (ITO) electrode surface. EsNCs functionalized 5-amino-labeled oligonucleotides assembled as the captured probes, which were hybridized with oligonucleotides as detecting targets (HIV-1). The ECL signal was effectively increased with the aid of potassium persulfate (K2S2O8) as a co-reactor. EsNCs exhibited a strong electrochemiluminescence emission which was used to detect sensitive and highly specific HIV-1 genes present in human serum samples. For other DNA markers detection, EsNCs can also be taken as a luminophore in MIP-ECL sensors. Using this system, HIV gene detection has been achieved in the range of 3.0 fmol/L to 0.3 nmol/L, with 0.3 fmol/L as the detection limit. The relative standard deviation (RSD) and recoveries were in the range of 1.78−4.2% and 95.00−101.2%, respectively [30]. Zhou et al. [31] developed a sandwich-type electro chemiluminescence sensor using the composite P-RGO@Au@Ru-SiO2 developed from Ru(bpy)32+-doped silica (Ru-SiO2) and gold-nanoparticle-decorated graphene (P-RGO@Au), for detecting of HIV-1 P24 antigen. The detection limit of this immunosensor was 1.0 × 10–9 mg/mL, and the linear range came between 1.0 × 10–9 and 1.0 × 10–5 mg/mL [31].
Optical nanosensors
An optical nanosensor is a type of biosensor used for virus detection. It works by measuring the changes in the transducer’s reflective index. There are two types of optical nanosensors: direct optic nanosensors and indirect optic nanosensors. Direct optic nanosensors generated signals due to the production of complexes on the surface of the transducer. Indirect optic nanosensors were designed with different labels to identify the bindings taking place and extend the signal. Indirect optic nanosensors can produce higher signals than direct ones, but they have the drawback of non-specific binding, and the cost of reagent of labelling is high. Optical nanosensors are having multipurpose detection scales, and hence they can measure different biomolecule types from various specimens. So, these sensors can be utilized for the detection of a wide range of viruses [32]. Photonic crystal (PC) nanostructured optical biosensor was used for HIV detection, and it used a 384-well microplate and the substrate involved was elastic polyester. This was a very effective method for HIV diagnosis [21, 32].
Shafiee et al. [33], in their study, developed a PC nanostructured optical biosensor that can perform the detection and quantification of HIV-1 from human biological samples. During illumination with a broad light source, the PC biosensor’s surface reflects resonantly in narrow wavelength bands. Biotarget that was adsorbed to the surface brought out a slight shift in resonant peak wavelength value (PWV), which enabled the detection of various layers of bimolecular viruses and a small number of viruses. This could be used for detecting HIV in serum and phosphate buffered saline (PBS), with a viral load range between 104–108 copies/mL based on the PWV shift. The PWV shift of HIV-1 subtype B samples with viral loads of 105, 106, 107, and 108 copies/mL was found as 85%, 85%, 89%, and 91%, respectively, and for subtype D HIV-1, the PWV shifts were observed as 85%, 93%, 95%, and 98%, respectively [33].
Field-effect transistor biosensors
Field-effect transistor (FET) type biosensors could be utilized for early detection and monitoring of HIV-1. FET biosensor is a liquid-ion gated, label-free biosensor. Nickel oxide (NiO) nanostructure, which is a p-type semiconducting metal, has applications in FET biosensors. This is because of their unique properties like outstanding biocompatibility, higher compatibility with micromachining, excellent electron transporting performance, great electrocatalytic activity, low cost, good sensitivity, and remarkably due to their photonic and tunable size-dependent surfaces. It was the best platform for desired transducers and in the immobilization of molecules like DNA, RNA, enzymes, and antibodies in the design of sensors for increasing sensitivity. NiO thin film and FET structure are the two parts of FET sensor. DNA will be immobilized onto NiO thin film. For the deposition of NiO thin film onto the glass substrate present, a reproducible radio frequency magnetron sputtering technique was used. The hybridization events on NiO film electrodes will be transduced to electric signals by the FET structure. The liquid ion-gated, label-free FET biosensor would precisely detect a low concentration of target HIV DNA in the sample. Using this biosensor, HIV detection was possible at a limit of detection (LOD) of 0.3 amol/L, in a linear range of 1.0 amol/L to10.0 nmol/L. Three different HIV DNA concentrations were added to PBS and a healthy serum sample was taken as the control group for HIV detection. The biosensor showcased excellent sensitivity and a greater percentage of recovery in a real blood sample. The RSD values and recoveries came in the range of 3.5–5.1% and 103.5–108.2 %, respectively [34].
Smartphone based surface acoustic wave biosensors
Smartphone connected surface acoustic wave (SAW) biosensor was developed, and it could rapidly detect HIV infection biomarkers in a blood sample within a few seconds. This system consisted of four disposable SAW biochips, a small control box, and a smartphone. Shear horizontal (SH)-SAW, was generated on the biochip via microfabrication and piezoelectric effect (Figure 3). Each chip consisted of a piezoelectric quartz crystal and IDEs with gold input and output, along with the presence of a sensing area in between. The sensing area was tailored with layers of captured protein and a thin gold film. If the blood sample contained the HIV biomarker, it would bind to the captured proteins on the biochip’s surface. This will cause a change in viscoelasticity and mass, which could be instantly identified by the phase change in SH-SAW [35]. For reading and analyzing the results, disposable SAW biochips were connected to a small-sized control box. For displaying, analyzing, and transmitting the results, a smartphone was used [36].
Turbé et al. [35] developed SAW biochips and the biochip was able to detect HIV antibodies from samples at the amount as low as 50 nmol/L within 10 s. It was also able to detect the p24 antigen which was a major biomarker of HIV infection. For this anti-p24 llama, VHH was coated on to SAW biochips, and the combination of llama VHH ligand and NIH-3537 detection antibody was able to induce a large phase change in response to 4 nmol/L within 20 min. When the background proteins were present in a one-million-fold concentration, the sensitivity of the biosensor for detecting HIV p24 proteins was on a nanomolar scale of 2–40 nmol/L, where 2 nmol/L was the lowest p24 detection concentration [35].
Graphene-based biosensors
Graphene nanoparticles are nowadays widely used in nano-sensor fabrication. They have the ability to enhance signal response in many sensing applications. They are having a large surface area and excellent biocompatibility with a wide range of molecules like DNA, enzymes, antibodies, proteins, and cells. Hence, graphene-based biosensors can detect different biomolecules and even cells. Graphene-based nanomaterials are utilized as a transducer in many biosensors. It can mediate electron transfer between bioreceptors and transducers. Graphene is having high fluorescent quenching efficiency and so can be used to produce fluorescent biosensors. Biosensors having surface-modified graphene with dendrimers have been generated for the early detection of diseases like AIDS/HIV [37]. Gong et al. [38] in his study designed a sensitive and simple DNA biosensor by the modification of glass electrodes using graphene-Nafion composite film for detecting HIV-1 gene and the sensor’s detection limit was found to be 2.3 × 10–14 mol/L (2.3 × 10–5 nmol/L). Wang et al. [39] constructed a graphene oxide (GO) based fluorescence polarization signal amplifier, and using this system, about 38.6 pmol/L HIV DNA was detected.
Some recent development in the detection of the HIV gene was using differential pulse voltammetry (DPV). It is an electrochemical biosensor, where the peak of the DPV current varies for single-stranded DNA (ssDNA) and dsDNA. Using the intrinsic topological insulator (TI) BiSbTeSe2, Jiang et al. [40], have developed a very sensitive, label-free electrochemical biosensor that can be used to determine HIV gene expression. In addition to having stable and sensitive electron transport, TIs with strong spin-orbit coupling may have robust surface states with low electronic noise. A linear relationship was found between the DPV peak currents and target DNA concentrations using BiSbTeSe2 biosensors, which exhibited a detection limit of 1.07 × 10–15 mol/L. DPV peak currents performed well after storage at 4°C for 7 days, and the biosensors had good selectivity and stability. Using topological materials, this study provides an effective method of developing biosensors that can be used for the determination of clinical markers and monitoring [40]. In another similar study by Xiong et al. [41], a large-size Bi2Se3 tape electrode (BTE) was prepared using a single crystal of 2 cm × 1 cm × 0.5 cm. For HIV-1 DNA electrochemical sensing, flexible BTE was explored as an efficient bioplatform for loading Au nanoparticles and probe DNA. Utilizing the dual principles of DPV as well as electrochemical impedance spectroscopy (EIS), the resultant biosensor exhibited a broad linear detection range between 0.1 fmol/L to 1 pmol/L. It also showed a very low LOD (50 amol/L), excellent reproducibility, selectivity, and stability, which was superior compared to the pmol/L DNA level of detection shown by Pt-Au, graphene-AuNPs hybrid biosensors. It was believed that Bi2Se3’s intrinsic surface state facilitates electron transfer due to its outstanding performance. Hence, the BTE electrochemical biosensor platform showed great potential for the detection of DNA biomarkers in a sensitive manner [41]. An innovative approach to testing HIV is self-testing, which allows individuals to collect their own specimens, conduct tests, and interpret the results privately. Using 100 μL finger-prick whole blood, Liu et al. [42], introduced a completely integrated nucleic acid testing (NAT) device. Microfluidic reagent cartridges and ultra-compact NAT-on-universal serial bus (USB) analyzers are included in the device. By dropping the finger-prick sample of blood into a collection tube along with the lysis buffer and loading the lysate on the top of the microfluidic cartridge, users can easily read the test results with a custom-built graphical user interface (GUI). Sample preparation and purification, as well as real-time loop-mediated isothermal amplification (RT-LAMP) is automated by the microfluidic cartridge and analyzer. At a 95% confidence level, they achieved 214 viral RNA copies/mL of whole blood after a turnaround time of 60 min. Their expectation is that the HIV NAT-on-USB device will be particularly useful to high-risk populations seeking private self-tests as early as possible after exposure due to its ease of use and high sensitivity [42].
Other applications of nanobiosensors in medicine
Cancer diagnosis
Ou et al. [43] developed a nanobiosensor using Mn3O4/Pd@Pt/horseradish peroxidase nano-probe that contained human epidermal growth factor receptor-2 (HER-2) for fast and early detection of breast cancer. This nanobiosensor was able to increase the rate of detection of breast cancer in its early stages and had a detection limit of up to 0.08 ng/mL. The sensor had a linear response in the range of 0.1–100.0 ng/mL [43]. Zhao et al. [44] constructed a SPR biosensor by fabricating lactose-functionalized gold nanorods (Lac-GNRs) to detect cancerous conditions. Galectin-1 is an important biomarker of tumor development, and with the aid of this biosensor, detection of Galectin-1 as small as 10–13 mol/L (10–4 nmol/L) was possible [44]. Liu et al. [45] designed an aptamer-AuNP strip biosensor for detecting circulating cancer cells, which reached a detection limit of 800 Ramos cells within 15 min using a portable strip reader.
Diagnosis of coronavirus
Huang et al. [46] developed a fluorescence fibre-optic biosensor that targeted the N-protein of severe acute respiratory syndrome-associated coronavirus (SARS-CoV) and was having a LOD of ∼1 pg/mL, and the linear range came between 0.1 pg/mL and 1 ng/mL. Ishikawa et al. [47] constructed a FET-based In2O3 nanowire with a LOD of 2–10 nmol/L and was having high specificity in the detection of SARS-CoV. Teengam et al. [48] designed a paper-based colorimetric DNA sensor based on silver nanoparticles (AgNPs) that targeted peptide nucleic acids (PNA) probes in Middle East respiratory syndrome coronavirus (MERS-CoV) and exhibited a LOD of 1.53 nmol/L with a linear range between 20–1, 000 nM. Layqah and Eissa [49] in their study performed an electrochemical method based on AuNPs, which targeted the S-protein of human coronavirus and was having high specificity, a LOD of 0.4 pg/mL, and a linear range between 0.001–100 ng/mL.
Diagnosis of other viruses
Manzano et al. [50] in his study developed a label-free electrochemical DNA biosensor based on ssDNA/AuNPs for the detection of hepatitis A virus (HAV) with a LOD of 0.65 pmol/L and a linear range between 10 fg/μL–10 pg/μL. For detecting hepatitis B virus (HBV), Qu et al. [51] constructed a silver nanocluster-MoS2 nanosheet with a LOD of 10.7 nmol/L and a linear range of 5–30 nmol/L. Azab and Fekry [52] developed a carbon nanotube-cobalt NPs-based nanosensor for the detection of HBV, and it exhibited a LOD of 8.82 × 10−10 mol/L and a linear range between 1.0 nmol/L–12 μmol/L. For detecting the Ebola virus, Ilkhani and Farhad [53] designed an electrochemical DNA biosensor based on ssDNA-AuNPs and it had a LOD of 4.7 nM. A FET biosensor based on GO-AuNPs was constructed by Chen et al. [54] for detecting Ebola antigen, and it exhibited a LOD of 1 ng/mL and the linear range came between 1–400 ng/mL. Bartolome et al. [55] developed a DNA sensor which was based on carbon nano-onions for Human papilloma virus oncogene detection and showcased a LOD of 0.5 nmol/L and a linear range of 0.5–20 nmol/L. Uludağ et al. [56] came up with carboxymethyl-dextran polymer sensor chips for detecting herpes simplex virus-1 (HSV-1), and these chips were having a LOD of 5.2 × 10−11 mol/L and a linear range between 5.2 × 10−11–1.3 × 10−7 mol/L. For the detection of the highly pathogenic avian influenza A (H5N1) virus, Wu et al. [57] designed strips based on AuNPs for electrophoresis-enhanced detection and they exhibited a LOD between 0.1–40 ng and a linear range of 0.1–100 ng.
Detection of bacterial pathogen
Starodub et al. [58] constructed high-specific biosensors based on SPR and total internal reflection ellipsometry (TIRE) for detecting Salmonella spp. For SPR biosensors, the sensitivity range was reported within 101–106 cells/mL, whereas TIRE had greater sensitivity and that was up to many cells in 10 mL. This system employed a surface binding layer and Ag-Ab reactions as the reactive part [58]. Vaisocherová-Lísalová et al. [59] used ultra-low fouling and poly (carboxibetaine acrylamide) based SPR biosensor to detect the same species in food. For this biosensor, an average response time of 80 min and a LOD of 7.4 × 103 CFU/mL were reported. In another study, Narmani et al. [60] constructed a AuNPs and magnetic NPs-based ultrasensitive and selective fluorescence DNA biosensor for detecting Vibrio cholera O1 OmpW gene which demonstrated a LOD of 2.34 ng/mL. Ahari et al. [61] designed a potentiometric nano-biosensor for detecting bacteria by means of the exotoxin emitted by them and it was detected that exotoxin density was up to 10–3 mol/L by using this biosensor.
Diagnosis of autoimmune diseases
Fagúndez et al. [62] developed an electrochemical immunosensor in sandwich format for detecting anti-dsDNA autoantibodies in patient’s serum samples. The patient was diagnosed with systemic lupus erythematosus (SLE) and the immunosensor was found to have LOD of 8 μg/mL by means of amperometric detection method. In another study, Li et al. [63] constructed an amperometric nanobiosensor based on AuNPs, titanium dioxide nanoparticles, and thionine (NGP-NTiP-Thi) for detecting macrophage migration inhibitory factor (MIF) in serum samples from patients having rheumatoid arthritis (RA). This sensor showed great specificity toward MIF and was having a LOD of 0.02 ng/mL, and the linear range came between 0.03–230 ng/mL [63]. Gracia Villa et al. [64] also designed an amperometric immunosensor for anti-citrullinated peptide antibodies (ACPAs), which is a major biomarker of RA. Wilson et al. [65] in their study employed a label-free EIS immunosensor for the detection of anti-tissue transglutaminase (anti-tTG) antibodies in serum collected from patients suffering from celiac disease. The system’s efficiency and biocompatibility were increased by using platinum modified polypyrrole-cobalt (II) salicyladiimine metallodendrimer nanocomposite. This platform exhibited a LOD of 201 ng/mL and a linear range of 10−5–10−4 mg/mL [65]. For the detection of anti-tTG antibodies, Singh et al. [66] developed a label-free nanogap-IDE biosensor based on AuNPs, and its dynamic detection range came between 30 pmol/L and 30 nmol/L using EIS. Habtamu et al. [67] also developed ruthenium (Ru)-based ECL immunosensor to detect anti-tTG, and the LOD of this platform was 0.5 ng/mL, with a linear range that was found between 1.5 ng/mL and 10 μg/mL. Derkus et al. [68] in his study developed a label-free electrochemical impedance immunosensor based on titaniumdioxide (TiO2) nanoparticles, for detecting anti-myelin basic protein (anti-MBP) autoantibodies in patients with multiple sclerosis (MS) and this sensor exhibited a LOD of 0.1495 ng/mL and the detection range lied between 0.4875–2, 500 ng/mL.
Conclusions
Nanotechnology is gaining attention in the field of disease diagnosis and treatment due to the advancement and possibilities in the developing era. Recently, nanomaterials have been incorporated into the generation of biosensors because of their unique properties. Nanomaterial-based biosensors, also known as nanobiosensors, can be used to detect various viruses, including HIV, which causes AIDS. Early detection of HIV infection is a way in which the severity and spread of the disease can be reduced and controlled. This mini-review discussed various nanobiosensors like optical biosensors, electrochemical biosensors, QD-based biosensors, etc., and their application in the early detection and maintenance of HIV as well as other applications in medicine.
Due to the availability of modern techniques, HIV biomarkers can be diagnosed with a high degree of accuracy. As a result of these methods, the number of tests conducted on a daily basis has increased globally, providing information about the severity of the infection. Microfluidics and nanotechnology should be used extensively in point of care (PoC) testing to simplify and increase testing. DNA nanobiosensors improved their sensitivity, ease of use, and trace amounts of the sample by applying nanomaterials and nanostructured interfaces. However, some of the proposed methods require amplification techniques in order to detect the results, resulting in longer detection times. Aside from this, the nucleic acid probe structures are used for sensing the need to maintain stability during detection and resist various solution conditions. In order to provide a clever and highly precise design concept assay, further improvements in the protocols are needed. In order to rapidly implement these technologies during HIV screenings, they need to be further developed.
Abbreviations
AIDS: | acquired immunodeficiency syndrome |
anti-tTG: | anti-tissue transglutaminase |
AuNPs: | gold nanoparticles |
BTE: | Bi2Se3 tape electrode |
DPV: | differential pulse voltammetry |
dsDNA: | double-stranded DNA |
EIS: | electrochemical impedance spectroscopy |
EsNCs: | Europium sulfide nanocrystals |
FET: | field-effect transistor |
HIV: | human immunodeficiency virus |
IDE: | interdigitated electrode |
LOD: | limit of detection |
NAT: | nucleic acid testing |
NiO: | nickel oxide |
PC: | photonic crystal |
PWV: | peak wavelength value |
QD: | quantum dot |
SAW: | surface acoustic wave |
SPR: | surface plasmon resonance |
ssDNA: | single-stranded DNA |
Declarations
Acknowledgment
The authors thank the Chettinad Academy of Research and Education for providing the infrastructure. We acknowledge Deepika B for her support in improving the figures.
Author contributions
SM and TJ: data curation, writing–original draft. KG and AG: conceptualization, investigation, visualization, methodology, writing–original draft, project administration, and writing–review & editing.
Conflicts of interest
The authors declare that they have no conflicts of interest.
Ethical approval
Not applicable.
Consent to participate
Not applicable.
Consent to publication
Not applicable.
Availability of data and materials
Not applicable.
Funding
Funding was received from the Council of Scientific and Industrial Research (CSIR), INDIA, for the grant with Scheme No. 012868/17/EMR-II. The funders had a role in data collection and preparation of the manuscript.
Copyright
© The Author(s) 2022.