Abstract
Radiation is a primary therapy in the treatment of thoracic malignancies with clear survival benefits. Consequently, patients with cancer are living longer but may be subject to a wide array of cardiotoxic effects from collateral radiation damage. Ensuing fibrosis can affect any portion of the cardiac parenchyma, increasing the risk for accelerated coronary artery disease, pericardial sequelae such as constrictive pericarditis, valvulopathy, restrictive cardiomyopathy, and a myriad of conduction system abnormalities. Unfortunately, the effects of cardiotoxicity can be subclinical or delayed and there remains an unmet need to standardize management strategies for these patients. Based on current data, it is prudent to consider percutaneous approaches first for coronary and valvular disease and traditional, supportive measures for the remaining sequelae. Every attempt should be made to undergo a complete operative haul due to the increased risks of re-operation if surgery is to be performed. Surrounding the patient with a multidisciplinary heart team is critical.
Keywords
Radiation-induced coronary artery disease, radiation valvulopathy, radiation-induced cardiomyopathyIntroduction
Radiation therapy (RT) is a primary or adjunctive therapy in the treatment of thoracic malignancies (i.e., bronchogenic, breast, esophageal, and lymphoma). With the increasing survival of patients living with cancer, preparing for sequelae of collateral thoracic radiation injury is imperative [1]. Radiation-induced heart disease (RIHD) is one of the most serious complications and comprises a spectrum of cardiotoxicity including coronary artery disease (CAD), pericarditis, valvulopathy, cardiomyopathy, and conduction system abnormalities [2]. Unfortunately, RIHD is often underrecognized by clinicians, since cardiotoxic effects may be subclinical or delayed [3–4]. The purpose of this paper is to fill the knowledge gaps and fulfill the following goals: to review the basic pathophysiology of the key components comprising RIHD (summarized for convenience in Figure 1) and clinical management, as pertinent for internal medicine and cardiovascular disease providers.
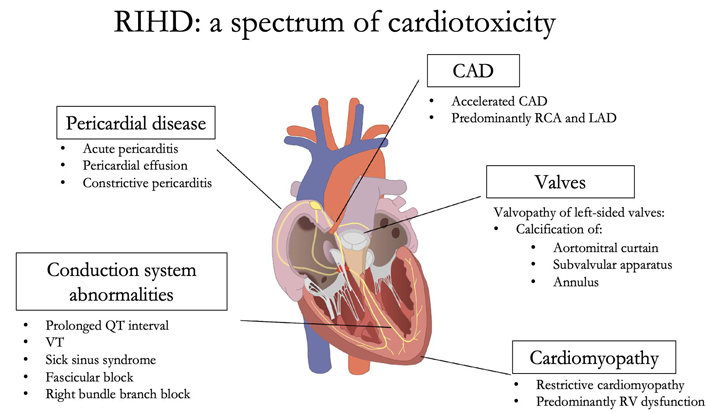
Cardiotoxicity spectrum secondary to RIHD. RCA: right coronary artery; LAD: left anterior descending; VT: ventricular tachycardia; RV: right ventricle
Recognition and management of CAD in RIHD
Cardiac cells display differing sensitivities to radiation. Cardiomyocytes are terminally differentiated, making them relatively resistant to the effects of radiation. On the other hand, coronary endothelial cells are highly radiosensitive [5]. Endothelial cell injury is currently believed to be the falling domino that contributes to the myriad of subsequent cardiotoxic effects [6].
During radiotherapy, reactive oxygen species are generated by the radiolysis of intracellular water. The oxygen radicals liberate nuclear factor kappa β (NF-κβ) from its inhibitory subunit, triggering a reaction cascade that terminates with increased pro-inflammatory gene expression [7]. NF-κβ additionally serves as the bridge for upregulating selectin adhesion molecules, which anchor migrating leukocytes to the site of injury. Recruited leukocytes secrete proinflammatory cytokines, perpetuating the acute phase response [8]. Following this acute phase, a quiescent period ensues, with no obvious microscopic tissue changes. Frequent radiation insult is a powerful initiator for the chronic, fibrotic period. This phase is dominated by transforming growth factor-beta 1 (TGF-β1), which suppresses collagenase activity and induces fibroblast differentiation into myofibroblasts, thereby intensifying fibrosis within the subendothelial spaces [9]. The end result includes stenotic coronary vessels with endothelial effacement, causing accelerated CAD [10].
Angiographically, lesions associated with radiation-induced CAD (RICAD) occur commonly at the ostia of the epicardial coronary arteries. There is a greater propensity for RCA and LAD arterial involvement due to their anterior positions within the radiation field. Lesions also tend to be larger and more tenacious when compared to non-irradiated controls [11]. In patients with obstructive RICAD, the decision to pursue coronary artery bypass grafting (CABG) over percutaneous coronary intervention (PCI) remains contentious. CABG poses challenges, since patients may be high-risk surgical candidates. Friable thoracic vessels increase bleeding risk and portend poor surgical site healing [12]. Patients may also have concomitant interstitial lung disease, disallowing safe extubation [13]. Mediastinal fibrosis poses technical challenges to the surgeon for field access. Arterial and venous conduits may be scarred, stenosed, or atretic from radiation damage [14]. Lastly, extensive circumferential calcification of the thoracic aorta precludes safe aortic clamping for cardiopulmonary bypass [15]. Despite these barriers, Dunn et al. [16] found evidence that surgery may have value. In their observational study, patients with RICAD who underwent CABG had no difference in long-term mortality and even lower major adverse cardiac events compared to their counterparts who underwent PCI, despite similarly complex coronary lesions [16]. One plausible explanation is that RICAD disproportionately affects the proximal coronaries, a factor associated with poorer outcomes in patients following PCI, compared to those with proximal disease undergoing CABG. For now, patients should be evaluated on a case-by-case basis. The American and European guidelines recommend a multidisciplinary heart-team approach, which focuses on the complexity of mediastinal anatomy, comorbidities, and the experience of both the interventionist and cardiothoracic surgeon [3]. Most importantly, the patient remains at the center of discussion at all times, with their values and personal preferences prioritized.
Recognition and management of pericardial sequelae in RIHD
Pericardial disease is a common manifestation of RIHD and more frequently occurs when greater than 30% of the cardiac volume is exposed to a minimum dose of 50 Gy [17]. A previous necropsy study reported that 70% of patients with RIHD had some degree of pericardial involvement, typically effusion or constriction [18]. Reassuringly, incident rates have decreased because of improved radiation precision, lower radiation doses, subcarinal blocking, equal weighting of anterior and posterior fields, and newer techniques involving proton therapy [19–20]. Recent findings also suggest that free breathing with voluntary deep inspiration breath holding can significantly reduce cardiac radiation exposure [21]. The contouring of cardiac substructures has additionally allowed for improved cardiac sparing [22]. Despite these advances, the clinician must still be prepared to diagnose and manage the pericardial sequelae of RIHD, which includes acute pericarditis, chronic pericardial effusion, and constrictive pericarditis (CP).
Acute pericarditis from radiotherapy is rare and occurs as a reaction to radiation damage and adjacent tumors with high necrosis burden, especially lymphomas [23]. The disorder is self-limiting, with 50% of patients not requiring intervention [17]. The remaining patients are conservatively managed with two weeks of a non-steroidal anti-inflammatory drug or high-dose aspirin, in conjunction with 3 months of colchicine. Due to the benign course and risk of tumor recurrence, radiotherapy should not be withheld in acute pericarditis [24].
Pericardial effusions can present months to years following high doses of radiotherapy [25]. The mechanism centers around radiation-induced microvascular injury, resulting in increased capillary permeability and exudation of protein-rich fluid. Impaired lymphatic drainage to the mediastinal and tracheobronchial lymph nodes from local fibrosis also contributes to fluid accumulation [26]. The median onset appears to be 6 months, with 91% of patients reporting no symptoms [25]. Patients within this category may be managed with serial echocardiograms. Symptomatic pericardial effusions devoid of tamponade physiology can be treated non-urgently using echocardiography with or without fluoroscopy-guided pericardiocentesis to confirm treatment response. It is prudent to leave an insignificant amount of fluid to avoid pericardial decompression syndrome [27]. An intrapericardial catheter is secured afterward and left in place until drainage rates are < 15–20 mL over 24 h to allow adherence between the visceral and parietal layers [28]. Patients with tamponade physiology should undergo emergent pericardiocentesis through echocardiographic or electrocardiographic guidance. The blind approach is associated with unacceptably high morbidity and mortality but is necessary in the patient with extremis if the previously mentioned monitoring approaches are not feasible [29].
CP is the end product of irreparable pericardial damage. Repeated radiation can result in a cellular milieu rich in TGF-β1, leading to terminal differentiation of fibroblasts and consequently, a several-fold increase in interstitial collagen deposition [30]. These microcellular sequences account for the fibrotic, non-compliant pericardium that can cripple diastolic function and lead to secondary heart failure with preserved ejection fraction (HFpEF). Constrictive changes typically occur following a latency period of 10 or more years, with future risk ranging as high as 20% if a patient sustained an initial episode of radiation-induced acute pericarditis [31]. Therefore, the clinician should retain a low degree of suspicion for constrictive physiology in patients with a history of thoracic RT presenting with new or recalcitrant heart failure. Symptomatic management of CP is achieved with diuretics. Sodium-glucose cotransporter 2 (SGLT-2) inhibitors are the only drug class with a potential mortality benefit in the HFpEF studies [32]. Thus, there may be a utility of these agents in the subset of CP patients with fibrotic extension into the endomyocardium. Unfortunately, there is inadequate representation of CP in these trials for there to be consensus. Pericardial stripping and pericardiectomy remain the cornerstone of management. However, among patients undergoing pericardiectomy, previous RT is the strongest predictor for adverse outcomes, with reportedly a 5-year survival rate of 12% [33]. These outcomes are likely related to the collateral effects of radiation on other cardiac structures. Moreover, pericardial peeling may be practically challenging, especially if there is underlying myocardial involvement [34]. Therefore in some cases, symptomatic management of HFpEF with diuretics and antihypertensives may be more reasonable.
Recognition and management of radiation valvulopathy
Radiation valvulopathy can be noticed within 10 years following RT. In a 20-year retrospective study, Heidenreich et al. [35] tracked the incidence of valvulopathy in asymptomatic patients with a history of Hodgkin’s lymphoma treated with mantle irradiation. At 10 years, mild or greater regurgitant murmurs were observed, with mitral insufficiency occurring most frequently in 26% of cases. By 20 years, the prevalence of valvular disease had increased, with aortic regurgitation occurring in 60%, mitral regurgitation in 52%, and aortic stenosis in 15% of cases [35]. These findings highlight that the time elapsed since RT contributes to the progression and severity of valvulopathy. Consequently, prolonged latency can falsely lead clinicians to attribute valvular abnormalities to age-related degenerative changes, which may preclude appropriately tailored screening, treatment, and planning for patients with RIHD.
Gross pathology of radiation valvulopathy reveals fibrotic, calcified valves and leaflets, which initially retract and predispose to regurgitation [36]. The pathogenesis is based on microvascular ischemia, fibrosis from radiation insult, and calcification [37]. The presence of valve calcification is likely due to the differentiation of valvular interstitial cells into an osteogenic phenotype [38]. Calcium preferentially deposits around the annulus, subvalvular apparatus, and continuously along the aortomitral curtain (i.e., the confluence of the anterior mitral valve, the non-coronary, and left coronary aortic leaflets), with sparing of the leaflet tips and commissures [39]. Aortomitral thickening and calcification have been independently associated with mortality in patients undergoing cardiac surgery, pointing to their utility as qualitative markers of radiation damage [40]. The aortic and mitral valves are disproportionately affected despite the anterior position of the right heart structures. This suggests that higher pressures and shear stress from the systemic circulation further damage already vulnerable valves [41].
Valve replacement is preferred over repair since irradiated valve tissue will progressively deteriorate. Patients with prior mediastinal radiation who undergo valve replacement have higher mortality and post-procedural complications compared to controls [42–45]. The optimal choice for valve intervention in RIHD is based largely on observational data, focusing on the aortic valve. In a retrospective, multicenter study, Elbadawi et al. [46] reported that transcatheter aortic valve replacement (TAVR) was associated with lower rates of in-hospital mortality, acute kidney injury, bleeding, and respiratory complications, compared to surgical aortic valve replacement (SAVR). Two additional works similarly showed that TAVR was associated with better 30-day expected mortality rates compared to intermediate-to-high-risk surgical patients undergoing SAVR. In low-risk patients, TAVR and SAVR were equal in offering survival advantages [47–48]. Thus, at this time, current data lies in favor of PCI over surgical overall, and even more so in isolated aortic stenosis and in patients without advanced CAD. Surgery is reasonable in low-risk patients and those who have confluent fibrosis affecting both left-sided valves. Elaborating on the latter point, in patients with continuous skeleton calcification, extending along the aortic annulus, aortomitral curtain, and anterior mitral valve, percutaneous methods will likely falter. These patients may have a small aortic root and annular cross-sectional area from radiation damage, making the minimalist approach more challenging. In this scenario, a double valve replacement is more palatable. If surgery is to be undertaken, every attempt should be made for a one-time operation to address all issues, due to the increased risks of re-operation in patients with RIHD. A summary of trials comparing outcomes between TAVR vs. SAVR is provided in Table 1.
TAVR vs. SAVR outcomes in patients with previous mediastinal irradiation
Trial | Key findings |
---|---|
Elbadawi et al. [46] (2020) | TAVR was associated with lower in-hospital mortality (1.2% vs. 2.0%), lower rates of acute kidney injury, bleeding, respiratory complications, and shorter hospital stays relative to SAVR. However, TAVR was associated with higher rates of pacemaker insertion. |
Yazdchi et al. [47] (2021) | SAVR had an operative mortality of 4.3% compared to 1.4% for TAVR. SAVR patients also had longer intensive care unit (ICU) duration and higher blood transfusion requirements. Both cohorts had similar rates of stroke and pacemaker implantation. |
Nauffal et al. [48] (2021) | TAVR was associated with lower rates of postoperative atrial fibrillation, pneumonia, pleural effusion, renal failure, and bleeding compared to SAVR. Stroke/transient ischemic attack and pacemaker implantation were higher with TAVR. Thirty-day mortality, cardiovascular mortality, and hospital readmission in the TAVR group were 4.6%, 1.7%, and 10.9% compared with 3.6%, 1.6%, and 11.2% in the SAVR group, respectively. |
Jørgensen et al. [56] (2021) | At the 8-year follow-up mark, the estimated composite outcome risk of all-cause mortality, stroke, or myocardial infarction (MI) was insignificant between TAVR and SAVR (54.5% vs. 54.8%). |
Kodali et al. [57] (2012) | TAVR and SAVR had similar outcomes pertaining to mortality, symptom reduction, and improved valve hemodynamics. However, paravalvular regurgitation was more frequent after TAVR and was associated with increased mortality later. |
Leon et al. [58] (2016) | TAVR resulted in larger aortic-valve areas than SAVR along with lower rates of acute kidney injury, severe bleeding, and new-onset atrial fibrillation. However, SAVR resulted in fewer major vascular complications and less paravalvular aortic regurgitation. |
Recognition and management of radiation-induced cardiomyopathy
Radiation can lead to fibrosis via direct cardiomyocyte insult or secondary insult from RICAD [18]. As previously described, radiation can accelerate the development of CAD via endothelial cell injury, which decreases the ratio of effective blood vessels to myocytes, leading to cell death. The inflammatory response to necrosis then creates an environment favorable for smooth muscle differentiation into myofibroblasts, which then replaces the interstitium and friable myocardium with the fibrotic substrate. The subsequent stiffening accounts for the diastolic dysfunction and restrictive phenotype that is observed [49]. The right ventricle is preferentially affected over the left due to its more anterior position [2]. Specific data on the management of radiation-induced cardiomyopathy is lacking. Heart failure symptoms can be treated with angiotensin-converting enzyme inhibitors, β-blockers, and diuretics. Treatment for diastolic dysfunction includes exercise training, optimization of risk factors, and potentially SGLT-2 inhibitors as mentioned earlier. Cardiac transplantation remains the penultimate solution, however, postoperative mortality, especially from wound dehiscence, is higher compared to patients with non-irradiated cardiomyopathy [50].
Recognition and management of radiation-induced conduction system disease
The cardiac conduction system can also be a victim of fibrosis and hypoxic tissue damage, though is a less reported complication, only occurring in 4–5% of cases with a latency period of months to years [2]. Patients may present with prolonged QT interval, VT, sinus node dysfunction, atrioventricular block, fascicular block, and bundle branch blocks with a predilection towards the right bundle due to its more anterior location and alignment with the radiation field [51]. Management includes the same workup and interventions as standard counterparts, including antiarrhythmic agents as well as a pacemaker or defibrillator when warranted.
Screening recommendations in RIHD
Primary prevention starts with annual exams and aggressive management of cardiovascular risk factors throughout survivorship. The American Society of Echocardiography (ASE) recommends screening with transthoracic echocardiogram (TTE) or coronary computed tomography angiography (CCTA) in patients who received more than 35 Gy approximately 5 years after completing therapy, with reassessment every 5 years thereafter for those without abnormalities [20].
TTE remains the mainstay in evaluating pericardial disease. Findings include pericardial effusion and the sequelae of constrictive physiology, which if present, confer 88% sensitivity and 91% specificity for the diagnosis of CP: respirophasic septal motion abnormalities, transmitral inflow with a ratio of early (E) and late (A) diastolic filling velocities (E/A ratio) > 0.8, annulus reversus with early diastolic velocity at the medial mitral annulus ≥ 9 cm/s, inferior vena cava (IVC) plethora, and exaggerated hepatic expiratory end-diastolic flow reversal [52]. TTE also provides clues for brewing cardiomyopathy through assessment of left ventricular ejection fraction and global longitudinal strain (GLS), which can pick up on subclinical myocardial toxicity. Significant GLS reduction (i.e., > 18%) is pronounced in areas most exposed to radiation, including the anterior, anteroseptal, and anterolateral walls [53]. TTE is also the primary tool for evaluating valvular abnormalities, such as calcification of the aortomitral curtain and subvalvular apparatus. Doppler assessment can additionally quantify stenosis and regurgitation.
Coronary artery assessment starts with the initial review of baseline computed tomography (CT) imaging obtained at the start and in follow-up identifies patients with asymptomatic CAD who may benefit from preventative therapy [54]. CCTA is slowly emerging at the forefront of primary prevention screening and is especially useful in patients with intermediate pretest probability for obstructive CAD. Moreover, CCTA addresses the false negative rates of functional stress testing in patients with luminal patency but highly vulnerable plaque. This vulnerable soft plaque can be inferred if the lesion has low attenuation, spotty microcalcifications, and a positive remodeling index ratio > 1.1. Fractional flow reserve < 0.80 also allows for the detection of flow-limiting disease [55]. Even more appealing is that CCTA confers less radiation than myocardial perfusion imaging.
Future perspectives
Prospective trials are needed to identify lab or imaging biomarkers for radiation heart damage. These novel predictors can serve to risk stratify patients who may develop cardiac events in the distant future. In addition, monitoring the sequelae of radiation toxicity based on individual risk status would enhance early intervention if needed. Efforts should also be undertaken to develop registries of RT-related cardiovascular disease events. These data banks can provide invaluable information for improving the standardization of screening guidelines.
Conclusions
Recent innovations in oncology have increased the number of cancer survivors. This has led to patients presenting with sequelae of collateral thoracic injury, including RIHD. Reassuringly, complication rates have decreased because of improved radiation precision. Nevertheless, physicians should be cognizant of the potential for cardiotoxicity decades after RT. Studies and clinical experience continue to provide guidance on how to treat these complex patients. However, further research is needed to standardize and optimize management.
Abbreviations
CABG: |
coronary artery bypass grafting |
CAD: |
coronary artery disease |
CCTA: |
coronary computed tomography angiography |
CP: |
constrictive pericarditis |
HFpEF: |
heart failure with preserved ejection fraction |
PCI: |
percutaneous coronary intervention |
RICAD: |
radiation-induced coronary artery disease |
RIHD: |
radiation-induced heart disease |
RT: |
radiation therapy |
SAVR: |
surgical aortic valve replacement |
TAVR: |
transcatheter aortic valve replacement |
TTE: |
transthoracic echocardiogram |
Declarations
Author contributions
CM: Investigation, Supervision, Writing—original draft, Writing—review & editing. PS: Investigation, Writing—original draft, Writing—review & editing, Visualization. JB: Writing—original draft. All authors read and approved the submitted version.
Conflicts of interest
The authors declare that they have no conflicts of interest.
Ethical approval
Not applicable.
Consent to participate
Not applicable.
Consent to publication
Not applicable.
Availability of data and materials
Datasets are available on request: The raw data supporting the conclusions of this manuscript will be made available by the authors, without undue reservation, to any qualified researcher.
Funding
Not applicable.
Copyright
© The Author(s) 2023.