Abstract
Translating biomaterials research into clinical products is a multidisciplinary yet rewarding journey. It should primarily aim to improve patient treatment and clinical outcomes by addressing unmet clinical needs. Four examples of commercial development of biomaterial implants illustrate the diversity of paths, starting from academic research work (AlchiMedics, TISSIUM, Cousin Surgery) or corporate initiatives to develop new products (Medtronic-Sofradim Production). They have been selected from the Translational Research session of the 2022 Conference of the European Society for Biomaterials (ESB) in Bordeaux (France). Commitment, agility, and perseverance were among the key common skills to successfully meet challenges, especially the most unexpected ones. All dimensions of translation projects must be integrated from the start, including the regulatory strategy.
Keywords
Biomaterials, medical devices, translation, clinical developmentIntroduction
The clinical development of biomaterials as implants can be extremely rewarding for patients hoping to improve clinical outcomes in the treatment of their disease. But it is also an entrepreneurial endeavor to translate early-stage concepts or ideas into commercial products for clinical use. This topic has been well covered by recent publications in peer-reviewed journals [1, 2]. It is most often the result of a team of multidisciplinary and well-trained engineers and, also, of experts in support functions (e.g., finance, legal, communication, regulatory and clinical affairs). The regulatory strategy must be integrated as early as possible in the development trajectory of new implants. Finding the right financing in a timely manner is another key factor driving a business to success.
The development of new products is never as simple as one might imagine or idealize at the start and takes longer than initially expected. During the European Society for Biomaterials (ESB) 2022 convention, held in Bordeaux, France, a translational research symposium was organized. Its intention was to illustrate the path of the clinical development of biomaterials from four real cases, presented by companies, AlchiMedics, TISSIUM, Cousin Surgery, and Medtronic. In three examples, the journey has roots in academic research, further developed by the companies in partnership or not with academic research teams. In contrast, Medtronic fully developed their new resorbable mesh entirely in-house, involving two teams, one based in the US and the other one in France.
From quantum chemistry to drug eluting stents: a historical narrative of how the electro-grafting of vinylic polymers ended up in patients
AlchiMedics is a French company, based in Paris, spun off in 2007 from the French Atomic Energy Commission (CEA), based on a technology of electro-grafting: brush polymers, a few hundred of nanometers thick, covalently bonded onto metallic surfaces are created in situ via an electrochemical reaction in organic medium (Figure 1). The technology initially was thought to be a nice “Velcro” layer, strongly bonded to the stent surface and ready to host drugs for their release while avoiding cracking and delamination during tortuous path and expansion.
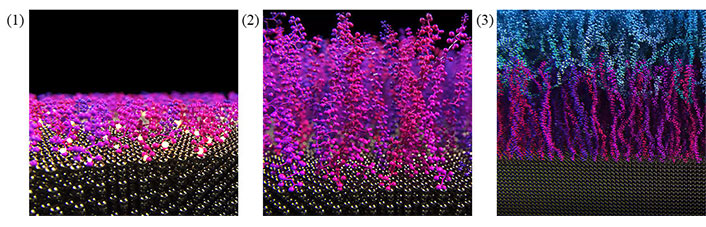
Molecular scale representation of the building of an electro-grafted (eG™) layer on the stent surface. (1) The stent is dipped into a bath of butyl methacrylate monomers in an organic solvent, and serves as a cathode in an electrochemical setup; (2) upon applying a cathodic voltage, the stent surface is turned into a Lewis base and serves as a polymerization initiator to grow a poly-butyl methacrylate brush, covalently bonded to the metal: the eG™ p-the butyl methacrylate molecule (p-BUMA); (3) the eG™ layer serves as an adhesion primer into which a layer of poly-lactide-co-glycolide-acid (PLGA) interpenetrates, like a “molecular Velcro”
The creation of the company was almost synchronous with the outburst of the “late thrombosis” issue described even in the headlines of The Wall Street Journal. The concept of a drug eluting stent, which was releasing a drug inhibiting endothelial cells as much (if not more) than smooth muscle cells, was deeply questioned. It was known at that time that endothelial cells were very sensitive to surface roughness and that their migration was hindered by obstacles. Brush polymer layers prepared by AlchiMedics had roughness lower than 1 nm reproducibly, and they evaluated how endothelial cells were migrating on them. Under the recommendation of Dr Renu Virmani at CVPath Institute, AlchiMedics conducted an animal implantation in rabbits, in which were compared, at 14 days, 28 days and 90 days, a bare stent, a stent coated with a stack made of an eG™ layer and a PLGA layer containing sirolimus, and a Cypher® stent from Cordis (Miami Lakes, Florida), which was considered as the benchmark at that time (Figure 2). This was presented at Transcatheter Cardiovascular Therapeutics conference (TCT) 2007.
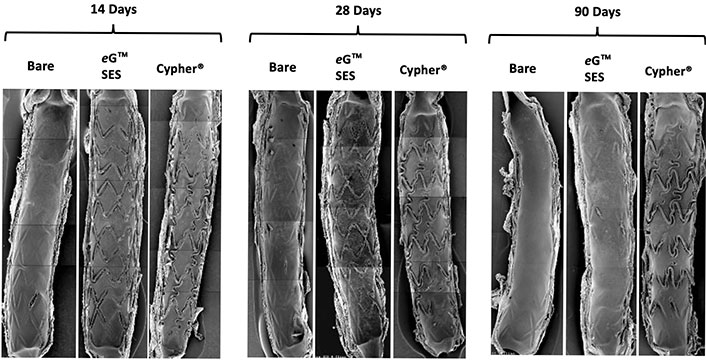
Scanning electron microscope (SEM) pictures at 14 days, 28 days and 90 days of the cross-section of rabbit iliac arteries implanted with a bare metal stent (316L), the same stent coated with a p-BUMA eG™ layer + PLGA layer loaded with 1.2 μg/mm2 of sirolimus [eG™ Sirolimus Eluting Stent (SES)], and the Cypher® stent by Cordis, loaded with the same amount of sirolimus. In all pictures, post-dilatation diamaters are 3.0 mm
Selecting a biodegradable polymer in 2007 was considered a bad choice, as some publications had concluded that biodegradable polymers were inflammatory (all polymers used in commercial stents were biostable). The strategy was to keep “free” the artery from the drug in a definitive manner after some time point, to give a chance to the eG™ layer to play its intended role. The results of these implantations were beyond all hopes with 95% strut coverage already at 14 days for AlchiMedics while the benchmark was 70% at 28 days in the same model and a full covering (100%) at 90 days (Figure 2).
This is how AlchiMedics started to engage in development partnerships with large stent corporations: yet none of these programs went to the end, except with a smaller Chinese company (Beijing Sun Technology), based in Tianjin. AlchiMedics started to collaborate with Beijing Sun Technology Inc. in 2007. First-in-man was completed successfully in 2008, and the product was approved in China in 2010, and launched in 2011 under the name “BuMA®”.
The superior healing performance of the BuMA Supreme® [a cobalt-chromium (CoCr) version of the original BuMA® Drug Eluting Stent (DES), bearing the same eG™ + PLGA/sirolimus stack] was later confirmed in 2018 in humans via Optical Coherence Tomography (OCT) at 1 month and 2 months, in comparison to the Xience™ DES from Abbott (Plymouth, Minnesota) [3]. This materialized clinically, in securing among the lowest level of major advert cardiac events (MACE) at 2 years. Several clinical trials have been conducted, comparing the HT Supreme® (the new name of the BuMA Supreme®) with the leading stents of the market, and demonstrating that the promise of AlchiMedics’ technology to boost endothelial coverage had been held.
Beijing Sun Technology Inc. later became SINOMED and acquired AlchiMedics in 2012. SINOMED is now a unicorn listed on the STAR stock exchange in Shanghai. AlchiMedics’ technology, first used in 2007 in humans, is now implanted in more than 1.5 million patients worldwide, via the BuMA® and HT Supreme® coronary, and NOVA DES™ intracranial drug eluting stent platforms. The HT Supreme® DES is approved in China, has received European Conformity (CE) marking in 2019, and European Medical Device Regulation (MDR) in 2023, and was awarded Investigational Device Exemption by the US Food and Drug Administration (FDA IDE) status in 2019 to start a clinical trial in the US in order to obtain Pre Market Approval (FDA PMA).
The technology was invented, and the company was founded by Dr. Christophe Bureau, former lab director at CEA. Dr Christophe Bureau received several drug eluting stents following a cardiovascular event at the end of 2021. The cardiologist implanted him the HT Supreme® drug eluting stent, successfully closing the loop of a 15-year journey. More than 18 months later, he is now in great health with extremely good cardiac constants.
Main challenges encountered
When AlchiMedics was created, the perceived value of the technology was essentially on its ability to promote a covalent bonding of the eG™ layer onto metals, and thus create a solid brush for mechanical attachment of the PLGA layer securing the absence of cracking and delamination of the polymer coating over expansion. This value proposition, although true, was rather slim: there were other coating technologies being developed that could achieve good enough results to make regulatory-approvable products. The sudden challenge of late thrombosis and its interpretation at the cellular level, highlighting the competition between endothelial cells and smooth muscle cells, was key: the booster performance of eG™ layers towards endothelialization was not anticipated and came as a deus ex machina in the success of the technology.
This might explain the reason why it was hard to obtain buy-in from major players in the field, as they were essentially examining the eG™ technology as a competitor to other more established approaches, like Chemical Vapor Deposition (CVD) or sol-gel methods. The challenge of pinpointing the value proposition linked to the technology was addressed very late, and AlchiMedics had to pivot and seek extra funding to demonstrate the value proposition with express animal trials, under the pressure of tectonic movements in the market.
Relative to this bottleneck moment, scale-up and regulatory were long and tedious, but not so difficult as such. The AlchiMedics Team knew there would be a way, and was lucky enough to have a very solid partner in the market access.
Lessons learnt
AlchiMedics has retained 3 main lessons from this experience:
Whatever the technology, the only thing that matters is the value proposition, and the correlated business model = who buys and why (“why not” being a non-acceptable answer). What does your technology bring that is totally unique? This is a general acid test for any startup company. For healthcare companies, this also translates into: what benefit do you bring to the patient if what you claim is true?
Do not reduce the problems you think you solve to your single domain of expertise: as material scientists, for too long the leadership Team was satisfied with solving a material science “problem”. Facing the issue of late thrombosis was kind of a slap in the face because it was at first sight making AlchiMedics technology totally invisible in the grand scheme of things. AlchiMedics’ CEO worships the talent and self-control of his teams, who were smart enough to spot that they were actually pushed to the front of the stage. Takeway is always re-question whether you know enough, always seek to respond to the “why”, at least as much as the “how”, and—if needed—upgrade your level of expertise to where the action is (cellular biology in our case).
Be resilient: once you understand your value proposition, and why doing what you do matters for patients, nothing will stop you. Good days will be days with one more good news than bad news, and you’ll be happy with it!
Shinning a light on tissue repair: from the bench to the bedside
Tissue adhesives have the potential to promote atraumatic repair of tissues. However, existing tissue adhesives are associated with a strong inflammatory response, weak adhesion, and/or poor control over the bonding process [4]. To meet this need, a viscous, light-activated adhesive that can be exposed to blood flow without compromising its adhesive strength, was developed [5]. The core technology, poly (glycerol sebacate) acrylate (PGSA), is based on the combination of naturally occurring compounds (glycerol and sebacic acid) to form a viscous pre-polymer that can be precisely applied to internal tissues during surgical procedures, both open and minimally invasive. Once applied to the target location, it is polymerized using an external blue light. The resulting bond is elastic, allowing the polymer to comply with the underlying tissue while remaining strongly adhered. Furthermore, this biocompatible polymer is biodegradable.
This versatile polymer platform can be used in several ways. In addition to being applied as a sealant or adhesive and polymerized on-demand, the pre-polymer can be used as a 3D printing resin to build high resolution scaffolds to support tissue repair. It can also be loaded with drugs and deployed potentially anywhere in the body creating a drug depot for local drug delivery.
Leveraging the PGSA polymer platform, originally developed at the Massachusetts Institute of Technology (MIT, Boston, USA), TISSIUM (previously named Gecko Biomedical) was created in France to develop integrated solutions to address diverse clinical needs. Its unique ability to be leveraged as diverse solutions is due to the modular platform design. For each specific indication, a set of dedicated surgical instrumentation is designed to maximize the usability and performance of the polymer. Furthermore, dedicated light sources, for open or minimally invasive procedures, are developed to promote controlled polymerization in each surgical scenario. The PGSA polymer platform offers promising solutions for atraumatic tissue repair.
The first product, a vascular sealant, has been evaluated in different large animal studies of open vascular carotid and aortic surgery. The pre-clinical results were then translated into a clinical setting in a prospective, single-arm multicenter study in patients requiring carotid endarterectomy using an expanded polytetrafluoroethylene (ePTFE) patch [6]. This product received a CE mark in 2017. The range of developed applications for the core polymer technology includes two additional concepts that have shown promise in preclinical studies. TISSIUM has designed a sutureless nerve coaptation system that demonstrated to be a promising alternative to micro-sutures and fibrin glue for strong and effective sensory and motor nerve repair in a rodent animal model. A solution for atraumatic hernia mesh fixation is also currently in development and its performance and safety have been assessed in a porcine model of laparoscopic intraperitoneal onlay mesh placement.
The path to translate a novel biomaterial from the bench to the bedside is not without challenges. To do so requires a strong multidisciplinary team that covers a wide spectrum of capabilities, such as research and development (R&D), manufacturing, clinical and regulatory. It also requires high investment. For example, due to the specificities of its manufacturing process and to scale up effectively, TISSIUM invested in its own manufacturing facilities, something unusual for MedTech startups. In addition, requires adaptability to a changing regulatory environment. This has been particularly true in Europe, with the new Medical Device Regulations (MDR).
CycloMesh™ as a breakthrough scientific innovation for improved surgery—from academic research to clinical validation
In the early 2000s, academic research led to the invention of an innovative and versatile cyclodextrin polymer (used in the design of CycloMesh™). In 2008, a collaboration was born between the academic research teams of University of Lille and the R&D teams of Cousin Surgery, who believed in the potential of this polymer for textile implants through the functionalization of the meshes. The chronic post-operative pain (CPOP) was identified as a frequent surgical complication in the inguinal visceral hernia. On the other hand, no implantable medical device with painkiller loading exists on the market. The management of pain problems, particularly in the field of visceral hernia, was thus retained as an application.
After a convention industrielle de formation par la recherche (CIFRE) or industrial research training convention PhD [7] came the stage of technological and industrial transfer (Figure 3). The visceral hernia mesh with the drug delivery system was born and the teams were working on the manufacturing process.
Designed and developed by Cousin Surgery, CycloMesh™ is an innovative implant for abdominal wall reconstruction with an exclusive technology patented in collaboration with the research teams of the University of Lille [CNRS, UMR8207-Unité Matériaux Et Transformation (UMET) or Materials and Transformations Unit & INSERM U1008-Advanced Drug Delivery Systems]. Based on Cousin Surgery’s expertise, CycloMesh™ is a mesh designed for the treatment of inguinal and femoral hernias. It allows mechanical reinforcement of the abdominal wall locally over the hernia hole after a hernia depression.
After surgical treatment of an inguinal hernia, 8% to 6% of patients develop chronic pain [8]. CycloMesh™ product has a drug delivery system (Figure 4) designed to be loaded per-operatively (during the operating time) by surgeons with the analgesic ropivacaine hydrochloride and then to allow sustained local release of this analgesic after implantation [9]. The interest of this drug loading system is to release progressively and locally the ropivacaine loaded directly onto the mesh implant compared to the systemic administration and rapid elimination with po analgesic intake.
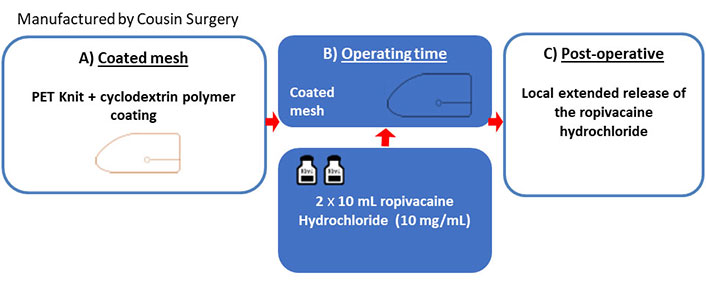
Operative mode of CycloMesh™. Phase A) coated implant; phase B) drug loading in operative room; phase C) local drug release post-operatively
The sterile parietal reinforcement implant with a drug delivery system is therefore a medical device indicated for inguinal & femoral hernia treatment. This device has two functions:
Primary function: mechanical function (parietal reinforcement), non-resorbable.
Secondary function: ropivacaine hydrochloride delivery system (resorbable cyclodextrin polymer coating).
During product development, the main difficulties encountered were related to the interaction between the polymer coating and the active molecule. Cousin Surgery evaluated the ability of the polymer coating to release the active ropivacaine molecule without chemical modification or loss of efficacy.
The new surgical solution as functionalized implant, intended to be used with an analgesic drug, ropivacaine hydrochloride, loaded directly on the textile per-operatively, is included at the border between the medical device and the pharmaceutical field. The challenge is therefore also to bridge together these two worlds, each with its own regulations.
Regarding this challenge, one of the major learnings during the constitution of the technical file is related to the regulatory requirements. Indeed, in this case, the medical device could be not considered as combined with drug [rule 14 of the MDR (EU) 2017/745] because it is intended to be loaded per-operatively; the drug used to be loaded on the medical device could be considered as no integral part of the medical device.
To complete all pre-clinical evidence, a multi-centric double-blind clinical trial has demonstrated patient benefits for this new therapeutical solution. It offers a broader spectrum for surgeons to embrace both abdominal wall reinforcement as well as offering better pain care for patients.
The development of the CycloMesh™ product has taken more than 10 years, from the initial design stage to the completion of the pre-market clinical study. Cousin Surgery is now awaiting the CE mark for 2023, which is required to start marketing in Europe. This collaboration has also delivered some scientific papers and numerous patents. CycloMesh™ has required a lot of investment and collective strength between academic and industrial partners, but there is no doubt that this innovation will open the way to the functionalization of new implantable medical devices.
A novel resorbable polyester, tailor-made to support as a knitted temporary support for primary ventral & incisional hernia repair
Aliphatic polyesters are a family of degradable polymers that have been extensively studied for the use in implantable medical devices both for soft and hard tissue repair. The polymer degradation occurs mostly by bulk hydrolysis, and the in vivo chemical degradation is therefore predictable. In addition, these polymers can be processed in several ways to produce unique designs of medical implants such as sutures, plates, screws, tacks, stents, woven or knitted textiles. The aliphatic polyesters most used for medical implants include polylactic acid (PLA), polyglycolic acid (PGA), polycaprolactone (PCL), poly-trimethylene carbonate (PTMC), and their copolymers.
In the last years, synthetic biodegradable polyesters have found an interest as an alternative to permanent polymers [e.g., polyethylene terephthalate (PET), polypropylene (PP)] and graft materials (e.g., porcine dermis xenografts) for use in hernia repair [10]. The reinforcement with biodegradable synthetic hernia mesh should be sufficiently strong to mechanically reinforce the abdominal wall during the most critical stage of wound healing (i.e., generally, the first 5 months) [11]. In addition, one of the interests of resorbable meshes might be the reduction of possible long-term complications (e.g., chronic inflammation, chronic pain) by material elimination and to promote tissue regeneration, but long-term clinical data are still fully lacking.
In this context, in 2022, more than 1.4 million meshes were used in the clinics worldwide in ventral hernia surgeries. This represents a global market of 895 million USD in 2022 and is expected to be over 1 billion USD by 2026. The resorbable mesh market was at 216 million USD in 2022. In addition, this market segment should grow quite fast with a compound annual growth rate of 5.3% between 2019 and 2030 to end up with projected sales at 294 million USD in 2030 [12].
For this purpose, Medtronic started in 2012 the investigation of resorbable meshes to reinforce tissue where weakness exists, notably during ventral hernia repair procedures. The trickiest part was the selection and development of the most suitable resorbable polymer. The initial choice was poly-L-lactic acid (PLLA). This polymer was chosen because of its degradation profile, and it was already used in Medtronic’s ProGrip™ technology. From the literature, this polymer is described with a satisfying resorption profile from in vitro studies, with a loss of half of the initial mechanical properties in about 6 months [13, 14]. This came into disillusion with animal studies of hernia models. The PLLA mesh prototypes broke earlier than expected within the first 6 months of implementation. The cyclic load of the abdominal wall had been underestimated. Indeed, breathing and physical activities generate deformations of the abdominal wall and therefore on the mesh itself when it is used to give strength to the wall. From this initial pitfall, a large investigation was made to investigate alternative commercial polymers but also to develop a proprietary series of resorbable polymers and yarns designed and tested to meet the specifications: temporary mechanical support for 20 weeks, including cyclic loading constraints.
This investigation ended with a polymer chosen from a dozen. Copolymers of lactic acid and caprolactone and copolymers of lactic acid and trimethylene carbonate have been synthesized and tested with different molar ratios of caprolactone and trimethylene to lactic acid. Random copolymers and copolymers with block design have been considered. The selected polymer was poly-L-lactide, poly-trimethylene carbonate copolymers (PLLA/TMC, 80/20 mol/mol), from mechanical fatigue tests. This polymer was designed as a triblock copolymer with the A-B-A’ structure wherein the A and A’ blocks each include polylactide, the B block includes poly-trimethylene carbonate and polylactide. It was selected from among a series of custom-made copolymers of lactide and trimethylene carbonate and copolymers of lactide and caprolactone. The choice of the polymer was based on its slow degradation rate in combination with good mechanical fatigue resistance profile, under cyclic loading. This mesh will be the first fully resorbable mesh including grips for tissue attachment. The mesh will give equivalent support to permanent synthetic mesh during critical healing period with material that gradually resorbs over time supporting strong repair. This has been shown by both in vitro testing including cyclic mechanical loading and in in vivo animal testing in a porcine hernia model.
The biocompatibility profile of the new mesh has been documented in depth. Particularly, an ISO 10993-6 study was completed to assess the local effects of the PLLA-TMC mesh after implantation, during its various stages of degradation, from its native state to its complete degradation. No systemic nor local adverse reactions have been observed from implantation to complete resorption and metabolization of the mesh in a subcutaneous implantation preclinical rabbit model. The complete in vivo degradation and resorption of the material occurred after 3–5 years (the total resorption period depends on numerous factors including unique patient physiology). The grips are present for easy mesh placement and, possibly, to reduce the number of penetrating fixations. The grips also approximate the mesh to the tissue which is beneficial for good tissue integration. The mesh attachment strength to tissues was also found to be statistically equivalent to the ProGrip™ Self-Gripping Polyester Mesh, the legacy non-resorbable ProGrip™ mesh (base of the knit in polyethylene terephthalate and micro grips in polylactide), in a swine model (Medtronic-Sofradim Production data of a study, performed in compliance to animal ethics guidelines, European animal welfare regulation and Good Laboratory Practice guidelines). Overall, the preclinical testing of the Transorb™ Mesh was compelling enough to make it a new mesh candidate for clinical use.
All key components of the project have been summarized (Figure 5). It started with the microgrip technology, improving the placement of the mesh for ventral hernia repair procedures. A mix of preclinical in vitro and in vivo evidence has been provided to show the safety and efficacy of the new resorbable mesh for the intended indications.
It was a long journey to fulfill all expectations, much longer and more costly than initially planned. Perseverance was a key factor. If the technology and the product you are developing are the most appropriate to meet the identified clinical needs, you should never give up. As long as you can overcome the difficulties, even those not imagined at the beginning of the project, the project will eventually be successful.
Conclusions
The four selected cases give an idea of the diversity of journeys leading to new clinical biomaterial implants.
The first case started from a technology, electro grafting of polymers on conducting surfaces, developed by CEA with very versatile applications, including biocompatibility, protection against corrosion, lubrication, soldering, functionalization, and adhesion [15]. It was applied successfully for the coating of vascular stents with sirolimus for the prevention of (re)-stenosis. But the technology was developed against the grain, in an unfavorable context. It led to unexpected partnerships with fast development and clinical demonstration of the benefit. The technology reached first the Chinese market, before entering the US and European markets much later in 2019. It is a fine example of perseverance, of thinking beyond current trends and of believing in the benefits of technology which have been the keys to the success of AlchiMedics.
The second case demonstrates the development of a new biomaterial driven by an unmet clinical need identified by a surgeon and was translated into a platform solution aimed to enable consistent and atraumatic tissue repair [5]. The initial research work carried out at MIT on the light-activated adhesive was rapidly translated into the first product, a vascular sealant, developed by TISSIUM, which received a CE mark 3 years after the publication of the original research. This adhesive solution has the potential to address different unmet clinical needs, while enabling innovative surgical techniques and atraumatic tissue repair. TISSIUM is currently in the final stages to gain regulatory approval of its solutions for different clinical indications, in the peripheral nerve and hernia fields. Beyond the technological challenge of industrialization and achieving regulatory approval for new adhesive materials, future work and clinical evidence will be key to supporting the adoption of these products by surgeons.
The third case is another illustration of the enhancement of a technological platform, initially developed by an academic laboratory and continued by Cousin Surgery in partnership with this laboratory. The use of cyclodextrin as a carrier for ropivacaine, an analgesic, meets the clinical need for pain relief after abdominal wall hernia surgery. This approach avoids positioning the mesh as a combined device. Overcoming all the challenges of developing a medical device while providing real clinical benefit is not without difficulty, with more than ten years to launch the first human trial. Being innovative, sufficiently disruptive with the market, is always done at the expense of time and needs patience.
The fourth case stemmed from the clinical need to offer resorbable meshes as alternatives to permanent meshes for the repair of abdominal wall hernias. The first approach was to start from PLA, polymers already known for their clinical use, that were believed to respond well to the specifications in terms of resorption profile for hernia repair. It was the confrontation of the new mesh with animal experimentations in an appropriate animal model that revealed the weaknesses of PLA and the need to specifically develop new polymers. Although it was not clearly mentioned in the other cases, the development journey for new products passes through development and check-type iteration loops. They can take longer times, depending on the products and their specifications, which can prove to be much more complex to address than initially planned.
In all cases, the development of new implants must absolutely meet clinical needs, to guarantee their clinical adoption. It should also come, from the very beginning, with a clear value proposition of the new product in the face of competition and by a business model, even a preliminary one, to ensure the economic viability of the new product development project. The generation of technological proofs of concept can come before the identification of clinical development opportunities, but the reconciliation with clinical issues must be done as early as possible. For various reasons, of all kinds and often unanticipated, development rarely takes place in a linear way, but in a more tortuous or surprising way. Through each of the cases, it is the agility and perseverance in the conduct of projects that determine the final success. It is also very important to emphasize the motivation of the team in charge of development and its ability to firmly believe in the technology or the product to reach the end, bringing new clinical solutions to patients.
Abbreviations
CE: |
European Conformity |
CEA: |
Atomic Energy Commission |
eG™: |
electro-grafted |
PGSA: |
poly (glycerol sebacate) acrylate |
PLA: |
polylactic acid |
PLGA: |
poly-lactide-co-glycolide-acid |
PLLA: |
poly-L-lactic acid |
Declarations
Author contributions
YB, CB, MJNP, FA, MC, RV, and DL: Conceptualization, Investigation, Writing—original draft, Writing—review & editing. All authors read and approved the submitted version.
Conflicts of interest
The authors declare that they have no conflicts of interest.
Ethical approval
Not applicable.
Consent to participate
Not applicable.
Consent to publication
Not applicable.
Availability of data and materials
Not applicable.
Funding
Not applicable.
Copyright
© The Author(s) 2024.