Abstract
Transmitted primarily by Aedes aegypti (Ae. aegypti) and Aedes albopictus (Ae. albopictus), arboviral diseases pose a major global public health threat. Dengue, chikungunya, and zika are increasingly prevalent in Southeast Asia. Among other arboviruses, dengue and zika are becoming more common in Central and South America. Given human encroachment into previously uninhabited, often deforested areas, to provide new housing in regions of population expansion, conceptualizing built urban environments in a novel way is urgently needed to safeguard against the growing climate change-driven threat of vector-borne diseases. By understanding the spread from a One Health perspective, enhanced control and prevention can be achieved. This is particularly important considering that climate change is likely to significantly impact the persistence of ponded water where mosquitoes breed due to increasing temperature and shifting rainfall patterns with regard to magnitude, duration, frequency, and season. Models can incorporate aquatic mosquito stages and adult spatial dynamics when habitats are heterogeneously available, thereby including dispersal and susceptible-exposed-infected-recovered (SEIR) epidemiology. Coupled with human population distribution (density, locations), atmospheric conditions (air temperature, precipitation), and hydrological conditions (soil moisture distribution, ponding persistence in topographic depressions), modeling has improved predictive ability for infection rates. However, it has not informed interventional approaches from an urban environment perspective which considers the role of ponds/lakes that support green spaces, the density of population that enables rapid spread of disease, and varying micro-habitats for various mosquito stages under climate change. Here, for an example of dengue in Vietnam, a preventive and predictive approach to design resilient urban environments is proposed, which uses data from rapidly expanding metropolitan communities to learn continually. This protocol deploys computational approaches including simulation and machine learning/artificial intelligence, underpinned by surveillance and medical data for validation and adaptive learning. Its application may best inform urban planning in low-middle income countries in tropical zones where arboviral pathogens are prevalent.
Keywords
Vector-borne disease, arbovirus, dengue, mosquito, urban environment, climate change, digital twin, VietnamIntroduction
Public health threat
The world’s population reached 8 billion people during 2022 and is projected to exceed 9.7 billion by 2050 [1]. This global community increasingly lives in urban environments, with a predicted rise from 55% of inhabitants currently to 80% by 2050 [2]. The rapid growth in urban population density poses significant risks for the spread of vector-borne diseases (VBDs) to human residents of metropolitan areas. VBDs are infectious diseases caused by parasitic, viral or bacterial pathogens that are transmitted to humans by the infectious bite of a blood-feeding arthropod, such as mosquitoes or ticks. Major examples include malaria, dengue, and Lyme disease, caused by a parasite, a virus, and a bacterium, respectively. The first two are global public health threats, the distributions of which are associated with the geographic range of their vector mosquito species [3]. In addition to unchecked expansion of built-up areas encroaching on ecologically sensitive areas, increased exchange between people and mosquitoes is promoted by raised ambient air temperatures and changing seasonal rainfall patterns that favor development of mosquito larvae and disease-transmitting adult mosquitoes [4]. Moreover, in tropical and subtropical zones the public’s awareness of proactive VBD prevention is not high [5], so larvae of mosquito species that transmit notable human pathogens are commonly detected in households and residential areas [6].
This conundrum of unplanned sprawling cities in developing countries raises an important question: is there a way to improve the urban environment to significantly reduce or eliminate the spread of VBDs? In particular, this applies to arthropod-borne (arbo) viruses including dengue, chikungunya, and zika that are transmitted by peridomestic Aedes spp. mosquitoes; i.e., they show a marked preference for living in and around human habitations. Built environments have become sufficiently resilient to provide considerable protection from severe weather events such as rain storms, heat waves, and freezing cold, as well as from flooding, earthquakes, and other natural disasters, and offer security from externalities such as violence and protests. The question that is addressed here is whether it is possible to also envision designing living, working, and recreational spaces for protection against infectious diseases by considering both indoor and outdoor environments as a whole.
A One Health approach
In order to address this question, there is a need to understand VBD spread from a One Health perspective. In other words, the recognition of the interdependence between humans, animals, and ecosystems through their shared (built) environment. As a result, better outcomes may be achieved in controlling or preventing these often debilitating and sometimes life-threatening diseases [7]. This viewpoint is particularly important because climate change is likely to significantly impact these interdependencies by altering the persistence of ponded water in which juvenile mosquitoes develop. This is due to increasing temperature and shifting rainfall patterns with regard to magnitude, duration, frequency, and season [8–10], which can thereby worsen the incidence of arboviral infections. Here, dengue is used as a prime example, but this could also apply to other globally prevalent pathogenic arboviruses.
Dengue virus transmission
The etiological agent of one of the most problematic mosquito-borne diseases of humans, dengue virus (DENV) causes around 100 million symptomatic cases each year worldwide, approximately 70% of which occur in Asia [11]. In 2013, the global economic burden of dengue was estimated at US $8.9 billion [12], a figure which will have grown considerably in the intervening decade. In tropical and subtropical countries, dengue is increasingly prevalent in cities and suburbs [11, 13], compared to rural areas, as the predominant Aedes aegypti (Ae. aegypti) and Aedes albopictus (Ae. albopictus) mosquitoes that transmit the virus have a predilection for urban settings, rather than forests or wetlands [14]. As an example of the public and economic impacts on a dengue-endemic country, in Vietnam, there are an estimated 2 million annual cases of clinical infection. Aside from multiple deaths, the prolonged period of recovery from severe infection, during when a person might not work, leads to between US $30–95 million in lost revenue each year [15, 16].
Building urban resilience to VBDs
Due to their rapid rate of urbanization, fast-growing economies in tropical and subtropical zones would particularly benefit from increasing urban resilience to dengue and other VBDs [17]. There is a pressing need for research on this subject, the outcomes of which would offer immediate opportunities for the implementation of strategies to positively impact affected communities. Discoveries that contribute to reducing disease transmission in the context of the built environment represent exceptional value to people in low-middle income countries and serve as a model worldwide.
Materials
Developing a comprehensive modeling framework to predict the risk of VBDs will pioneer a new aspect of smart health. This applies preventive and predictive approaches for urban planning and learning that continually use data from a given country or region’s rapidly expanding urban development (Southeast Asia, e.g., Vietnam). The proposed protocol deploys computational approaches, including both process-based numerical simulation and machine learning/artificial intelligence, to develop long-term design strategies. Adaptive learning is achieved using environmental surveillance and epidemiological data (typically available from the tertiary care center serving a district, or nationally from a country’s Ministry of Health) for model validation.
Procedure
Modeling urban resilience to dengue
A virtual representation of the physical urban environment, a so-called digital twin, receives sensor data for factors that influence DENV transmission, such as temperature, humidity, and carbon dioxide (CO2) concentration. A model predicts infection risk levels in near real-time and makes associated public health recommendations for risk reduction. This has an immediate and significant impact on future planning of rapidly increasing urbanization in line with fast rates of economic growth. Moreover, the outcomes have broad applicability in tropical and subtropical zones across the world where dengue is prevalent. At present, approaching half of the world’s population lives in these regions, so are potentially at risk of infection [18].
Four interrelated tasks may be investigated in parallel, the findings from each of which may help to refine other related tasks. First, developing a neighborhood scale computational model, with the aim to expand to town/city scale, to predict DENV transmission under different climate scenarios. Second, collecting data for disease incidence and mosquito abundance from selected locations to refine the model. Third, collecting empirical data to understand how different types of interventions are related to disease prevalence and mosquito abundance. Fourth, developing a large-scale model to determine air flow and thermal conditions in selected urban developments and then using machine learning to develop a surrogate model for a digital twin of these housing projects. Online sensors set up for temperature, humidity, and wind speed provide real-world data linked to the digital twin. The combination of modeling, empirical data for disease incidence and mosquito abundance, and real-time sensing allows the digital twin to predict the disease risk level and recommended intervention strategies.
Task 1: evaluating the impacts of climate change on dengue dynamics
The transmission cycle of dengue is notably complex, involving two different replication cycles of DENV alternating in human and Aedes species mosquito hosts (Figure 1). These cycles are often controlled by fluctuations dominated by the random nature of population events (e.g., mosquito dynamics and human mobility) and the space-time variability of suitable environmental conditions. The development of DENV inside adult mosquitoes, which are ectothermic, is air temperature-dependent and expected to be affected by global warming. Current evidence suggests that future climate change, particularly with more frequent extreme weather events, may amplify dengue transmission [19, 20]. This is through increasing mosquito abundance, higher rates of both mosquito biting and survival, and a shorter DENV extrinsic incubation period—the duration between when a mosquito takes a viremic blood meal and when it becomes infectious.
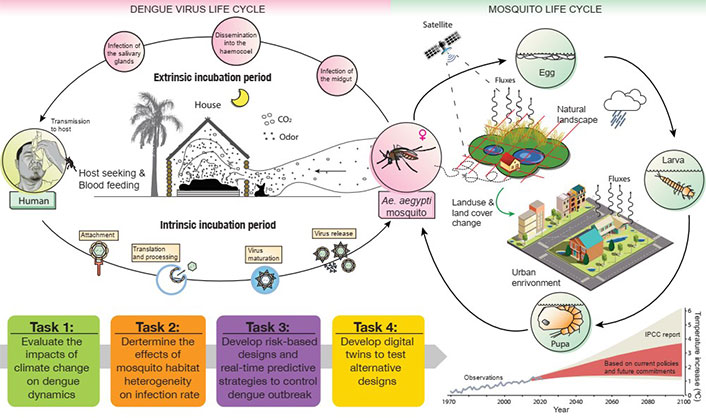
Interlinked life cycles of Aedes mosquitoes and DENV, showing proposed tasks. DENV is transmitted to hosts through saliva by an infectious carrier mosquito when blood-feeding. The arbovirus advances through several stages inside the mosquito, where DENV continues developing with a daytime air temperature dependence until the mosquito becomes infectious to humans. Climate change, as manifested through elevated atmospheric CO2, higher ambient temperature, and altered precipitation magnitude, induces changes in hydrological variability. Changing landscapes, from natural to urban environments, may affect local weather more acutely than long-term climate change. Along with socioeconomic activities, these processes have myriad potential impacts on dengue transmission. IPCC: Intergovernmental Panel on Climate Change
Based on our previous work [21], it is possible to develop a stochastic lattice-based integrated dengue model to predict the dynamics of DENV transmission under climate change for the urban environment. The proposed model consists of two time-continuous space-discrete sub-models that consider the non-linear relationship between temperature and DENV development (including of all four serotypes) inside Aedes vectors to estimate the DENV extrinsic incubation period under global warming. It incorporates explicit coupling between entomological and viral processes, epidemiological statuses, and hydroclimatic conditions to capture the dynamics of dengue. The stochastic entomological model resolves the virus life cycle in aquatic (egg, larva, pupa) and adult stages of Aedes mosquitoes. The coupled epidemiology-virology model simulates stochastically the circulation of DENV in human hosts and adult Aedes vectors using the well-known susceptible-exposed-infected-recovered (SEIR) approach. These two sub-models can be iteratively coupled in discrete space domains through the equality constraint of adult vector populations. In addition, this model also incorporates a meta-population approach to describe spatial movements among discrete geographic domains in urban environments.
The proposed model moves beyond the current state-of-the-art modeling of VBDs. These explicitly incorporate the aquatic stage of the mosquito life cycle and the spatial dynamics of mosquitoes, the distribution of human population, atmospheric conditions, and hydrological conditions into an SEIR epidemiological model [21]. While such models have improved predictive ability for infection rates, they were not developed to gain insights into interventional approaches by coupling the life cycles of vector mosquitoes and arbovirus across the horizontal and vertical heterogeneity of the modern, planned urban environment. In order to design sustainable and healthy metropolitan communities, consideration should be given to the role of parks and detention ponds/lakes that support green spaces, the density of population that enables rapid spread of VBDs, and changing micro-habitats of the mosquito life cycle under climate change. Accordingly, the model may better inform local government policies for the good maintenance of public spaces that should be implemented in order to reduce the risk of mosquito breeding in sites that have been specifically designed for human recreational and leisure activities.
Task 2: determining how heterogeneity of mosquito habitat (horizontally and vertically) in an urban environment affects human infection rate
Ae. aegypti and Ae. albopictus, the main vectors of DENV transmission in urban environments, are anthropophilic mosquito species [22]. The preferred habitat for adult Ae. aegypti, in particular, can be described as a household setting. Typically, gravid adult females lay desiccation-resistant eggs in water-holding artificial containers (e.g., plant pots, buckets, cans, and lids) in and around the home, many of which are discarded and/or small, making source reduction of juvenile habitats in urban environments especially challenging. Mosquito larvae graze on aquatic microbial growth, which may vary considerably in quality and is driven largely by autochthonous inputs of terrestrial leaf litter detritus [22]. In areas where electricity and water supplies are unreliable, households may store water, increasing the likelihood of colonization by Aedes mosquitoes [23]. Due to variations in the reliability of these municipal services, the presence of artificial container habitats, and human population density, in the example of Vietnam the risk of exposure to DENV varies with human land-use gradients [24], socioeconomic gradients [25], and the built environment [26]. However, scant attention has been given to either the vertical built environment or the strategic implementation of stormwater infrastructure to minimize mosquito habitats in tropical urban environments.
The model developed in task 1 may be validated and refined using epidemiological data for disease incidence and field data for mosquito abundance and dengue prevalence. For instance, in Vietnam, the two largest cities Hanoi and Ho Chi Minh City could be selected, together with a third location, Cam Lam, which is under development into a major urban center. Hanoi to the north and especially Ho Chi Minh City to the south are often hotspots of dengue outbreaks [11]. These are conurbations of several million people, with high population densities and increasingly developed residential and business infrastructure, which also serve as major transportation hubs in Vietnam. The south-central coastal city of Cam Lam, on the other hand, is being developed into a smart city of more modest size. These locations have distinct hydroclimatic conditions that may influence mosquito habitats.
By searching for larval habitats in and around households and public green spaces, including water-holding containers and stormwater habitats, mosquito juvenile stages may be visually and/or dip-sampled and their presence/abundance quantified. For surveillance of adult mosquitoes, a combination of traditional ovitrap [27], BG-Sentinel trap [28], and gravid Aedes trap [29] can be deployed, as different trap types may vary in efficacy across environmental contexts. Temperature, humidity, and wind speed require measuring at sampling sites. As mosquito habitats depend on vegetation and the chemical composition of the water [30, 31], the types and coverage of plants in each area require documenting. Water samples need to be characterized chemically for ammonia, phosphate, nitrate, and pH using field sensor kits. Sampling frequency should be at least weekly and increased depending on weather conditions. In order to study the movement of mosquitoes horizontally and vertically in the urban built environment, an iridescent dye is applied to sterilized adult male Ae. aegypti, which are then released as part of a mark-recapture study [32]. Sterile male mosquitoes pose no health risk to humans since they are unable to contribute to mosquito reproduction and do not feed on blood. In initial studies, the number of sterilized mosquitoes released into the environment will be so few as to minimize the potential ecological and evolutionary impacts. Recapturing at different distances from release points enables modeling of the probability of dispersal distances both horizontally and vertically in the built environment. Past datasets, including mosquito abundance and dengue disease incidence (weekly and monthly) at sub-province levels, may also be obtained from a nation’s Ministry of Health to support task 1.
Task 3: developing risk-based design and near real-time prediction-based interventional strategies for infection control and prevention
Existing survey-based studies that use aggregated counts over large spatial units are often inaccurate, either over- or under-estimating the effect of a containment measure. This is because such methods assume the instances of activities to be distributed uniformly across entire cities, provinces, or states [33]. Given the localized effectiveness of a single instance of one containment activity, there is a need for analyses at similarly small (sub-city) spatial resolutions. This requires collecting empirical evidence regarding the efficacy of highly localized containment and intervention activities that target vector mosquito species. Findings may facilitate the optimization of near real-time prediction-based control and prevention strategies in resource-limited, local-level urban settings. If the conceptual framework is successful, consideration should be given to challenges in scaling up effective interventions from a small trial into broader policy and practice. Key factors might include maintaining accuracy and required sources for validating the outcomes, as well as linking data from implementation monitoring to decision making throughout the process [34].
The following interventions can be considered: managing the spatiotemporal distribution of rainwater puddles in present and future climate; distracting the ability of mosquitoes to find hosts by disrupting CO2 gradients that they use to seek them out; and considering stochasticity [e.g., strategies for reducing entrainment of dengue in a domain (neighborhood)]. The non-random deployment of each intervention activity in relation to dengue cases, as well as the deployment of organized containment activities in different epidemiological contexts, such as indoor residual spraying and fogging in the vicinity of a residential building, are accounted for by different modeling frameworks. These also incorporate individual behaviors regarding such activities as keeping plant pots and other containers in which residual water may collect on apartment balconies at different elevations above ground level to which mosquitoes will have variable access. Predictive results from these spatiotemporal analyses will reinforce existing knowledge from observational data on the efficacy of containment activities aimed at adult mosquitoes. By applying the model under varying climate conditions, this informs better building designs and ensures ‘future-proof’ urban planning that discourages and distracts mosquitoes from breeding in a peridomestic environment. Mosquito data should be collected as described in task 2.
Task 4: developing digital twins to test alternative designs for built urban environments
A digital twin may be built by leveraging our newly developed computational fluid dynamics (CFD) model to test alternative urban designs. Since a mosquito cannot be treated as a passive scalar field, there is a two-way coupling between its flying behavior and the surrounding air flow. First, a local scale structure model [35, 36] should be constructed to characterize the aerodynamic behaviors of mosquito migration. The flying characteristics are extracted and fed into a large-scale meteorology-coupled CFD model. Second, the high-fidelity meteorology-coupled CFD simulations characterize the local microclimatic dynamics under different urban configurations. The digital twin is a large eddy simulation (LES)-based model to resolve the atmospheric boundary layer dynamics and meteorology of built environments (Figure 2). These CFD simulations provide detailed spatiotemporal microclimatic fields and reveal the effects of urban configuration on their evolution under both present-day and future climate conditions. Multiple representative urban types in different geographical regions of Vietnam may be used as test sites. The high-fidelity model is built on a finite element discretization that can resolve complex urban geometry and incorporate realistic boundary conditions [37, 38]. These LES simulations are conducted over a domain size of 1 km × 1 km (i.e., neighborhood scale) at a mesh resolution of 1 m, coupled with real-world climate-modeled meteorology. We have recently developed a physics-informed transfer learning framework leveraging satellite imagery, satellite-measured land surface temperature, forcing meteorology data, physics-informed neural networks, and transfer learning techniques to produce urban air temperatures [7, 39]. This set of high-resolution urban air temperature data allows validation of the LES simulations.
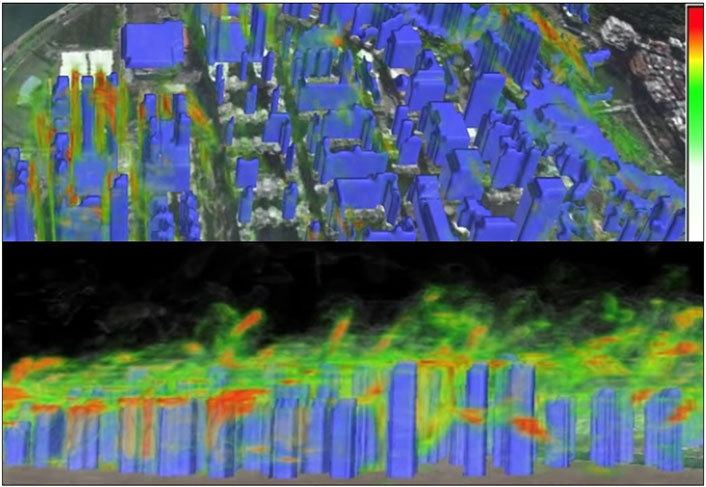
High-fidelity LES over a new-build urban landscape. Streamlines are air flow directions and colors denote wind velocity [blue (slowest) < green < yellow < red (fastest)]
The high-fidelity modeled data is used to predict spatial (both horizontal and vertical) and temporal heterogeneity of mosquito habitats in the urban environment that affect human infection rate. In order to ensure the usability of the digital twin, which needs real-time sensing data and to be computationally efficient, there is a need to develop fast surrogate models using physics-informed machine learning [40, 41] by leveraging labeled data generated by the high-fidelity model.
Sensors for temperature, humidity, and wind speed should be installed in selected residential locations, which are studied in tasks 2 and 3. The digital twin provides simulation of dengue disease risk and intervention strategies in near real-time based on the models developed in task 1, and empirical data collected in tasks 2 and 3. For locations that are planned for future development (e.g., Cam Lam), the digital twin will allow near real-time simulation of alternative neighborhood designs to reduce dengue disease risk.
Expected results
VBDs pose a significant public health threat across the globe. Dengue, chikungunya, and zika, which are all transmitted by Ae. aegypti and Ae. albopictus, are increasingly prevalent in Southeast Asia. West Nile virus occurs frequently in the US, and outbreaks of dengue, zika, and other arboviruses are becoming more common throughout the Americas. This model is expected to provide a breakthrough in conceptualizing built urban environments in a new way to safeguard against the growing climate change-driven threat of all these mosquito-transmitted viral pathogens.
The outcomes of this multidisciplinary research may be disseminated to building contractors to inform and advise their future residential developments through appropriate technology transfer, thereby implementing new smart health protocols for integrated data collection and disease management. The described novel modeling framework will first be tested in Vietnam in the context of dengue control and prevention when planning new-build urban environments. However, in recognizing the humanitarian responsibility to improve global capacity to combat public health threats, it can be easily adapted to various climatic and urban development settings.
Potential limitations of the approach are acknowledged, such as the accuracy of sensor data and the impact of human behavior on disease transmission. Also, some aspects of mosquito behavior or microclimatic dynamics may not be captured accurately in the simulations. These and other potential sources of error in the results will be minimized or accounted for. Subsequent refinements of the initial model will aim to identify limitations and boundaries, how it can be applied, and to which situations. Additionally, it would be interesting to explore how effectively a digital twin can be used for testing designs for non-traditional, alternative urban developments.
Abbreviations
Ae. aegypti: | Aedes aegypti |
Ae. albopictus: | Aedes albopictus |
CFD: | computational fluid dynamics |
CO2: | carbon dioxide |
DENV: | dengue virus |
LES: | large eddy simulation |
VBDs: | vector-borne diseases |
Declarations
Acknowledgments
The authors thank colleagues at the VinUniversity-University of Illinois Smart Health Center, and the Division of Microbiology, Vinmec Healthcare System, for their fruitful discussions.
Author contributions
PK and THN: Conceptualization, Data curation, Methodology, Funding acquisition, Writing—review & editing. PVVL and BFA: Data curation, Methodology, Writing—review & editing. JY and LZ: Methodology, Writing—review & editing. AWTR: Conceptualization, Data curation, Methodology, Funding acquisition, Writing—original draft, Writing—review & editing. All authors read and approved the submitted version.
Conflicts of interest
The authors declare that they have no conflicts of interest.
Ethical approval
Not applicable.
Consent to participate
Not applicable.
Consent to publication
Not applicable.
Availability of data and materials
Not applicable.
Funding
The vector-borne diseases urban environment modeling research discussed in this paper receives financial support from the
Copyright
© The Author(s) 2023.